Development of Large Area Protonic Ceramic Cells for Stack Implementation
Meeting abstracts(2023)
摘要
Protonic Ceramic fuel and electrolysis Cells (PCCs) offer a promising alternative to oxide-conducting cells in the fields of renewable energy and hydrogen. Due to their operation at reduced temperatures and with pure hydrogen flows (while in SOCs the hydrogen flow is diluted with water) they present a potential of cost reduction in large-scale applications. However, the SOC technology is more advanced and is already commercialized at the stack level and implemented in demonstration plants. The newer PCC technology is not yet at the same level as cells and stacks are not commercially available, but research on the scale-up and implementation of cells into stacks and systems is a major topic in the PCC landscape. A major obstacle to the increase of the PCC technology readiness is the scale of the cells themselves. Research on electrochemical activity is mostly reserved for button cells with areas of less than 10 cm 2 . For the cells to be integrated into a stack, the active area needs to be larger to allow for a cost-efficient production of larger-scale devices. However, the manufacture of large cells is complicated by the increase in frequency of defects such as pinholes, cracks, bends among others. These prevent the use of a cell in or decrease the quality of an electrochemical performance measurement. Therefore, a careful and thorough adaption of the manufacturing process is necessary. Compared to common laboratory-scale preparations such as powder pressing, the use of wet chemical industrial processes allows for the elaboration of larger cells and the establishment of protocols that are more easily scalable and may be utilized in commercial applications in the future. Using a multi-step manufacturing wet chemical route, a number of hydrogen electrode-supported cells with areas of over 60 cm 2 and half-cells with a maximum of 75 cm 2 have been achieved. First, the hydrogen electrode is manufactured via tape-casting from a slurry containing NiO and BCZY721 (BaCe 0.7 Zr 0.2 Y 0.1 O 3-d ) as the functional materials. After drying, the cutouts are coated with a BCZY721 electrolyte ink by screen-printing. The two layers are co-sintered at 1300°C to yield a half-cell. The low sintering temperature limits the effect of shrinkage of the half-cell, while a dense electrolyte is nonetheless ensured by utilizing infiltrated ZnO as a sintering aid. The thusly produced half-cells are then coated with air electrode layers composed of LSCF (La 0.4 Sr 0.6 Co 0.2 Fe 0.8 O 3-δ ) or BGLC (Ba 0.5 Gd 0.8 La 0.7 Co 2 O 6-δ ) compositions. To improve the thermomechanical compatibility, a composite layer of BCZY271 and the respective air electrode material is applied before the pure air electrode layer. A second sintering step is performed to ensure a good bonding at the interface between the electrolyte and the air electrode layers. The resulting microstructure is a dense electrolyte of 5-10 µm thickness, whereas the electrodes remain highly porous to ease the gas diffusion. The significant parameters crucial to a high yield of suitable large cells are the electrolyte ink composition and screen-printing process parameters, and the sintering configuration (temperature and pressure applied). The effects of these parameters on the cell output and the electrochemical characterization results of the manufactured complete cells including electrochemical impedance spectroscopy (EIS) will be discussed. As a step towards the implementation into stacks, results on these cells in a serial repeating unit (SRU), i.e. including interconnects and sealing, will also be discussed. Figure 1
更多查看译文
关键词
stack,ceramic
AI 理解论文
溯源树
样例
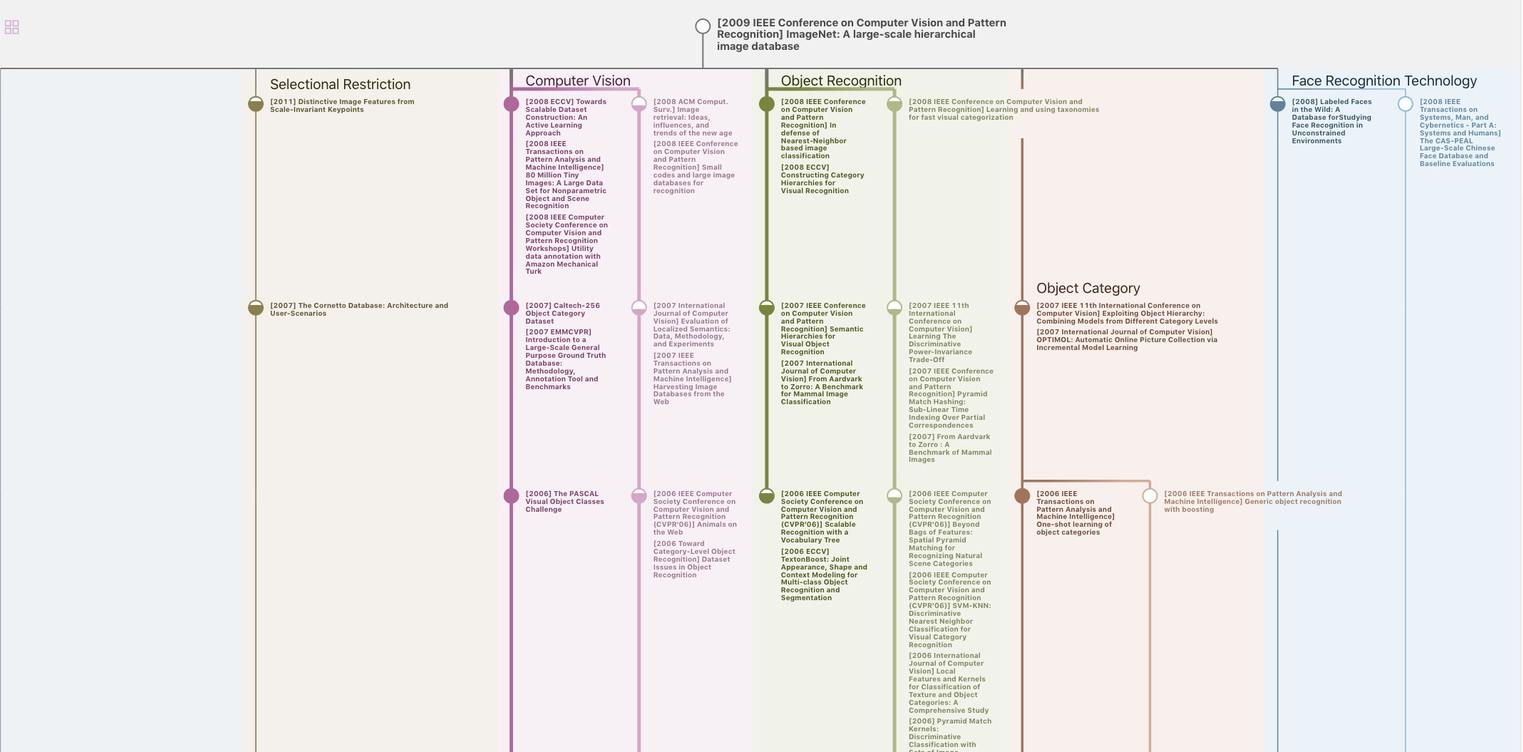
生成溯源树,研究论文发展脉络
Chat Paper
正在生成论文摘要