Theoretical Advances in the Ecology and Evolution of Mutualistic Interactions – Review of a Symposium (SYMP 14) Organized at ESA + CSEE 2022 Joint Meeting
Bulletin of the Ecological Society of America(2023)
摘要
“Therefore, we attempt to treat the same problem with several alternative models each with different simplifications but with a common biological assumption.… Hence our truth is the intersection of independent lies.” (Levins 1966). Mutualisms are bidirectional, beneficial interspecific interactions ubiquitous across taxa (Bronstein 2015). They contribute significantly to ecosystem function and nutrient cycling. For instance, ~40% of global food crops are dependent on a mutualist partner for pollination (Klein et al. 2007). Microbial mutualists, in particular mycorrhizal fungi and nitrogen-fixing bacteria, are responsible for ~75% of annual plant phosphorus uptake and a significant fraction of nitrogen uptake as well (van der Heijden et al. 2008). To better understand these interactions, we require an overarching theoretical framework. This is because empirical results are easily confounded by system-specific differences in interactions, timescale issues, and noise in ecological data. Developing theory for mutualisms is beset by several challenges. First, mutualisms are highly diverse in the currency of benefits they exchange. In plant–microbe mutualisms, the benefits exchanged are usually nutritional resources (carbon and phosphorus, or carbon and nitrogen) (Hacskaylo 1972, Oldroyd et al. 2011). In plant–pollinator mutualisms, however, pollinators obtain benefits in the form of nectar but plants obtain benefits in the form of pollinators facilitating increased movement of pollen (Pellmyr et al. 1996, Pellmyr 2003). The diversity of forms of benefits makes it challenging to develop a theory of mutualism population dynamics that can apply across different interaction types. Further, mutualisms are often temporally dynamic interactions in which the benefits exchanged between species are often mediated by trait evolution. For instance, plants sanction how much carbon they provide to rhizobia in different nodules based on the nitrogen benefits it receives from the bacteria (Denison 2000, West et al. 2002). Similarly, plants evolve to abort fruits which have been parasitized by the larvae of pollinators, preventing exploitation (Janzen 1979, James et al. 1994). In both cases, evolution plays a vital role in stabilizing mutualistic interaction dynamics and species population dynamics. Thus, integrating evolutionary trajectories of species is a second challenge that theory can address to enhance our understanding of mutualisms. Finally, integrating mutualisms into a whole community ecology framework that includes other species interactions such as predation, parasitism, and competition is also an ongoing goal of theory. In order to address these challenges, it is not sufficient to build a framework using empirical studies. Mathematical models serve as a perfect tool to explore different aspects of complex mutualistic species interactions. Much like different empirical systems, different modeling frameworks may be useful for addressing different kinds of questions about mutualism. For instance, network models track the structure and dynamics of pairwise interactions including mutualisms to study how disturbances propagate and functions emerge in species-rich communities (May 1972, Valdovinos et al. 2016, Hale et al. 2020). Trait-explicit evolutionary models such as those based on quantitative genetics or adaptive dynamics study how evolutionary dynamics shape the ecological population dynamics of mutualisms through time (Lande 1982, McPeek 2017, McPeek et al. 2021, 2022). Community ecology models study how interactions between organisms, including mutualism, influence species coexistence, community structure and stability, with a subset of approaches focusing on the emergence of pairwise interactions in small food web “modules” (Holt 1977, Chase and Leibold 2003, Sun et al. 2019, Koffel et al. 2021). Further, incorporating spatial structure into mutualism models using agent-based simulations or partial differential equations (PDEs) allows exploration of how mutualisms vary across space and addresses emergence and maintenance of cooperation between species (Doebeli and Knowlton 1998, Parker 1999, 2001, Stump et al. 2018a,b). Nevertheless, all these kinds of models draw on similar principles, such as the economic principles of consumer-resource interactions and cost–benefit analyses as drivers of behavior in mutualisms. The goal of mutualism theory is not to create one unifying mathematical model for mutualisms, but to draw on common insights from these multifaceted approaches to facilitate their integration and enhance our general conceptual understanding of mutualism. Developing mathematical theory unique to mutualisms helps generate predictions for future experiments as well as aid its integration into the broader context of species interactions. These goals are the main focus of our symposium speakers, who are all early career researchers employing different theoretical approaches to the study of mutualism ecology. Each of the three speakers addressed one of the three challenges highlighted above – diversity in ecological models of mutualism, incorporating eco-evolutionary dynamics, and emergence of mutualisms and its unification with other species interactions – with the aim of introducing the latest developments in mutualism theory to a wide audience of ecologists and evolutionary biologists. This symposium was moderated by Naven Narayanan from the University of Minnesota, with talks given by Kayla Hale (University of Michigan), Thomas Koffel (Michigan State University), and Sarah McPeek (University of Virginia). This article provides a brief summary of the three presentations and the discussion period that followed the talks. In the discussion portion, we highlight some future research directions for mutualism theory, both in a mathematical and empirical context, that emerged from the symposium. Kayla Hale began the symposium by reviewing the history of ecological theory of mutualism, beginning with the famous instability of Lotka–Volterra models that use linear terms for benefit exchanges and the subsequent delay of nearly 40 years before new models were developed. Empirical understanding of the remarkable diversity of natural histories of mutualisms stimulated new interest in mutualism theory beginning in the 1980s as authors focused on finding mechanisms that could reconcile the ubiquity and importance of mutualisms observed in the natural world and the instability of simple models. However, such theory has a long history of being forgotten and rediscovered, perhaps because many models have been criticized as too abstract to be useful or too case-specific to provide insight into the nature of mutualisms more broadly (Bronstein 2015). Hale then advocated an approach for developing a theory that balances detail and generality: building models that permit parameterization and interpretation empirically but are also simple enough to scale up to networks of multiple species interacting without exploding in computational complexity. In this way, conceptual mechanisms can be mapped to qualitative patterns in population dynamics, which can advance understanding of how the varied natural histories of mutualistic partners can impact their populations, communities, and ecosystems. Hale demonstrated this approach for pollination and seed dispersal mutualisms, showing that the requirement for outcrossing in plants causes the unique dynamics of Allee effects and bistable coexistence in pollination mutualisms. However, both mutualisms show stable coexistence at high density and destabilizing thresholds at low density when at least one partner is obligate (Hale et al. 2022). Reviewing historical models of mutualisms showed that this was a general prediction, robust across different inspiring systems and level of detail (Hale and Valdovinos 2021). Additionally, different types of threshold dynamics suggest different underlying mechanisms at play. Empirically measuring whether each partner's population density is increasing or decreasing when one is at high density and the other is at low density can be used to distinguish between potential dynamical drivers, such as the presence of nonlinear costs. This set of qualitative dynamics can be used to characterize the dynamics of mutualisms empirically and guide future study of mutualisms in networks including predation, herbivory, and competition interactions. Thomas Koffel pointed out the need to develop a general theory of species interactions and niche concepts that includes mutualism, extending beyond its current emphasis on competition. The presentation focused on Contemporary Niche Theory (Chase and Leibold 2003) which looks at species coexistence and exclusion along varying resource availability, and its connections with Modern Coexistence Theory (Chesson 2000). In these frameworks, niche difference quantifies the strength of competition, and can be defined by an angular metric that measures the range of conditions that enables coexistence (Saavedra et al. 2017). How can these niche concepts be used to quantify mutualism? The presentation answered this question using metabolic cross-feeding between two bacterial strains, as an example of resource-explicit mutualism (Sun et al. 2019). The main finding was that angular metrics of niche difference still apply to positive interactions, unlike many other metrics (Spaak and De Laender 2020). In fact, high values of niche difference are characteristic of mutualism, while low values correspond to competition. Interestingly, niche differences can also be used to quantify the extent of “niche expansion” by mutualists, a familiar concept from the theory of facilitation (Bruno et al. 2003). In addition, very high levels of mutualism allowed for alternative stable states between persistence and extinction of the mutualistic pair, a pairwise-level example of Allee effect and its associated ‘Allee niche’ (Holt 2009). Koffel further showed the generality of the approach by quantifying the strength of ‘apparent-mutualism’ between plants and carnivores in a short food chain. Sarah McPeek's talk asked the audience to consider ecological theory as a valuable generator of empirically testable predictions. Ecological theory can help researchers address difficult but essential questions in their field by using models to run empirically impractical, if not impossible, experiments. The challenging puzzle at the center of McPeek's work is that of eco-evolutionary feedbacks in mutualisms such as the consumer-resource mutualism between nectar-producing plants and nectar-consuming pollinators (McPeek et al. 2021, 2022). Resource traits such as nectar-production rate and nectar volume evolve via natural selection from mutualistic consumers and other ecological pressures. Over generations, the population dynamics of resource-provider species and resource-consumer species will respond to changing resource supply dynamics, which will in turn affect the dynamics of natural selection on resource traits. Detangling the causes and consequences of these feedbacks helps ecologists understand and predict contemporary and future ecological patterns in mutualisms. McPeek and her coauthors' modeling framework measures the costs and benefits of nectar-production traits as their concrete effects on the growth rate of the plant's population. The model uses the multivariate breeder's equation approach from quantitative genetics to track change in the plant's nectar traits across many generations and corresponding change in the population sizes of the interacting species (Lande 1982, 2007). Plant and pollinator populations achieve an evolutionary equilibrium when the costs and benefits of nectar production balance, effecting stabilizing selection on the plant's nectar traits. Within this general framework, McPeek ran numerical experiments by adjusting parameters that represent three facets of the environment: the foraging activity of pollinators, the death rate of pollinators in the environment, and the intensity of herbivory on plant leaf tissue. Together, these experiments show that increasing nectar supply, which in turn increases both species' population sizes, evolves when ecological conditions limit the frequency of plant–pollinator interactions either via reductions in pollinator foraging effort (pollinator behavior) or reductions in the population sizes of one or both species (e.g., habitat quality for pollinators, herbivory). This highlights an additional benefit of mutualism for the resource provider species: individual plants that produce more nectar for pollinators enhance the growth of their entire plant population through time via their nectar's positive effects on pollinator population sizes. To conclude her talk, McPeek highlighted two approaches empirical researchers could use to test the assumptions and predictions of her theoretical framework. First, researchers should compare the nectar dynamics and interaction dynamics of plants and pollinators found in different ecological settings such as environments with different qualities of habitat for pollinators. Second, researchers should manipulate a facet of the environment such as the number of leaf herbivores in controlled experimental treatments and measure how this ecological difference affects selection on a plant's nectar traits. One approach uses standing ecological variation as a natural experiment to understand the ecological factors that may shape evolved differences in resource provisioning, while the other approach manipulates ecological variables to understand how ecology drives natural selection on resource traits. Combining these two designs in a variety of plant–pollinator mutualisms and other consumer-resource mutualisms will build understanding of the resource-mediated eco-evolutionary feedbacks that shape mutualism ecology in nature. In a panel discussion following the speakers' talks, the audience brought up several questions and open research directions. Broadly, these points fell into one of three categories: costs of mutualism, cheating in mutualism, and integrating mutualisms in diverse communities. Below we outline our perspectives on each of these three key research areas and suggest future directions for empirical and field research: Mathematical models of mutualisms require costs for mutualist populations to equilibrate to a steady state; otherwise, the mutualist population sizes and interaction rates can only increase without limits. Therefore, theoretical perspectives on mutualism require some form of cost to the interaction. For example, McPeek et al.'s quantitative genetic framework assumes that individual plants pay reproductive costs for producing nectar based on several experimental studies demonstrating lower seed production in higher nectar-producing plants (e.g., Pyke 1991). However, we currently have piecemeal evidence that substantial costs of mutualism occur in natural interactions (Bronstein 2001, Morris et al. 2010, Aizen et al. 2014). Are costs a defining feature of mutualisms in nature, or are they more constraints of certain mathematical approaches than biology? We need more research to assess the mechanisms and magnitudes of costs in a variety of mutualisms. Further, we need to understand how substantially costs contribute to the overall dynamics of resource or services exchange in a variety of systems. For instance, available estimates of population level effects of mutualism costs have shown the costs are small and easily swamped out by other demographic factors (Pyke 1991, Kang et al. 2011, Brandenburg et al. 2012, Ford et al. 2015). Therefore, despite the historical emphasis on balancing costs and benefits in the mutualism literature, the simple approach to modeling mutualisms, focusing on their benefits, may be the most justifiable (Hale and Valdovinos 2021). Another common component of theoretical models of mutualism are saturating benefits of mutualism (Hale and Valdovinos 2021). For instance, in the framework developed by McPeek et al., saturating benefits of pollination define the evolution of investment by plants in their mutualistic partners (McPeek et al. 2021). A pollinator can only consume so much nectar, and a plant can only produce so many seeds. Once all those seeds are pollinated, there is no benefit to the plant of continuing to attract pollinators. Many other models of consumer-resource mutualisms have incorporated some form of saturating benefit as a defining feature of the interaction (Holland et al. 2002, Holland and DeAngelis 2009, 2010). However, empirical evidence suggests that benefit functions in mutualism are sometimes saturating at high interaction rates and other times not (e.g., Morris et al. 2010). We need more research that links individual costs and benefits of mutualism with species population dynamics (Chaianunporn and Hovestadt 2012, Mack 2012). Here, theoretical models can help target our investigation because theory sets up certain relationships at the individual level and generates predictions at the population level based on the assumptions. We can examine the validity of model assumptions in systems that match certain predictions and systems that deviate from certain predictions to gain a clearer understanding of the mechanisms governing mutualistic population dynamics in nature. Many mutualism ecologists are interested in when mutualistic interactions break down via cheating, a scenario where one partner gains greater benefits without providing the mutualistic good or service to the other partner (Soberon Mainero and Martinez del Rio 1985, Bronstein 2001). In models of mutualism, the accessibility of cheating strategies depends on the specified mechanisms by which species exchange benefits and, in the case of evolutionary models, the form of selection acting on mutualist traits. In McPeek's theory, for example, pollinators fertilize more plant ovules as they consume more nectar, and traits are subject to stabilizing selection, both of which prevent a sustained ‘cheating’ phenotype. In general, McPeek's's theory suggests cheating should be rare, particularly in environments that hamper population growth of one or both partner species and where resources or benefits exchanged are highly localized. Koffel, on the other hand, pointed out that the public nature of resources in his models makes mutualism particularly susceptible to the tragedy of the commons, where ‘cheaters' can exploit a mutualistic partner without providing any benefit in return. Since these ‘cheaters' do not have to pay the cost associated with reciprocating, they tend to have a higher fitness and thus displace the true mutualists. In this sense, theoretical work on mutualism mirrors empirical work on mutualisms where cheating is common and expected in some systems but rare or even nonexistent in others (Jones et al. 2015). The difference in susceptibility to cheaters between McPeek and Koffel's models potentially arise from different assumptions about the localization of resources. Pollinators must visit flowers to access nectar, while microbes can readily draw nutrients from their environment. Future research must be geared to identify how degrees of resource localization can influence the ease with which cheaters can invade a mutualism. In order to do so, spatial models of mutualism are necessary. However, these models are often very complex and not amenable to simple analysis, limiting their generality (Pande et al. 2016, Stump et al. 2018a,b). A big remaining challenge lies in extending the simple niche theory framework presented here to account for spatial structure while keeping the models tractable, opening the door to better models that provide a clearer understanding of the evolution of mutualism. Hale mentioned that although the focus of the presentation was on pairwise models, studying mutualisms in a network setting is critical. For example, pairwise models show the robust qualitative dynamics whether or not mutualistic rewards are explicitly modeled (Revilla 2015). However, explicit rewards dynamics are important in a network setting for coexistence of mutualists (Valdovinos et al. 2016, Valdovinos and Marsland 2021) and diversity and ecosystem function in food webs (Hale et al. 2020). Similarly, Koffel was asked if his approaches could be applied to quantify the strength and nature of interactions beyond pairs of species. While Saavedra et al. (2017) provided some avenues of generalization on competitive Lotka–Volterra systems, the general challenge associated with describing interactions in diverse communities and understanding how these interactions determine community structures remains. NN initiated and led the symposium and its write-up, after which all authors contributed equally to this manuscript. Each author wrote one of the first four sections with all authors jointly writing the final section. All authors reviewed and edited the manuscript. Authors were listed alphabetically after the first author. No data were collected for this study.
更多查看译文
关键词
mutualistic interactions,ecology,evolution,theoretical advances
AI 理解论文
溯源树
样例
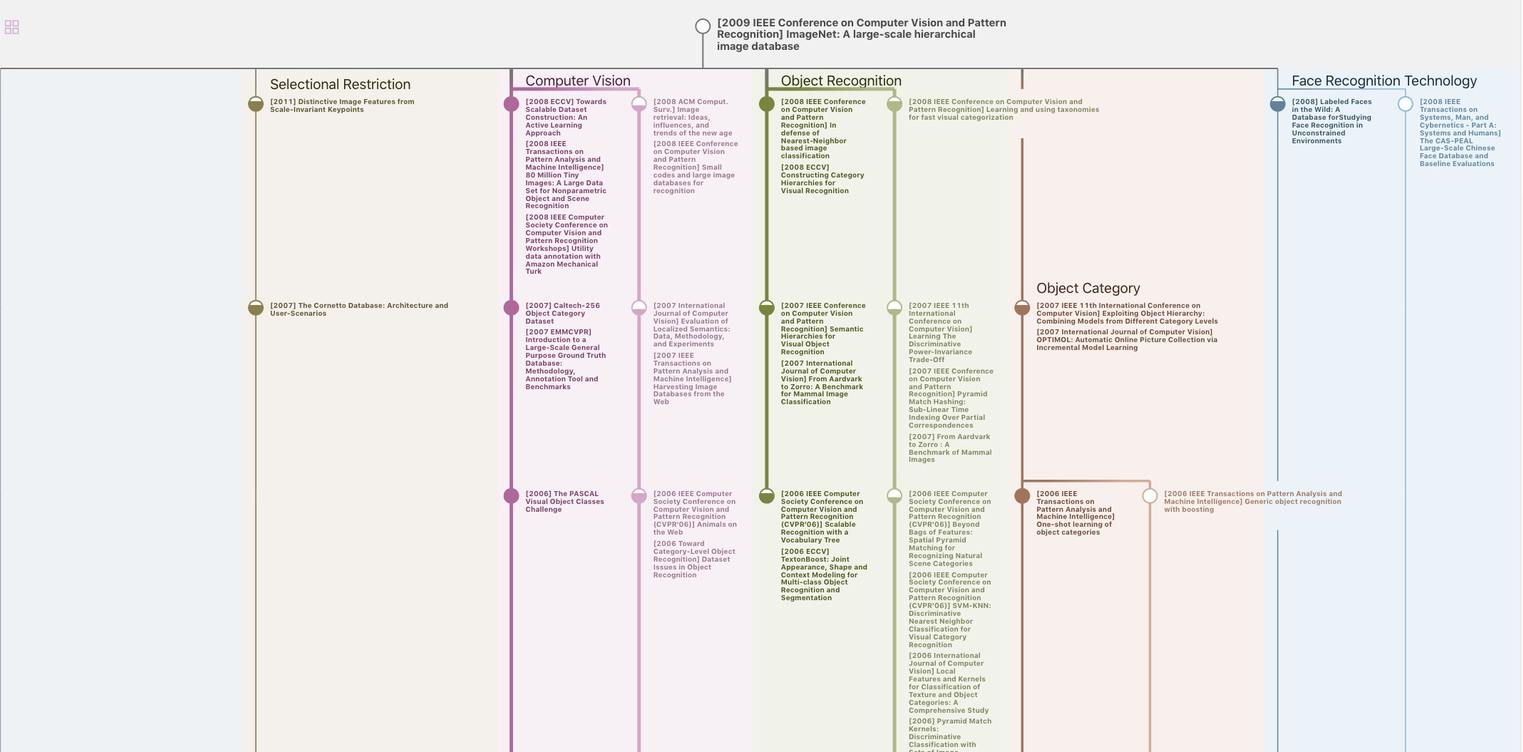
生成溯源树,研究论文发展脉络
Chat Paper
正在生成论文摘要