Inhibition of Osteoclast Bone Resorption by Disrupting Vacuolar H+-ATPase A3-B2 Subunit Interaction
Journal of biological chemistry/The Journal of biological chemistry(2010)
摘要
Vacuolar H+-ATPases (V-ATPases) are highly expressed in ruffled borders of bone-resorbing osteoclasts, where they play a crucial role in skeletal remodeling. To discover protein-protein interactions with the a subunit in mammalian V-ATPases, a GAL4 activation domain fusion library was constructed from an in vitro osteoclast model, receptor activator of NF-κB ligand-differentiated RAW 264.7 cells. This library was screened with a bait construct consisting of a GAL4 binding domain fused to the N-terminal domain of V-ATPase a3 subunit (NTa3), the a subunit isoform that is highly expressed in osteoclasts (a1 and a2 are also expressed, to a lesser degree, whereas a4 is kidney-specific). One of the prey proteins identified was the V-ATPase B2 subunit, which is also highly expressed in osteoclasts (B1 is not expressed). Further characterization, using pulldown and solid-phase binding assays, revealed an interaction between NTa3 and the C-terminal domains of both B1 and B2 subunits. Dual B binding domains of equal affinity were observed in NTa, suggesting a possible model for interaction between these subunits in the V-ATPase complex. Furthermore, the a3-B2 interaction appeared to be moderately favored over a1, a2, and a4 interactions with B2, suggesting a mechanism for the specific subunit assembly of plasma membrane V-ATPase in osteoclasts. Solid-phase binding assays were subsequently used to screen a chemical library for inhibitors of the a3-B2 interaction. A small molecule benzohydrazide derivative was found to inhibit osteoclast resorption with an IC50 of ∼1.2 µm on both synthetic hydroxyapatite surfaces and dentin slices, without significantly affecting RAW 264.7 cell viability or receptor activator of NF-κB ligand-mediated osteoclast differentiation. Further understanding of these interactions and inhibitors may contribute to the design of novel therapeutics for bone loss disorders, such as osteoporosis and rheumatoid arthritis. Vacuolar H+-ATPases (V-ATPases) are highly expressed in ruffled borders of bone-resorbing osteoclasts, where they play a crucial role in skeletal remodeling. To discover protein-protein interactions with the a subunit in mammalian V-ATPases, a GAL4 activation domain fusion library was constructed from an in vitro osteoclast model, receptor activator of NF-κB ligand-differentiated RAW 264.7 cells. This library was screened with a bait construct consisting of a GAL4 binding domain fused to the N-terminal domain of V-ATPase a3 subunit (NTa3), the a subunit isoform that is highly expressed in osteoclasts (a1 and a2 are also expressed, to a lesser degree, whereas a4 is kidney-specific). One of the prey proteins identified was the V-ATPase B2 subunit, which is also highly expressed in osteoclasts (B1 is not expressed). Further characterization, using pulldown and solid-phase binding assays, revealed an interaction between NTa3 and the C-terminal domains of both B1 and B2 subunits. Dual B binding domains of equal affinity were observed in NTa, suggesting a possible model for interaction between these subunits in the V-ATPase complex. Furthermore, the a3-B2 interaction appeared to be moderately favored over a1, a2, and a4 interactions with B2, suggesting a mechanism for the specific subunit assembly of plasma membrane V-ATPase in osteoclasts. Solid-phase binding assays were subsequently used to screen a chemical library for inhibitors of the a3-B2 interaction. A small molecule benzohydrazide derivative was found to inhibit osteoclast resorption with an IC50 of ∼1.2 µm on both synthetic hydroxyapatite surfaces and dentin slices, without significantly affecting RAW 264.7 cell viability or receptor activator of NF-κB ligand-mediated osteoclast differentiation. Further understanding of these interactions and inhibitors may contribute to the design of novel therapeutics for bone loss disorders, such as osteoporosis and rheumatoid arthritis. IntroductionV-ATPases 2The abbreviations used are: V-ATPasevacuolar H+-ATPaseBMMbone marrow mononuclearKM911043,4-dihydroxy-N′-(2-hydroxybenzylidene)benzohydrazideMTS3-(4,5-dimethylthiazol-2-yl)-5-(3-carboxymethoxyphenyl)-2-(4-sulfophenyl)-2H-tetrazolium, inner saltRANKLreceptor activator of NF-κB ligandTRAPtartrate-resistant acid phosphataseTRXthioredoxinNi-NTAnickel-nitrilotriacetic acidα-MEMα-minimal essential mediumM-CSFmacrophage-colony stimulating factor. are proton pumps ubiquitous in eukaryotic cells, where they acidify numerous intracellular membrane compartments, including Golgi, endosomes, lysosomes, clathrin-coated vesicles, chromaffin granules, and insulin secretory granules (reviewed in Refs. 1Marshansky V. Futai M. Curr. Opin. Cell Biol. 2008; 20: 415-426Crossref PubMed Scopus (369) Google Scholar, 2Kane P.M. Microbiol. Mol. Biol. Rev. 2006; 70: 177-191Crossref PubMed Scopus (323) Google Scholar, 3Jefferies K.C. Cipriano D.J. Forgac M. Arch. Biochem. Biophys. 2008; 476: 33-42Crossref PubMed Scopus (199) Google Scholar, 4Cipriano D.J. Wang Y. Bond S. Hinton A. Jefferies K.C. Qi J. Forgac M. Biochim. Biophys. Acta. 2008; 1777: 599-604Crossref PubMed Scopus (117) Google Scholar, 5Saroussi S. Nelson N. J. Exp. Biol. 2009; 212: 1604-1610Crossref PubMed Scopus (44) Google Scholar, 6Gluck S.L. Underhill D.M. Iyori M. Holliday L.S. Kostrominova T.Y. Lee B.S. Annu. Rev. Physiol. 1996; 58: 427-445Crossref PubMed Scopus (124) Google Scholar, 7Stevens T.H. Forgac M. Annu. Rev. Cell Dev. Biol. 1997; 13: 779-808Crossref PubMed Scopus (520) Google Scholar, 8Nelson N. Harvey W.R. Physiol. Rev. 1999; 79: 361-385Crossref PubMed Scopus (367) Google Scholar, 9Forgac M. J. Biol. Chem. 1999; 274: 12951-12954Abstract Full Text Full Text PDF PubMed Scopus (261) Google Scholar, 10Forgac M. J. Exp. Biol. 2000; 203: 71-80Crossref PubMed Google Scholar, 11Nishi T. Forgac M. Nat. Rev. Mol. Cell Biol. 2002; 3: 94-103Crossref PubMed Scopus (985) Google Scholar). V-ATPases also pump protons across the plasma membrane into the extracellular space in a variety of specialized cells, including renal duct intercalated cells, clear cells of the epididymis, and osteoclasts. Here they are involved in functions, including pH homeostasis, sperm maturation, and bone resorption and remodeling. Mutations in V-ATPase subunits lead to diseases, such as renal tubular acidosis and osteopetrosis. Furthermore, their inappropriate activity can contribute to osteoporosis and tumor metastasis (12Forgac M. Nat. Rev. Mol. Cell Biol. 2007; 8: 917-929Crossref PubMed Scopus (1049) Google Scholar, 13Xu J. Cheng T. Feng H.T. Pavlos N.J. Zheng M.H. Histol. Histopathol. 2007; 22: 443-454PubMed Google Scholar, 14Sennoune S.R. Bakunts K. Martínez G.M. Chua-Tuan J.L. Kebir Y. Attaya M.N. Martínez-Zaguilán R. Am. J. Physiol. Cell Physiol. 2004; 286: C1443-C1452Crossref PubMed Scopus (284) Google Scholar, 15Hinton A. Sennoune S.R. Bond S. Fang M. Reuveni M. Sahagian G.G. Jay D. Martinez-Zaguilan R. Forgac M. J. Biol. Chem. 2009; 284: 16400-16408Abstract Full Text Full Text PDF PubMed Scopus (163) Google Scholar).V-ATPases are multisubunit molecular motors, structurally analogous to the F1F0-ATP synthases (F-ATPases), but working “in reverse” (16Yokoyama K. Nakano M. Imamura H. Yoshida M. Tamakoshi M. J. Biol. Chem. 2003; 278: 24255-24258Abstract Full Text Full Text PDF PubMed Scopus (75) Google Scholar, 17Imamura H. Nakano M. Noji H. Muneyuki E. Ohkuma S. Yoshida M. Yokoyama K. Proc. Natl. Acad. Sci. U.S.A. 2003; 100: 2312-2315Crossref PubMed Scopus (163) Google Scholar, 18Zhang Z. Zheng Y. Mazon H. Milgrom E. Kitagawa N. Kish-Trier E. Heck A.J. Kane P.M. Wilkens S. J. Biol. Chem. 2008; 283: 35983-35995Abstract Full Text Full Text PDF PubMed Scopus (94) Google Scholar, 19Drory O. Nelson N. Physiology. 2006; 21: 317-325Crossref PubMed Scopus (47) Google Scholar, 20Cross R.L. Müller V. FEBS Lett. 2004; 576: 1-4Crossref PubMed Scopus (127) Google Scholar). Thus, V-ATPases create proton gradients across membranes by utilizing the energy of ATP hydrolysis, rather than utilizing the potential energy of proton gradients to synthesize ATP.The mammalian V-ATPase complex includes at least 14 different subunits, some of which have multiple isoforms (13Xu J. Cheng T. Feng H.T. Pavlos N.J. Zheng M.H. Histol. Histopathol. 2007; 22: 443-454PubMed Google Scholar). The complex can be divided into two sectors, the cytoplasmic V1 sector and the integral membrane V0 sector. The V1 sector consists of subunits A–H, with a likely stoichiometry of A3B3CDE3FG3H (18Zhang Z. Zheng Y. Mazon H. Milgrom E. Kitagawa N. Kish-Trier E. Heck A.J. Kane P.M. Wilkens S. J. Biol. Chem. 2008; 283: 35983-35995Abstract Full Text Full Text PDF PubMed Scopus (94) Google Scholar). The mammalian V0 sector consists of subunits a, c, c″, d, e, and Ac45, and the stoichiometry appears to be ac5c″deAc45, whereas in yeast one of the c subunits is replaced with a homologous c′ subunit. Regulation of V-ATPase function can occur through dissociation of the complex into its separately inactive V1 and V0 sectors, with the reversible loss of the C subunit from the complex (21Kane P.M. J. Biol. Chem. 1995; 270: 17025-17032Abstract Full Text Full Text PDF PubMed Google Scholar, 22Huss M. Wieczorek H. FEBS Lett. 2007; 581: 5566-5572Crossref PubMed Scopus (22) Google Scholar).Much of the architecture of the V-ATPases, and their molecular motor function, has been inferred from the structure-function analogy with F-ATPases, which, unlike the V-ATPase complexes, have had their structures largely resolved by x-ray crystallography. Crude confirmation of this analogy has been obtained, thus far, largely from single particle electron microscopic analysis of V-ATPases and chemical cross-linking experiments (5Saroussi S. Nelson N. J. Exp. Biol. 2009; 212: 1604-1610Crossref PubMed Scopus (44) Google Scholar, 18Zhang Z. Zheng Y. Mazon H. Milgrom E. Kitagawa N. Kish-Trier E. Heck A.J. Kane P.M. Wilkens S. J. Biol. Chem. 2008; 283: 35983-35995Abstract Full Text Full Text PDF PubMed Scopus (94) Google Scholar, 23Grüber G. Wieczorek H. Harvey W.R. Müller V. J. Exp. Biol. 2001; 204: 2597-2605Crossref PubMed Google Scholar, 24Muench S.P. Huss M. Song C.F. Phillips C. Wieczorek H. Trinick J. Harrison M.A. J. Mol. Biol. 2009; 386: 989-999Crossref PubMed Scopus (82) Google Scholar). Many of the more precise details of intra-complex interactions of V-ATPase subunits, or their extra complex associations with non-V-ATPase polypeptides, remain to be characterized.Landolt-Marticorena et al. (25Landolt-Marticorena C. Williams K.M. Correa J. Chen W. Manolson M.F. J. Biol. Chem. 2000; 275: 15449-15457Abstract Full Text Full Text PDF PubMed Scopus (91) Google Scholar) have shown previously, using yeast two-hybrid analysis, that the a subunit of the V0 sector interacts with the A and H subunits of the V1 sector, interactions that are thought to contribute to the stator complex that is required for the function of the V-ATPase molecular motor (26Féthière J. Venzke D. Diepholz M. Seybert A. Geerlof A. Gentzel M. Wilm M. Böttcher B. J. Biol. Chem. 2004; 279: 40670-40676Abstract Full Text Full Text PDF PubMed Scopus (48) Google Scholar, 27Diepholz M. Börsch M. Böttcher B. Biochem. Soc. Trans. 2008; 36: 1027-1031Crossref PubMed Scopus (34) Google Scholar). The work presented here approaches the problem of further characterizing V-ATPase structure and function by implementing new yeast two-hybrid studies using a cDNA library derived from the murine RAW 264.7 cell line, which is capable of differentiating into osteoclasts in the presence of the cytokine, RANKL (28Raschke W.C. Baird S. Ralph P. Nakoinz I. Cell. 1978; 15: 261-267Abstract Full Text PDF PubMed Scopus (635) Google Scholar, 29Hsu H. Lacey D.L. Dunstan C.R. Solovyev I. Colombero A. Timms E. Tan H.L. Elliott G. Kelley M.J. Sarosi I. Wang L. Xia X.Z. Elliott R. Chiu L. Black T. Scully S. Capparelli C. Morony S. Shimamoto G. Bass M.B. Boyle W.J. Proc. Natl. Acad. Sci. U.S.A. 1999; 96: 3540-3545Crossref PubMed Scopus (1407) Google Scholar, 30Collin-Osdoby P. Yu X. Zheng H. Osdoby P. Methods Mol. Med. 2003; 80: 153-166PubMed Google Scholar). This system is of particular interest because of its high level expression of plasma membrane V-ATPase, which is responsible for proton secretion into the extracellular resorption lacunae of osteoclasts through their specialized ruffled border. This function is required to dissolve bone (31Blair H.C. Teitelbaum S.L. Ghiselli R. Gluck S. Science. 1989; 245: 855-857Crossref PubMed Scopus (727) Google Scholar, 32Väänänen H.K. Karhukorpi E.K. Sundquist K. Wallmark B. Roininen I. Hentunen T. Tuukkanen J. Lakkakorpi P. J. Cell Biol. 1990; 111: 1305-1311Crossref PubMed Scopus (323) Google Scholar), and its disruption in mammals results in the sclerosing bone disorder, osteopetrosis (33Del Fattore A. Cappariello A. Teti A. Bone. 2008; 42: 19-29Crossref PubMed Scopus (207) Google Scholar, 34Kane P.M. J. Bioenerg. Biomembr. 2007; 39: 415-421Crossref PubMed Scopus (80) Google Scholar), although excessive activity results in pathological bone loss, as in osteoporosis (13Xu J. Cheng T. Feng H.T. Pavlos N.J. Zheng M.H. Histol. Histopathol. 2007; 22: 443-454PubMed Google Scholar). Further understanding of the structure and function of V-ATPases will aid the development of targeted therapeutics for the treatment of bone loss diseases, such as osteoporosis and rheumatoid arthritis (13Xu J. Cheng T. Feng H.T. Pavlos N.J. Zheng M.H. Histol. Histopathol. 2007; 22: 443-454PubMed Google Scholar).RESULTSThe initial intention of this work was to determine the set of protein binding partners that interact specifically with the a3 subunit that is highly expressed as part of the plasma membrane V-ATPase of osteoclasts. Crucial interactions with it could potentially be exploited as molecular targets for the treatment of osteolytic disease (12Forgac M. Nat. Rev. Mol. Cell Biol. 2007; 8: 917-929Crossref PubMed Scopus (1049) Google Scholar, 13Xu J. Cheng T. Feng H.T. Pavlos N.J. Zheng M.H. Histol. Histopathol. 2007; 22: 443-454PubMed Google Scholar). Therefore, a mouse cDNA library was constructed from RANKL-differentiated RAW 264.7 cells (29Hsu H. Lacey D.L. Dunstan C.R. Solovyev I. Colombero A. Timms E. Tan H.L. Elliott G. Kelley M.J. Sarosi I. Wang L. Xia X.Z. Elliott R. Chiu L. Black T. Scully S. Capparelli C. Morony S. Shimamoto G. Bass M.B. Boyle W.J. Proc. Natl. Acad. Sci. U.S.A. 1999; 96: 3540-3545Crossref PubMed Scopus (1407) Google Scholar, 30Collin-Osdoby P. Yu X. Zheng H. Osdoby P. Methods Mol. Med. 2003; 80: 153-166PubMed Google Scholar) representing a range of osteoclasts from single TRAP-positive cells to cells containing over 20 nuclei (Fig. 1A) in the HybriZap 2.1 phagemid vector system (37Fields S. Song O. Nature. 1989; 340: 245-246Crossref PubMed Scopus (4822) Google Scholar). The cDNA library was probed in YRG-2 yeast, using a construct of NTa3 (the N-terminal domain of a3, amino acids 1–393; see Table 1) in the yeast two-hybrid GAL4 binding domain vector pBD GAL4 Cam. Although many hits were obtained, the outcome of this screening was difficult to interpret because of unresolved issues with a high background of self-activation with all a subunit bait constructs attempted (data not shown except for NTa3); however, a strong interaction that was consistently above background was found with the full-length B2 subunit of V-ATPase (Fig. 1B). This interaction was confirmed using the second reporter gene, lacZ, and by affinity pulldowns of NTa3 with B2-coated glutathione-Sepharose 4B beads (Fig. 1C).It was of interest to determine whether there were differences among the four murine a subunit isoforms (a1-4) in terms of their interactions with the two murine B isoforms (B1 and B2). TRX fusion proteins (with His tag), NTa1, NTa2, NTa3, and NTa4, were expressed in E. coli and purified using standard Ni(II) affinity chromatography. GST fusion proteins, B1 and B2, were similarly prepared but using glutathione affinity chromatography (Fig. 2A; see also “Experimental Procedures” and Table 1). Affinity pulldowns, as shown in Fig. 2B, were performed, showing that a1, a2, a3, and a4 were efficiently pulled down with both B1 and B2, with somewhat less a2 and strikingly more a3 being pulled down; however, differences between the comparative abilities of B1 and B2 to pull down any of the NTa isoforms were not discernible in replicate experiments.FIGURE 2Expression of NTa and B subunits and pulldown assays of subunit pairs. A, affinity-purified fusion proteins (2 µg, each) separated on 8% SDS-PAGE and stained with Coomassie Blue R-250. Left panel shows proteins expressed from pET32a(+) vector; M, molecular weight standards; followed by (2 µg each) a1, TRX-NTa1 fusion protein; a2, TRX-NTa2; a3, TRX-NTa3; a4, TRX-NTa4. Right panel shows proteins expressed from pGEX-4T-1 vector; B1, GST-B1(GH) (left lane, affinity-purified on GST beads; right lane, repurified on Ni(II) beads); B2, GST-B2(GH) (left lane, affinity-purified on GST beads; right lane, repurified on Ni(II) beads). Photographs are of dried gels. B, pulldowns of TRX fusions of all N-terminal a subunit domains with GST fusions of either B1 or B2 subunits. Glutathione beads were coated with bacterial lysates from cells expressing either GST-B1 or GST-B2 fusion proteins (1 µg/ml total protein), washed, and then exposed to lysates containing TRX-NTa fusions derived from a1, a2, a3, and a4 subunits (1 µg/ml total protein). Washed beads were eluted and run on 8% SDS-PAGE, blotted, and probed with anti-GST antibody. Image was developed using chemiluminescence.View Large Image Figure ViewerDownload Hi-res image Download (PPT)To make more quantifiable determinations of relative binding, an ELISA-based solid-phase binding assay was designed to compare saturation curves for the binding of NTa3, the N-terminal domain of the osteoclast specific isoform, with B1 and B2. Saturation curves (with log abscissae), shown in Fig. 3A, again indicated that differences between NTa3-B1 binding and NTa3-B2 binding were not significant at p ≤ 0.05. Half-maximal binding values were 2.7 ± 0.5 nm for NTa3-B1 and 1.9 ± 0.4 nm for NTa3-B2.FIGURE 3ELISA-based binding assays of NTa3 interactions with B1 and B2 subunits. A shows ELISA saturation curves of B1 (heavy line and error bars) and B2 (light line and error bars) binding to NTa3. ELISA plates were coated with TRX-NTa3 ligand and probed with 2-fold serial dilutions of analyte (GST-B1 or GST-B2 fusion proteins, 9.8 pm to 160 nm). Binding of GST alone was negligible, as were the signals obtained without ligand or either analyte protein (data not shown). Absorbance at 450 nm was determined after staining with an anti-GST-HRP sandwich and color development with 3,3′,5,5′-tetramethylbenzidine (see “Experimental Procedures”). Absorbance at the reference wavelength of 600 nm was subtracted to normalize optical variance among wells (A600 was typically <3% of the maximum A450 signal). Each curve shows means ± S.D. bars (n = 3, in duplicate). Differences between B1 and B2 interacting with NTa3 were not significant. B shows ELISA saturation curves of NTa3ΔT7 (heavy line and error bars), NTa3T7 (light line and error bars), and NTa3(dashed line) binding to CTB2. ELISA plates were coated with GST-CTB2 ligand and probed with 2-fold serial dilutions of analyte (TRX-NTa3ΔT7, TRX-NTa3T7, or TRX-NTa3 fusion proteins, 25 pm to 400 nm). Shown also is purification of TRX-NTa3ΔT7 and TRX-NTa3T7 (SDS-PAGE, inset panel). TRX alone showed negligible binding (data not shown). Each curve shows means ± S.D. bars (n = 3, in duplicate).View Large Image Figure ViewerDownload Hi-res image Download (PPT)Because a3 and B2 subunit isoforms both are highly expressed in the plasma membranes of active osteoclasts (38Manolson M.F. Yu H. Chen W. Yao Y. Li K. Lees R.L. Heersche J.N. J. Biol. Chem. 2003; 278: 49271-49278Abstract Full Text Full Text PDF PubMed Scopus (88) Google Scholar, 39Lee B.S. Holliday L.S. Ojikutu B. Krits I. Gluck S.L. Am. J. Physiol. 1996; 270: C382-C388Crossref PubMed Google Scholar), attention was focused on that pair, and experiments were done to further delineate the sites of binding. A splice variant of the a3 subunit, referred to as Tirc7, with an N-terminal truncation of 217 amino acids, is expressed in T cells (40Heinemann T. Bulwin G.C. Randall J. Schnieders B. Sandhoff K. Volk H.D. Milford E. Gullans S.R. Utku N. Genomics. 1999; 57: 398-406Crossref PubMed Scopus (65) Google Scholar). This variant may delineate a natural domain boundary between the deleted, non-Tirc7, N-terminal half of NTa3 (referred to here as ΔT7) and the Tirc7-specific, C-terminal half of NTa3 (referred to here as T7). We prepared NTa3ΔT7 and NTa3T7 fusion constructs (Fig. 3B, inset, and see Table 1) and, as shown in Fig. 3B, used these to probe B2 ligand, in a reverse of the binding experiment shown in Fig. 3A. NTa3 binding to immobilized B2 was observed at a much lower apparent affinity than B2 binding to immobilized NTa3 (950 ± 310 nm, half-maximal; a 500-fold lower apparent affinity). Surprisingly, the fragmented NTa3 not only bound with a significantly higher apparent affinity (p = 0.01) than the intact moiety, but both halves bound equally well. The half-maximal binding concentrations were 121 ± 84 nm for NTa3ΔT7 and 134 ± 81 nm for NTa3T7, which are not significantly different. Possible explanations for these apparent anomalies are addressed under “Discussion.”Holliday et al. (41Holliday L.S. Lu M. Lee B.S. Nelson R.D. Solivan S. Zhang L. Gluck S.L. J. Biol. Chem. 2000; 275: 32331-32337Abstract Full Text Full Text PDF PubMed Scopus (155) Google Scholar) split B2 into an N-terminal domain (amino acids 1–117 in the mouse ortholog) and a C-terminal domain (amino acids 118–511) and showed that actin binding was confined to the N-terminal domain. In this study, similar constructs were prepared (Table 1) to express GST fusions of N- and C-terminal domains of B2 (Fig. 4A), and solid-phase binding assays were performed (Fig. 4B). In contrast to actin binding, a3 binding to the B2 subunit appeared to be confined to the C-terminal domain, CTB2 (Fig. 4B). Binding of CTB2 to NTa3 was half-maximal at 4.8 ± 1.2 nm, which was not significantly different from binding to the full-length B2 subunit (half-maximal at 3.6 ± 0.8 nm), whereas binding of the N-terminal domain (NTB2) to NTa3 was reduced 50-fold, being half-maximal at 240 ± 30 nm. This difference was highly significant (p = 0.0002).FIGURE 4Determining the domains of interaction between the a and B subunits. A, gel on left shows purified proteins used in pulldown assays and ELISA binding analysis. MW, molecular weight standards; followed by (2 µg each) NTa3, TRX-NTa3; B2, full-length B2 fusion protein, GST-B2(GH); NTB2, GST-NTB2 (N-terminal domain); CTB2, GST-CTB2 (C-terminal domain). Blot on right shows pulldowns of TRX-NTa3 with GST-B2(GH), GST-NTB2, and GST-CTB2, as described. Right-most lane shows control with GST alone (identical blot and exposure with intervening lanes removed). Note that NTB2 pull down is not greatly different from GST control. B, ELISA, as in Fig. 3, but comparing binding of B2 (full-length B2 subunit, GST-B2(GH), heavy line) with that of CTB2 (C-terminal domain of B2, GST-CTB2, light line) and NTB2 (the N-terminal domain of B2, GST-NTB2, dashed line). Fusion protein analytes were 2-fold serially diluted from 400 nm to 24 pm. Each curve shows means ± S.D. bars (n = 3, in duplicate). C shows ELISA-based binding assay using plates coated with GST-CTB2. Analytes were TRX-NTa1–4 (a1, dotted line; a2, light line; a3, heavy line; a4, dashed line) 2-fold serially diluted from 15.6 µm to 0.95 nm. Each curve shows means ± S.D. bars (n = 3, in duplicate). D, similar to C, but using plates coated with GST-B1. Analytes were TRX-NTa1–4 (a1, dotted line; a2, light line; a3, heavy line; a4, dashed line) 2-fold serially diluted from 6.0 µm to 0.15 nm. Each curve shows means ± S.D. bars (n = 2, in duplicate). Automated curve fitting failed for the NTB2 curve because it does not approach saturation. A manually estimated interpolation was derived on the assumption that the data converge in a manner similar to the convergence for B2 and CTB2.View Large Image Figure ViewerDownload Hi-res image Download (PPT)It was of interest to compare the binding of a1–4 subunit isoforms to B2. To do this, ELISA plates were coated with CTB2 ligand and probed with a1–4 analytes (Fig. 4C). Interestingly, NTa3 showed a significantly higher (>2-fold) apparent affinity (half-maximal at 390 ± 32 nm) for binding CTB2 than was seen for NTa2-CTB2 (p = 0.006) and NTa4-CTB2 (p = 0.02) binding, which were statistically indistinguishable at (half-maximal values of 830 ± 140 nm and 1.1 ± 0.3 µm, respectively). The NTa1-CTB2 interaction seemed to have the lowest apparent affinity, 6-fold less than that of the NTa3-CTB2 interaction, with a half-maximal value of 2.5 ± 1.3 µm, which could be distinguished from the NTa2-B2 and NTa4-B2 interactions (p = 0.01). The data suggest that a3-B2 interaction may have a privileged affinity, in comparison with the other a-B subunit interactions.This latter observation begs the question whether binding of a1–4 subunit isoforms to B1 follows a similar distribution. Fig. 4D shows that this is not the case. Apparent affinities are as follows: NTa1-B1, 2.3 ± 0.4 µm; NTa2-B1, 460 ± 57 nm; NTa3-B1, 740 ± 240 nm; NTa4-B1, 1.24 ± 0.04 µm. It is of interest that although the apparent affinities of binding are nearly identical for a1 and a4 with B1 and B2, a2 and a3 have switched relative positions, i.e. a3 paired with B2 seems to be favored in the B2-binding series, whereas a2 paired with B1 seems to be favored in the B1-binding series. Statistical tests, however, indicate that, for binding to B1, a2 and a4 are different (p = 0.001) and a1 binding differs from the rest of the subunits (p ≤ 0.05), but a2 and a3 are not significantly different (p = 0.25).One of the proposed functions of the a subunit in the V-ATPase complex is to act as a stator to prevent futile rotation of the A3B3 catalytic headpiece. It is presumed to accomplish this by anchoring to the membrane through its C-terminal domain, as part of the V0 complex, and binding to the V1 complex through its N-terminal domain, by interactions with the catalytic head group and peripheral stalk components (12Forgac M. Nat. Rev. Mol. Cell Biol. 2007; 8: 917-929Crossref PubMed Scopus (1049) Google Scholar, 27Diepholz M. Börsch M. Böttcher B. Biochem. Soc. Trans. 2008; 36: 1027-1031Crossref PubMed Scopus (34) Google Scholar). We speculated that small molecule inhibitors of a3-B2 interaction might disrupt stator organization and/or destabilize the V1V0 complex and provide a means of regulating V-ATPase activity, possibly specifically targeting the plasma membrane V-ATPase that contains the a3 and B2 subunit isoforms. This could have therapeutic potential for alleviating bone loss disease (13Xu J. Cheng T. Feng H.T. Pavlos N.J. Zheng M.H. Histol. Histopathol. 2007; 22: 443-454PubMed Google Scholar) and limiting the metastatic potential of some tumors (15Hinton A. Sennoune S.R. Bond S. Fang M. Reuveni M. Sahagian G.G. Jay D. Martinez-Zaguilan R. Forgac M. J. Biol. Chem. 2009; 284: 16400-16408Abstract Full Text Full Text PDF PubMed Scopus (163) Google Scholar).To screen for small molecule inhibitors, we adapted the above described ELISA-based solid-phase binding assay for use in a high throughput robotics platform (see under “Experimental Procedures”). Synthetic chemical screening yielded primary hits at a rate of ∼0.2%. Rescreening and evaluation of primary assay dose-response curves reduced this to 0.04% of compounds initially tested. B-scores (35Brideau C. Gunter B. Pikounis B. Liaw A. J. Biomol. Screen. 2003; 8: 634-647Crossref PubMed Scopus (251) Google Scholar), for all 10,241 compounds that were screened, are plotted in Fig. 5A.FIGURE 5High throughput primary screening for inhibitors of a3-B2 interaction, using an ELISA-based binding assay. The Chembridge DIVERSet collection of 10,000 synthetic chemical compounds was screened using the ELISA-based binding assay described and executed on a robotics platform (see “Experimental Procedures”). A, B-score statistics are shown for the compounds, where ELISA absorbances were analyzed in the presence of test compounds. The B-score statistic smoothes systematic machine error and takes into account positive and negative controls. Small black diamonds indicate results for individual compounds. White dashed line indicates mean (B-score = 0), and dotted black lines indicate ± 3 S.D. from the mean. Outliers with a B-score less than ± 3 S.D. were regarded as hits. Hits were retested twice in the primary screen and ranked according to deviation from the mean. The highest ranking hits (with lowest B-scores) were picked for secondary screening. The hit discussed here, KM91104, is indicated with a heavy circle. The only analog of KM91104 in the library (KM91201) is indicated with a light circle. It was also identified as a hit in the primary screen but failed in downstream validation screens (unacceptably high IC50; see also Fig. 9). B shows the chemical structure of compound KM91104, 3,4-dihydroxy-N′-(2-hydroxybenzylidene)benzohydrazide (left panel). This compound was the best hit, overall, in terms of effectiveness in inhibiting osteoclast resorption without effect on osteoclast v
更多查看译文
AI 理解论文
溯源树
样例
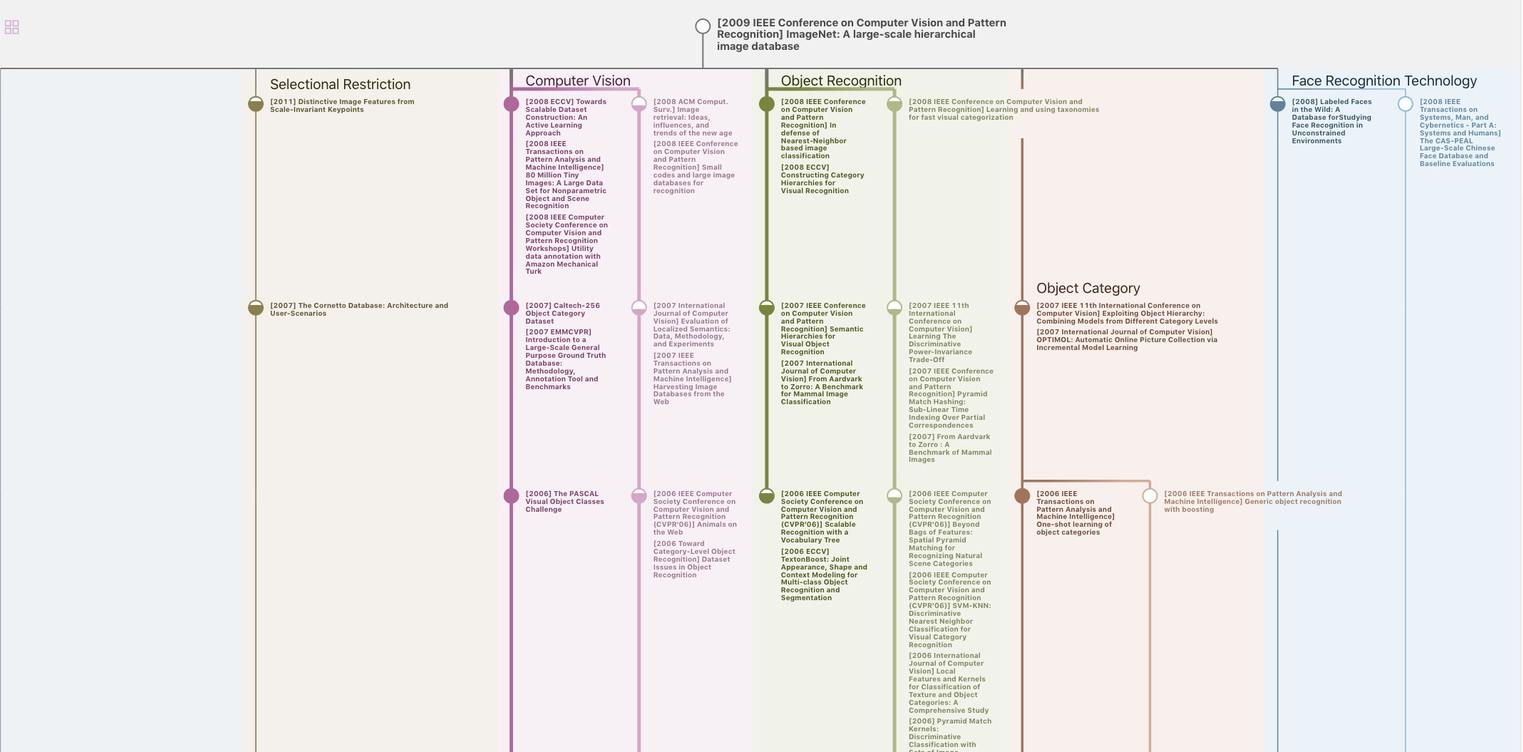
生成溯源树,研究论文发展脉络
Chat Paper
正在生成论文摘要