Rapid parallel acquisition of somatic mutations afterNPM1in acute myeloid leukaemia evolution
British Journal of Haematology(2016)
Abstract
The steps in the progression from subclinical clonal haemopoiesis to acute myeloid leukaemia (AML) have not been defined, nor has its likelihood or latency. NPM1 mutations were not found to drive clonal haemopoiesis in haematologically normal individuals(Genovese et al, 2014; Jaiswal et al, 2014; Xie et al, 2014; McKerrell et al, 2015), highlighting their strong leukaemogenic pedigree, but making them difficult to capture at a stage prior to frank AML. As occasional cases of NPM1-mutated myelodysplastic syndrome/myeloproliferative neoplasm have been described(Caudill et al, 2006; Peng et al, 2016), we hypothesised that detailed analysis of such cases could offer insights into leukaemic evolution after the acquisition of NPM1 mutations. Here, we describe the genetic events driving rapid progression of a case of NPM1 mutant chronic myelomonocytic leukaemia (CMML) to full-blown AML. A 50-year-old woman with an eight-week history of tiredness was found to be anaemic (Hb 75 g/l) and thrombocytopenic (platelet count 116 × 109/l). Her white cell (WCC) (8·7 × 109/l) and neutrophil (5·48 × 109/l) counts were normal but she had monocytosis (1·74 × 109/l). The bone marrow aspirate was haemodilute, but trephine biopsy showed marked myeloid hyperplasia and <5% blasts (Figure S1). The karyotype was normal and a diagnosis of CMML was made. After 83 d she developed fever, abdominal discomfort and a WCC of 209 × 109/l with 82% myeloblasts. A diagnosis of AML was confirmed on bone marrow aspiration (Figure S2) and molecular testing identified NPM1 and FLT3 internal tandem duplication (ITD) mutations. Analysis of CMML DNA identified the NPM1 but not the FLT3-ITD mutation. The subsequent clinical course, summarized in Fig 1, unfortunately ended when our patient succumbed to multiply-relapsed AML 11 months later. To understand the molecular events following acquisition of the NPM1 mutation, we performed exome sequencing [SureSelect Human Exon 50 Mb Kit baits (Agilent, Santa Cara, CA), HiSeq2000 (Illumina, San Diego, CA)] of bone marrow DNA at CMML diagnosis, AML diagnosis and first complete remission. Caveman analysis identified 43 single nucleotide variants (SNV) in AML and 62 in CMML DNA, of which 23 were shared (Tables SI and SII). Pindel analysis identified ten indels in AML and eight in CMML, of which two were shared, including the NPM1 mutation (Table SIII). Interestingly, the 81-nucleotide long FLT3-ITD detected by diagnostic polymerase chain reaction (PCR) in the AML sample was not detected; a well-known limitation of sequencing/bioinformatics pipelines. To contrast the subclonal structures of CMML and AML, we used Dirichlet cluster analysis of variant allele frequencies (VAF) (Bolli et al, 2014). This revealed a cluster of 16 shared mutations present in most CMML and AML cells, including those affecting DNMT3A, TET2 and NPM1 (Fig 2A). By contrast, many other mutations were subclonal and most exhibited different VAFs between CMML and AML, including those affecting SMC3, PTPN11 and NRAS (Fig 2A). CEBPA and FLT3-ITD mutations were not included in the analysis as they are poorly captured by exome sequencing/analysis. We proceeded to study 20 somatic variants, including the eight putative driver mutations described above, using PCR followed by deep sequencing (MiSeq) as described before(Conte et al, 2013) at nine time points (Fig 1) including CMML, AML, remission, relapse and normal control DNA. This gave 65000 × mean coverage at the studied loci (after excluding 6 sample/gene combinations with <1000 reads). As anticipated, PCR-derived VAFs agreed closely with exome data for all genes except CEBPA and FLT3-ITD. The VAFs (Fig 2B) enabled us to determine that DNMT3A and TET2 mutations were acquired first, followed by NPM1 and CEBPA. All four mutations had high VAF in CMML, in keeping with a clonal sweep after acquisition of the last mutation (CEBPA or NPM1) and prior to AML transformation. Other driver mutations were depleted (PTPN11) or enriched (FLT3, NRAS and SMC3) after AML transformation. Mutations affecting NRAS and FLT3 were barely detectable in CMML but present at high VAF in AML, although their VAF in relapse samples indicates that they were present in separate clones. Notably, FLT3-ITD had a VAF of 24% in the AML diagnosis sample, was not detected in the initial relapse and had a VAF of 87% in the final relapse, in keeping with loss-of-heterozygosity (LOH). In fact, copy number analysis of exome data using Allele-specific copy number analysis of tumours (ASCAT, Van Loo et al, 2010) confirmed copy-neutral LOH affecting most of chromosome 13 (including FLT3) in the AML (Figure S3). We describe the molecular events in a case of CMML to AML transformation, a rare instance in which the onward evolution of NPM1 mutant leukaemia could be observed in real time. NPM1 mutations are rarely identified prior to the development of AML, despite the fact that they are not “late” mutations. There are two possible explanations for this, first that NPM1 mutations remain clinically silent for long periods until later mutations lead to the development of AML, but this is unlikely given the absence of NPM1 mutations in the blood of healthy individuals with clonal haemopoiesis(Genovese et al, 2014; Jaiswal et al, 2014; Xie et al, 2014; McKerrell et al, 2015). The alternative explanation is that NPM1 mutations are followed by the rapid acquisition of subsequent mutations, narrowing the time window for detecting them in a preclinical phase. In the case presented here, the acquisition of different leukaemogenic mutations in independent cells within a very short time suggests that, at least in some cases, NPM1 mutations are followed by the rapid acquisition of subsequent events leading to leukaemic transformation. This rapid transformation is in marked contrast to the slow stochastic progression of clones with isolated mutations, such as those found in patients with age-related clonal haemopoiesis(Xie et al, 2014). Importantly, despite the rapid appearance of new mutations, the total numbers of mutations in the AML and CMML exomes were similar, arguing against an increased mutagenesis to explain the phenomenon (Ding et al, 2012). Instead, our observations propose that the number of stem/progenitor cells susceptible to leukaemic transformation was greatly expanded by the NPM1 and/or CEBPA mutation(s), making the overall likelihood of acquisition of subsequent leukaemogenic mutations in one or more stem/progenitor cell(s) very high. In turn this offers an explanation for the frequently subclonal nature of de novo AML(Ding et al, 2012), despite its explosive onset. Also, it argues that eradication of the NPM1-mutant clone is essential for AML eradication. This project was funded by a Leukaemia and Lymphoma Research/British Society for Haematology Clinical Research Training Fellowship to CG and by the Wellcome Trust Sanger Institute (grant number WT098051). GV is funded by a Wellcome Trust Senior Fellowship in Clinical Science (WT095663MA) and work in his laboratory is also funded by the Kay Kendal Leukaemia Fund. I.V. is funded by Spanish Ministerio de Economía y Competitividad subprograma Ramón y Cajal. We would like to thank Servicio Santander Supercomputación for their support. We would like to acknowledge Cambridge Blood and Stem Cell Biobank and the Cancer Molecular Diagnosis Laboratory, Cambridge Biomedical Research Centre (National Institute for Health Reasearch, UK) for help with sample collection and processing. We would like to thank the scientists at the Haematolo-Oncology Diagnostic Centre and Drs Charles Crawley and Jenny Craig, Department of Haematology, Cambridge University Hospitals. Performed laboratory experiments: CSG, NM, AB; Performed bioinformatic analysis: CSG, NB, IV, DW, PVL; Performed moprhology/histology analysis: HE, MVV; Designed the study & wrote the paper: CSG, GV; Supervised the study: GV. The authors have no conflicts of interest to disclose. Fig S1.Bone marrow trephine at the time of CMML diagnosis, showing a hypercellular marrow with myeloid hyperplasia, but with a predominance of differentiated forms and less than 5% blasts. Fig S2. Bone marrow aspirate at the time of AML diagnosis showing a predominance of myeloid blasts. Fig S3. Copy number analysis of exome data using the ASCAT algorirthm15 shows copy-neutral loss-of-heterozygosity of chromosome 13 leading to homozygosity for FLT3-ITD. Table SI. Single nucleotide variants shared by both the CMML and AML samples as detected by Caveman analysis. Table SII. Single nucleotide variants which were unique to either the CMML (blue) or AML (grey) samples. Table SIII. Pindel variants detected in the CMML (blue) and AML (grey) samples. Table SIV. The absolute number and proportion of reads assigned to the variant allele for nine of the mutations at each time point. Please note: The publisher is not responsible for the content or functionality of any supporting information supplied by the authors. Any queries (other than missing content) should be directed to the corresponding author for the article.
MoreTranslated text
Key words
mutations
AI Read Science
Must-Reading Tree
Example
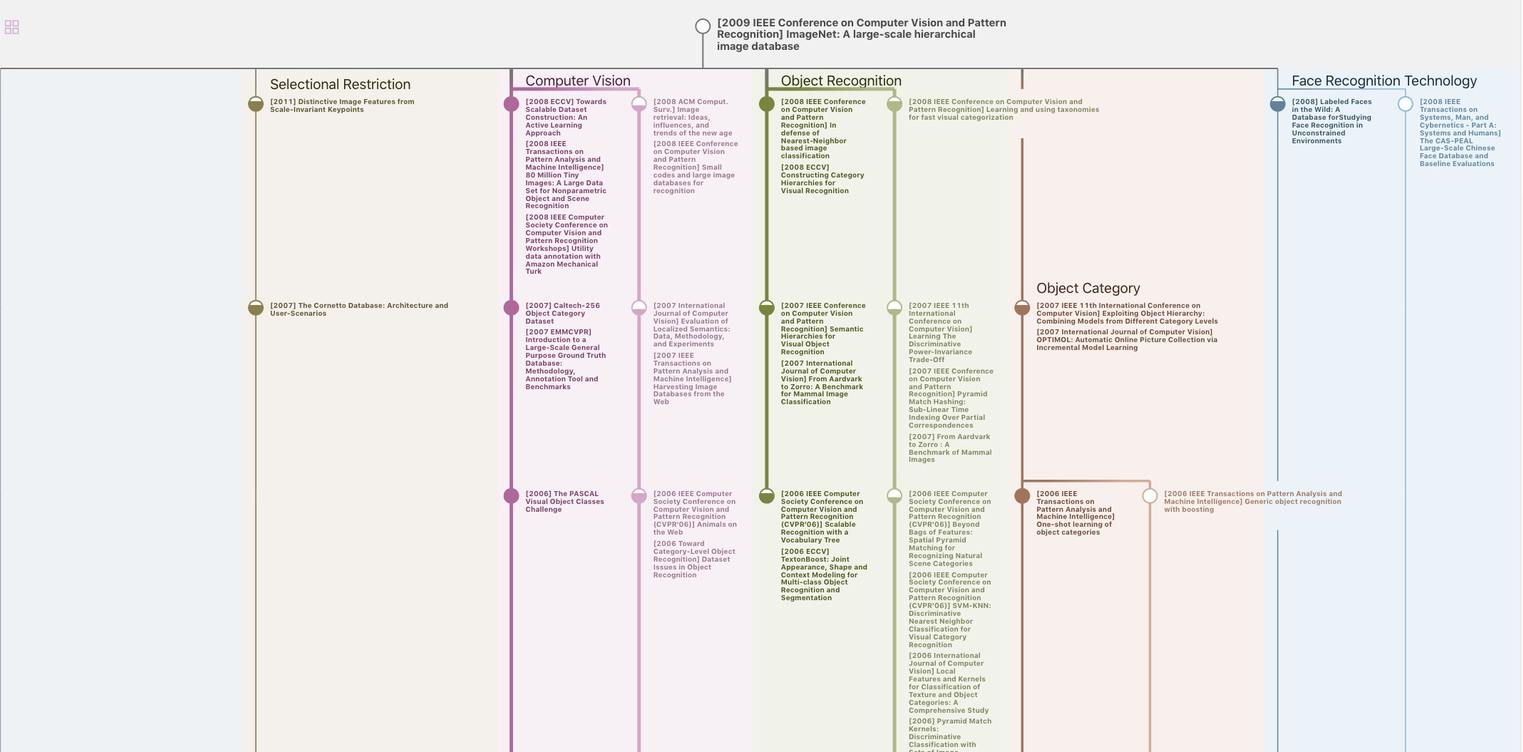
Generate MRT to find the research sequence of this paper
Chat Paper
Summary is being generated by the instructions you defined