A role for Mog1 in H2Bub1 and H3K4me3 regulation affecting RNAPII transcription and mRNA export
EMBO Reports(2018)
摘要
Article24 September 2018free access Transparent process A role for Mog1 in H2Bub1 and H3K4me3 regulation affecting RNAPII transcription and mRNA export Paula Oliete-Calvo Gene expression and mRNA Metabolism Laboratory, Centro de Investigación Príncipe Felipe (CIPF), Valencia, Spain Search for more papers by this author Joan Serrano-Quílez orcid.org/0000-0002-6578-8463 Gene expression and mRNA Metabolism Laboratory, Centro de Investigación Príncipe Felipe (CIPF), Valencia, Spain Gene expression and mRNA Metabolism Laboratory, Instituto de Biomedicina de Valencia, Consejo Superior de Investigaciones Científicas (CSIC), Valencia, Spain Search for more papers by this author Carme Nuño-Cabanes Gene expression and mRNA Metabolism Laboratory, Centro de Investigación Príncipe Felipe (CIPF), Valencia, Spain Gene expression and mRNA Metabolism Laboratory, Instituto de Biomedicina de Valencia, Consejo Superior de Investigaciones Científicas (CSIC), Valencia, Spain Search for more papers by this author María E Pérez-Martínez Departamento de Bioquímica y Biología Molecular and E.R.I. Biotecmed, Facultad de Biología, Universitat de València, Burjassot, Spain Search for more papers by this author Luis M Soares Department of Biological Chemistry and Molecular Pharmacology, Harvard Medical School, Boston, MA, USA Search for more papers by this author Bernhard Dichtl School of Life and Environmental Sciences, Faculty of Science, Engineering and Built Environment, Centre for Cellular and Molecular Biology, Deakin University, Geelong, Vic., Australia Search for more papers by this author Stephen Buratowski Department of Biological Chemistry and Molecular Pharmacology, Harvard Medical School, Boston, MA, USA Search for more papers by this author José E Pérez-Ortín Departamento de Bioquímica y Biología Molecular and E.R.I. Biotecmed, Facultad de Biología, Universitat de València, Burjassot, Spain Search for more papers by this author Susana Rodríguez-Navarro Corresponding Author [email protected] orcid.org/0000-0001-7472-3111 Gene expression and mRNA Metabolism Laboratory, Centro de Investigación Príncipe Felipe (CIPF), Valencia, Spain Gene expression and mRNA Metabolism Laboratory, Instituto de Biomedicina de Valencia, Consejo Superior de Investigaciones Científicas (CSIC), Valencia, Spain Search for more papers by this author Paula Oliete-Calvo Gene expression and mRNA Metabolism Laboratory, Centro de Investigación Príncipe Felipe (CIPF), Valencia, Spain Search for more papers by this author Joan Serrano-Quílez orcid.org/0000-0002-6578-8463 Gene expression and mRNA Metabolism Laboratory, Centro de Investigación Príncipe Felipe (CIPF), Valencia, Spain Gene expression and mRNA Metabolism Laboratory, Instituto de Biomedicina de Valencia, Consejo Superior de Investigaciones Científicas (CSIC), Valencia, Spain Search for more papers by this author Carme Nuño-Cabanes Gene expression and mRNA Metabolism Laboratory, Centro de Investigación Príncipe Felipe (CIPF), Valencia, Spain Gene expression and mRNA Metabolism Laboratory, Instituto de Biomedicina de Valencia, Consejo Superior de Investigaciones Científicas (CSIC), Valencia, Spain Search for more papers by this author María E Pérez-Martínez Departamento de Bioquímica y Biología Molecular and E.R.I. Biotecmed, Facultad de Biología, Universitat de València, Burjassot, Spain Search for more papers by this author Luis M Soares Department of Biological Chemistry and Molecular Pharmacology, Harvard Medical School, Boston, MA, USA Search for more papers by this author Bernhard Dichtl School of Life and Environmental Sciences, Faculty of Science, Engineering and Built Environment, Centre for Cellular and Molecular Biology, Deakin University, Geelong, Vic., Australia Search for more papers by this author Stephen Buratowski Department of Biological Chemistry and Molecular Pharmacology, Harvard Medical School, Boston, MA, USA Search for more papers by this author José E Pérez-Ortín Departamento de Bioquímica y Biología Molecular and E.R.I. Biotecmed, Facultad de Biología, Universitat de València, Burjassot, Spain Search for more papers by this author Susana Rodríguez-Navarro Corresponding Author [email protected] orcid.org/0000-0001-7472-3111 Gene expression and mRNA Metabolism Laboratory, Centro de Investigación Príncipe Felipe (CIPF), Valencia, Spain Gene expression and mRNA Metabolism Laboratory, Instituto de Biomedicina de Valencia, Consejo Superior de Investigaciones Científicas (CSIC), Valencia, Spain Search for more papers by this author Author Information Paula Oliete-Calvo1,‡, Joan Serrano-Quílez1,2,‡, Carme Nuño-Cabanes1,2, María E Pérez-Martínez3, Luis M Soares4, Bernhard Dichtl5, Stephen Buratowski4, José E Pérez-Ortín3 and Susana Rodríguez-Navarro *,1,2 1Gene expression and mRNA Metabolism Laboratory, Centro de Investigación Príncipe Felipe (CIPF), Valencia, Spain 2Gene expression and mRNA Metabolism Laboratory, Instituto de Biomedicina de Valencia, Consejo Superior de Investigaciones Científicas (CSIC), Valencia, Spain 3Departamento de Bioquímica y Biología Molecular and E.R.I. Biotecmed, Facultad de Biología, Universitat de València, Burjassot, Spain 4Department of Biological Chemistry and Molecular Pharmacology, Harvard Medical School, Boston, MA, USA 5School of Life and Environmental Sciences, Faculty of Science, Engineering and Built Environment, Centre for Cellular and Molecular Biology, Deakin University, Geelong, Vic., Australia ‡These authors contributed equally to this work *Corresponding author. Tel: +34 963391760; E-mail: [email protected] EMBO Rep (2018)19:e45992https://doi.org/10.15252/embr.201845992 PDFDownload PDF of article text and main figures. Peer ReviewDownload a summary of the editorial decision process including editorial decision letters, reviewer comments and author responses to feedback. ToolsAdd to favoritesDownload CitationsTrack CitationsPermissions ShareFacebookTwitterLinked InMendeleyWechatReddit Figures & Info Abstract Monoubiquitination of histone H2B (to H2Bub1) is required for downstream events including histone H3 methylation, transcription, and mRNA export. The mechanisms and players regulating these events have not yet been completely delineated. Here, we show that the conserved Ran-binding protein Mog1 is required to sustain normal levels of H2Bub1 and H3K4me3 in Saccharomyces cerevisiae. Mog1 is needed for gene body recruitment of Rad6, Bre1, and Rtf1 that are involved in H2B ubiquitination and genetically interacts with these factors. We provide evidence that the absence of MOG1 impacts on cellular processes such as transcription, DNA replication, and mRNA export, which are linked to H2Bub1. Importantly, the mRNA export defect in mog1Δ strains is exacerbated by the absence of factors that decrease H2Bub1 levels. Consistent with a role in sustaining H2Bub and H3K4me3 levels, Mog1 co-precipitates with components that participate in these modifications such as Bre1, Rtf1, and the COMPASS-associated factors Shg1 and Sdc1. These results reveal a novel role for Mog1 in H2B ubiquitination, transcription, and mRNA biogenesis. Synopsis The conserved Mog1 protein is important for H2Bub1, H3K4me3, transcription, and mRNA export. This novel role is distinct from its known ability to bind Ran-GTP during protein transport. Mog1 is required to sustain normal levels of H2Bub1 and H3K4me3 and co-precipitates with components that participate in these modifications in Saccharomyces cerevisiae. The absence of MOG1 impacts on cellular processes linked to H2Bub1, such as transcription and mRNA export. Mog1 binding to Ran is not required for its role in epigenetic modifications. Introduction Eukaryotic gene expression is a complex process that is regulated at multiple levels, including nuclear and cytoplasmic events. Prior to mRNA export from the nucleus, a sophisticated crosstalk between different histone modifications acts to control transcription and its coupling to mRNA processing and export 1-3. A crucial histone modification implicated in this crosstalk is monoubiquitination of histone H2B on lysine 123 in yeast (K123) or on lysine 120 in mammals (K120) to form H2Bub1 4. H2Bub1 is involved in a variety of biological processes ranging from cellular growth to transcription initiation and elongation, and gene silencing 5. Dysregulation of this modification in higher eukaryotes leads to important pathological events such as tumorigenesis and deficiencies in cellular differentiation and development 6, 7. The E2 ubiquitin-conjugating enzyme Rad6 catalyzes H2B monoubiquitination in conjunction with the E3 ubiquitin ligase Bre1 and the transcriptional regulatory protein Lge1 8-11. H2Bub1 is present throughout the entire transcriptional units of active genes due to the association of Rad6 and Bre1 with chromatin through their interaction with activators and with RNA polymerase II (RNAPII) 12, 13. H2B is, therefore, co-transcriptionally ubiquitinated, and its H2Bub1 levels correlate with RNAPII elongation rates 14. The monoubiquitination of H2B also requires the presence of the PAF complex (PAFc). Recent studies have shown that the Rtf1 subunit of the PAFc acts as a cofactor for H2B monoubiquitination by promoting the ability of Bre1 to stimulate Rad6 activity 15. Mutations affecting H2Bub1 levels specifically abolish di- and trimethylation of H3K4 and H3K79 while only marginally affecting monomethylation 16, 17. Rad6/Bre1-dependent H2Bub1 also promotes the ubiquitination of Swd2 resulting in control of the recruitment to chromatin of Spp1, a COMPASS subunit that stimulates the overall rate of methylation of H3K4 18, 19. It is consequently thought that the PAFc affects H3K4 and H3K79 methylation through its effect on H2B ubiquitination 8, 20. Both the addition and the removal of the ubiquitin moiety to and from H2B are required for optimal gene transcription. H2Bub1 is deubiquitinated by two ubiquitin-specific proteases in S. cerevisiae: Ubp8 and Ubp10. Ubp8 is part of the deubiquitination module (DUBm) of the SAGA complex, which is composed of four proteins that are conserved from yeast to humans: yUbp8/hUSP22, ySus1/hENY2, ySgf73/hATXN7, and ySgf11/hATXN7L3 21-25. The presence of these four subunits, together with their correct assembly, is necessary for H2Bub1 deubiquitination 26-31. Several studies have provided important insights into the crystal structure of the DUBm and have shown that the four components are remarkably intertwined 28, 31, 32. Notably, recent work in vitro has shown that the DUBm can contact the nucleosome core particle acidic patch via Sgf11 to which it competes with Rad6-Bre1 for binding. This competition might serve to fine-tune cellular levels of H2Bub1 genome-wide 33. H2Bub1 is also functionally connected with mRNA export. For instance, Sus1, a component of both SAGA DUBm and TREX-2 complexes, plays a key role in coupling transcription activation, H2Bub1 deubiquitination, and mRNA export 34. It has also been shown that deletion of either DUBm subunits Sgf11 or Sgf73 exacerbates mRNA export defects 35, 36, while the TREX-2 subunit Sem1 is required for SAGA-dependent deubiquitinating activity 37. These data highlight the bidirectional functional connection between the two complexes. In addition, H2Bub1 controls the formation of export-competent mRNPs by affecting recruitment of the different Mex67 adaptors to these complexes and by regulating the levels of the COMPASS subunit Swd2 2. All of these findings indicate strong crosstalk between H2Bub1 and mRNP biogenesis. Here, we identified a novel component required for proper H2B monoubiquitination in yeast: Mog1, which is a conserved Ran GTPase-binding protein that is required for nuclear protein import 38-40. We demonstrate that deletion of MOG1 affects the global levels of both H2Bub1 and H3K4me3 and the chromatin recruitment of factors implicated in their modifications. Using Genomic Run-On (GRO), Mog1-TAP purification, and ChIP techniques, we determined that cells lacking MOG1 showed a global impairment of transcription that is consistent with the co-purification of Mog1 with factors involved in transcription and with Mog1 association with chromatin. Notably, defects in mRNA export are exacerbated by the combination of MOG1 deletion and mutations in H2B ubiquitination factors, suggesting that upstream processes that are partly coordinated by Mog1 affect mRNA export. This new function of Mog1, which is independent of Mog1 binding to Ran-GTP, extends its functions beyond its well-known role in protein import. Results Mog1 stimulates the monoubiquitination of H2B Sus1 plays a critical role in coordinating histone H2B monoubiquitination, transcription, and mRNA export 35, 36. We hypothesized that identification of factors that genetically interact with SUS1 might lead to the discovery of new factors involved in this coordination. We found that mog1Δ displayed the strongest genetic interaction with SUS1 deletion in genome-wide screenings using synthetic genetic arrays (SGA) (mog1Δ: SGA score −0.67 41, 42. Mog1 is a nuclear protein that interacts with Ran-GTP. Mog1 stimulates the release of GTP from Ran, thereby conferring directionality to the nuclear import and export pathways 39, 40. In order to validate this genetic interaction, we created a double-deletion mutant and analyzed the effect of MOG1 deletion on the growth of cells lacking SUS1. mog1Δ showed a negative genetic interaction with sus1Δ (Fig 1A). Next, we analyzed Gene Ontology (GO) of all genes that showed a genetic interaction with mog1Δ (Dataset EV1). Notably, the four members of the SAGA deubiquitination module (Sus1, Sgf73, Ubp8, and Sgf11) were the genes whose GO annotation had the highest enrichment (Table EV1), suggesting that the DUBm components are genetically linked to MOG1. To confirm these genetic interactions, double mutants were created in which mog1Δ was combined with mutants of DUBm components. Genetic interactions were evident when MOG1 deletion was combined with deletion of UBP8 or of SGF73, with the strongest effect on growth observed for the double mutant mog1Δubp8Δ (Fig 1A). Figure 1. Monoubiquitination of H2B requires Mog1 A. MOG1 interacts genetically with the H2B deubiquitination machinery. Tenfold serial dilutions of the indicated strains were spotted on YPD and incubated for 2 days at the indicated temperatures. B. Expression levels of total H2B and H2Bub1 were analyzed in wild-type (WT), mog1Δ, ubp8Δ, and ubp8Δmog1Δ whole-cell lysates by Western blotting using anti-total H2B and anti-H2Bub1 antibodies. Bar graphs of H2Bub1 levels after H2B normalization show the mean and standard deviations of at least three independent experiments. Error bars represent SD. The P-value was calculated using Student's t-test (*P = 0.01–0.05; **P = 0.001–0.01; ***P < 0.001). C–E. The indicated strains were diluted as described in (A) and were then spotted on YPD or YPGal and incubated for 2 days. Download figure Download PowerPoint Since genetic interactions might potentially be indicative of functional relationships, we decided to analyze the global levels of histone H2Bub1 in a mog1Δ mutant. The absence of MOG1 significantly reduced the total level of H2Bub1as determined by Western blotting (Fig 1B). To determine whether the low levels of H2Bub1 were a consequence of low ubiquitination or of high Ubp8-dependent deubiquitinating activity, the double mutant mog1Δubp8Δ was also analyzed. Fig 1B shows that the reduction in H2Bub1 levels observed in the absence of MOG1 was not fully rescued by UBP8 deletion, suggesting that the low H2Bub1 level in the mog1Δ strain was not UBP8-dependent. In accordance with this result, MOG1 deletion did not affect the interactions of TAP-tagged Ubp8 with other cellular proteins or its chromatin association (Fig EV1A and B and Table EV2). In summary, these data suggested that Mog1 affected H2B monoubiquitination rather than the opposing H2B deubiquitinating activity. Click here to expand this figure. Figure EV1. Ubp8 phenotypes are not affected by MOG1 deletion A representative Coomassie-stained SDS–PAGE gel showing the pattern of proteins co-purifying with Ubp8-TAP from wild-type (WT) or mog1Δ cells. Protein markers (M) of the indicated molecular weight (kDa) are shown in lane M. The list of proteins detected using mass spectrometric analysis is shown in Table EV2. ChIP analysis of the presence of TAP-tagged Ubp8 at ADH1, PMA1, and YEF3 promoter (P) and coding regions (5'ORF) in WT or mog1Δ strains. The bar graphs indicate the mean and standard deviation for at least three independent experiments. Significance of the differences was calculated using Student's t-test. Download figure Download PowerPoint Mog1 interacts genetically with components involved in H2B monoubiquitination To explore the interaction between Mog1 and the H2B monoubiquitination machinery, genetic assays were conducted in which MOG1 deletion was combined with individual mutants of RAD6 (E2), BRE1 (E3), or its associated protein LGE1. Growth assays indicated that mutation of MOG1 led to a synthetic sickness phenotype when combined with a deletion of any of the three genes (Fig 1C). Since H2Bub1 levels are also regulated by the PAFc 43, we combined MOG1 deletion with deletion of the PAFc subunit RTF1 and found that the resulting double mutant displayed an additive growth phenotype (Fig 1D). These results indicated that Mog1 and Rad6, Bre1, or Rtf1 act in independent pathways. However, since the absence of any of the factors Rad6, Bre1, or Rtf1 alone results in undetectable levels of H2Bub1 15, 33, it is unlikely that the growth phenotypes of the double mutants are a consequence of further reduction in H2Bub1 levels that is caused by mog1Δ. To examine whether a process in addition to H2B monoubiquitination is affected by the loss of Mog1, we analyzed the genetic interaction between mog1Δ and a histone H2B mutant (H2B-K123R) that is specifically defective in H2B monoubiquitination 44. As shown in Fig 1E, deletion of MOG1 in the H2B-K123R mutant resulted in a subtle growth retardation suggesting that Mog1 may affect another process (or processes) in addition to H2B monoubiquitination. Since Mog1 participates in nuclear protein import, we asked whether partial disruption of this pathway might be responsible for the observed genetic interactions and the decreased levels of H2Bub1. To examine this possibility, Bre1 and Rad6 were GFP-tagged and their cellular localization in mog1Δ compared to wild-type cells was monitored using confocal microscopy. Similar to wild-type, both Rad6 and Bre1 showed a nuclear localization in cells lacking MOG1 (Fig EV2A and B). In addition, GFP-tagged Rtf1 and Ubp8 showed a nuclear localization in cells lacking MOG1 (Fig EV2C and D). In summary, these genetic and functional data suggested that Mog1 affects another pathway(s) in addition to H2B monoubiquitination. Click here to expand this figure. Figure EV2. Mog1 is not required for Rad6, Bre1, Rtf1, or Ubp8 nuclear localization A–D. Representative images showing the GFP signal for Rad6-GFP (A), Bre1-GFP (B), Rtf1-GFP (C), and Ubp8-GFP (D) in WT or mog1Δ strains. DAPI (DNA) and BF (bright field) pictures of the same images are shown. In all images, the scale bar corresponds to 5 μm. Download figure Download PowerPoint Global levels of H3K4me3 decrease in the absence of MOG1 H2Bub1 promotes the presence of H3K4me3 at actively transcribing genes 45. The molecular mechanisms behind this crosstalk are complex and are postulated to require multiple factors. H3K4 is methylated by chromatin binding of Set1 and its associated factors (the COMPASS complex), which correlates with productive transcription elongation and mRNA biogenesis 45, 46. In S. cerevisiae, Set1 associates with chromatin even in the absence of H2Bub1; therefore, it was proposed that Set1 chromatin recruitment is not the primary function of H2Bub1 17, 47. However, in S. pombe, H2Bub1 directly enhances the activity of intact Set1C by stabilizing its interaction with chromatin. To determine how H3K4me3 might be affected in the absence of MOG1, differences in global trimethylation levels of H3K4 were analyzed in the presence and absence of MOG1. Western analysis revealed that levels of H3K4me3 were significantly reduced in mog1Δ whole-cell protein extracts compared to wild-type extracts (Fig 2A). Concomitantly, the deletion of MOG1 led to a slight decrease in trimethylation of H3K4 at promoter and 5'ORF regions of ADH1, PMA1, and YEF3 genes as assessed by ChIP analysis (Fig 2B). Figure 2. Mog1 affects trimethylation of H3K4 The expression levels of H3K4me3 and H3 in total extracts of wild-type (WT), mog1Δ, set1Δ, and spp1Δ strains were analyzed by Western blotting using the indicated antibodies. Bar graphs of H3K4me3 levels after H3 normalization show the mean and standard deviations of at least three independent experiments. The P-value was calculated using Student's t-test (*P = 0.01–0.05; ***P < 0.001). ChIP analysis of H3K4me3 presence at ADH1, PMA1, and YEF3 promoter (P) and 5'ORF in wild-type (WT) or mog1Δ strains relative to total H3. The bar graphs indicate the mean and standard deviation for at least three independent experiments. The significance of the differences was calculated using Student's t-test (*P = 0.01–0.05; **P = 0.001–0.01; ***P < 0.001). MOG1 interacts genetically with the H3 methylases Set1 and Set2. Tenfold serial dilutions of the indicated strains were spotted on YPD and incubated for 2 days at the indicated temperatures. Histone H3K4A and H3K4A mog1Δ strains were compared with their isogenic strains as in (C). Download figure Download PowerPoint H2Bub1 not only promotes Set1-dependent histone H3K4 methylation, but also Dot1-dependent H3K79 methylation 48, and it facilitates the Set2-mediated methylation of H3K36 on some intron-containing genes 3. However, global levels of H3K79me3 and of H3K36me3 were not affected by the loss of MOG1 (Fig EV3A and B). Additionally, no effect on H3K4me2 levels was observed by deletion of MOG1 (Fig EV3C). These observations are similar to previous findings that H2B mutations that specifically affect its C-terminal helix (T122R, T122D, and R119A) had no effect on H3K4me2 or on H3K79me2 or me3 levels but did affect H3K4me3 levels 49. Click here to expand this figure. Figure EV3. Mog1 is not required for H3K79me3, H3K36me3, and H3K4me2 methylations The expression levels of H3K79me3 and of total H3 in total extracts from WT, mog1Δ, and bre1Δ cells were analyzed by Western blotting using the indicated antibodies. As in (A) except that set2Δ, WT, and mog1Δ strains were analyzed using an anti-H3K36me3 antibody. As in (A) except that WT, mog1Δ, and rad1Δ strains were analyzed using an anti-H3K4me2 antibody. Download figure Download PowerPoint Next, we tested the potential genetic interaction between Mog1 and the methylases responsible for these modifications. A slow growth phenotype was observed when mog1Δ was combined with set1Δ or set2Δ but not when it was combined with dot1Δ (Fig 2C). Since a pronounced phenotype of mog1Δ cells was a reduction in H3K4me3 levels, we addressed whether the slow growth observed for mog1Δset1Δ was directly related to this specific lysine modification of H3. To this end, we deleted MOG1 in a H3K4A mutant background and observed that the resulting strain was synthetic sick at 33°C (Fig 2D). In summary, these genetic results suggested that the synthetic growth effects observed for mog1Δ in combination with mutants affecting H2B ubiquitination and H3 methylation cannot only be explained by the loss of these modifications. These findings are consistent with the notion that MOG1 deletion disrupts independent pathways that converge on an essential process (or processes) in yeast cells. Mog1 absence affects H2B monoubiquitination-dependent processes such as transcription The above data show that Mog1 was required to sustain correct levels of H2Bub1. H2Bub1 has been implicated in various fundamental cellular functions including the regulation of transcription 50, nucleosome dynamics, and cell size control 51. Among these functions, H2Bub1 promotes the stability and advancement of replication forks by regulating nucleosome stability on newly replicated DNA 52. It has been shown that the non-ubiquitinatable H2B mutant (K123R) and bre1Δ cells are sensitive to hydroxyurea (HU), a replication stress-causing agent 52. To examine whether MOG1 deletion lead toward sensitivity to HU, mog1Δ or bre1Δmog1Δ cells were grown in media containing different concentrations of HU. Notably, mog1Δ cells were sensitive to hydroxyurea, and this sensitivity increased in the double mutant bre1Δmog1Δ (Fig 3A). Figure 3. MOG1 deletion affects sensitivity to hydroxyurea and RNAPII-dependent transcription A. Tenfold serial dilutions of the indicated strains were spotted on YPD containing the indicated concentrations of hydroxyurea (HU) and were incubated for 2–3 days. B, C. (B) mRNA synthesis rates (SR) as determined in Genomic Run-On analysis and (C) mRNA abundance (RA), in mog1Δ compared with wild-type (WT) cells. Bar graphs showing the average level and standard deviation of three experiments (n = 3) for SR and RA values obtained as the median of the whole gene dataset values in arbitrary units (×107) from image analysis quantification. Error bars represent SD. Significance of the differences was obtained using Student's t-test, and statistical difference is considered as *P = 0.01–0.05. The scatter plots (right panels) show the variation of the transcription (B) or mRNA (C) levels of individual genes. Pearson correlation values are −0.039 (SR) and −0.042 (RA). This low correlation and the flat shape of the clouds on scatter plots (see red tendency line) indicates that there is no bias of the transcriptional effect with regard to expression level. D. Box plots of SR and RA median levels of mog1Δ cells compared with WT for all genes (Total), SAGA and TFIID-dominated genes. These data were obtained from three independent replicates (n = 3) of the RA and SR data that were averaged as explained in the main text. The line within the box and the diplayed number represent the median of the whole data set (Total) for 351 (for RA) or 316 (SR) SAGA-dominated genes and for the 3,903 (RA) or 3,403 (SR) TFIID-dominated genes. The box represents the second quartile and the whiskers the first and third quartiles. Significance of the differences was calculated using Student's t-test. No significant difference between the different groups was found. The similar variations in SRs and RAs indicate that there are no changes in mRNA half-lives upon MOG1 deletion (shown in Fig EV4). The total number of genes with confident value in the analyses was 3,953 for RA and 3,989 for SR. Download figure Download PowerPoint Since H2B ubiquitination is a major regulator of transcription 53, we considered whether the absence of MOG1 might interfere with global mRNA transcription. To date, no functional interactions between Mog1 and the transcriptional machinery have been reported. To address such possible interactions, we conducted Genomic Run-On (GRO) experiments 54 to calculate the mRNA synthesis rate (SR, see Materials and Methods) for each transcript in wild-type and mog1Δ cells. In addition to the SR, the mRNA abundance (RA) for each gene was also calculated. These results showed that the global levels of both the SR and RA were significantly lower for mog1Δ compared to wild-type (0.746 and 0.707, respectively, see Fig 3B and C). The quantitatively similar behavior of SR and RA indicates that global mRNA stability was not appreciably different between the two yeast strains (Fig EV4). Moreover, supportive of a general role for Mog1 in affecting the transcriptional rate and mRNA abundance, similar effects were observed for transcription of SAGA- and TFIID-dominated genes (Fig 3D), in agreement with the contribution of both SAGA and TFIID to the expression of nearly all yeast genes 55-58. These findings led us to the conclusion that Mog1 has a genome-wide impact on RNAPII-transcribed mRNAs. Click here to expand this figure. Figure EV4. Global mRNA stability did not change appreciably in the absence of Mog1Box plot showing the relative stability for mRNAs (RS) calculated for 3,450 genes by dividing the mRNA amount data (RA) by the synthesis rate data (SR) used in Fig 3. These data were obtained from three independent replicates (n = 3) of the RA and SR data that were averaged as explained in the main text. The line within the box and the displayed number represent the median of the whole data set (Total) and for 316 SAGA-dominated genes and 3,403 TFIID-dominated genes are shown. The box represents the second quartile and the whiskers the first and third quartiles. Significance of the differences was calculated using Student's t-test. No significant difference between them was found. Download figure Download PowerPoint Mog1 affects the occupancy of H2B ubiquitination-promoting factors on transcribed genes Ubiquitination and deubiquitination of H2B during transcription are complex processes that require the participation of multiple factors 51. Recent reports have shown that one such factor, Rtf1 (a PAFc subunit), acts as a cofactor in H2B ubiquitination by promoting the ability of Bre1 to direct Rad6 to lysines on H2B or by stimulating Rad6 catalytic activity 15. To gain insights into a possible upstream role for Mog1 in H2B ubiquitination, chromatin recruitment of Rad6, Bre1, and Rtf1 to the 5′ and 3′ coding regions of ADH1, PMA1, and YEF3 was examined by ChIP in mog1Δ and wild-type cells. We found that the gene presence of Rad6, Bre1, and Rtf1 was differentially aff
更多查看译文
关键词
rnapii transcription,mrna export,h3k4me3 regulation
AI 理解论文
溯源树
样例
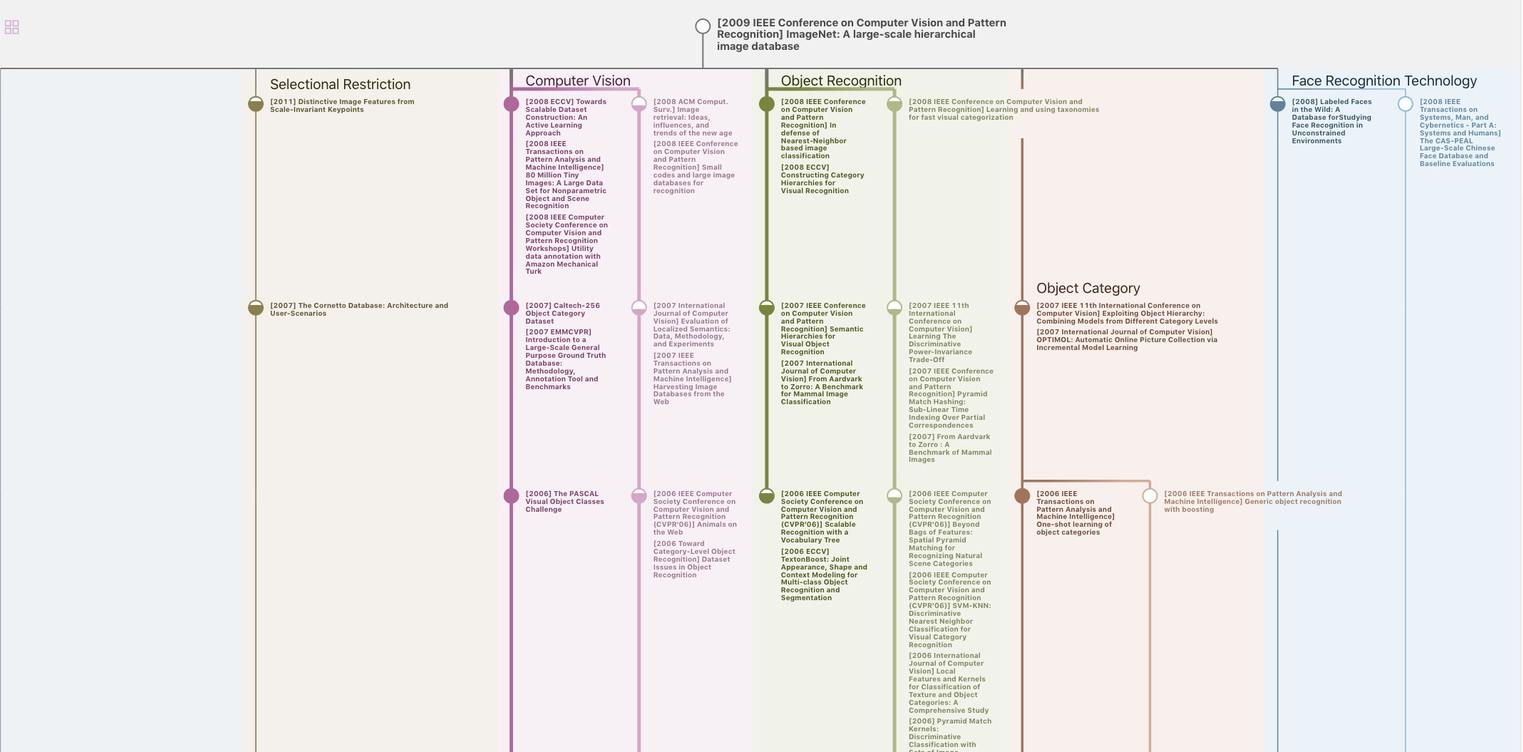
生成溯源树,研究论文发展脉络
Chat Paper
正在生成论文摘要