Activation of β1,3-N-Acetylglucosaminyltransferase-2 (β3Gn-T2) by β3Gn-T8
Journal of Biological Chemistry(2008)
摘要
Enzymatic activities of some glycosyltransferases are markedly increased via complex formation with other transferases or cofactor proteins. We previously showed that β1,3-N-acetylglucosaminyltransferase-2 (β3Gn-T2) and β3Gn-T8 can form a heterodimer in vitro and that the complex exhibits much higher enzymatic activity than either enzyme alone (Seko, A., and Yamashita, K. (2005) Glycobiology 15, 943–951). Here we examined this activation and the biological significance of complex formation in differentiated HL-60 cells. β3Gn-T2 and -T8 were co-immunoprecipitated from the lysates of both-transfected COS-7 cells, indicating their association in vivo. We prepared inactive mutants of both enzymes by destroying the DXD motifs. The mixture of mutated β3Gn-T2 and intact β3Gn-T8 did not exhibit any activation, whereas the mixture of intact β3Gn-T2 and mutated β3Gn-T8 had increased activity, indicating the activation of β3Gn-T2 via complex formation. Next, we compared expression levels of β3Gn-T1-T8 in HL-60 cells and DMSO-treated differentiated HL-60 cells, which produce larger poly-N-acetyllactosamine chains. The expression level of β3Gn-T8 in the differentiated cells was 2.6-fold higher than in the untreated cells. Overexpression of β3Gn-T8, but not β3Gn-T2, induced an increase in poly-N-acetyllactosamine chains in HL-60 cells. These results raise a possibility that up-regulation of β3Gn-T8 in differentiated HL-60 cells increases poly-N-acetyllactosamine chains by activating intrinsic β3Gn-T2. Enzymatic activities of some glycosyltransferases are markedly increased via complex formation with other transferases or cofactor proteins. We previously showed that β1,3-N-acetylglucosaminyltransferase-2 (β3Gn-T2) and β3Gn-T8 can form a heterodimer in vitro and that the complex exhibits much higher enzymatic activity than either enzyme alone (Seko, A., and Yamashita, K. (2005) Glycobiology 15, 943–951). Here we examined this activation and the biological significance of complex formation in differentiated HL-60 cells. β3Gn-T2 and -T8 were co-immunoprecipitated from the lysates of both-transfected COS-7 cells, indicating their association in vivo. We prepared inactive mutants of both enzymes by destroying the DXD motifs. The mixture of mutated β3Gn-T2 and intact β3Gn-T8 did not exhibit any activation, whereas the mixture of intact β3Gn-T2 and mutated β3Gn-T8 had increased activity, indicating the activation of β3Gn-T2 via complex formation. Next, we compared expression levels of β3Gn-T1-T8 in HL-60 cells and DMSO-treated differentiated HL-60 cells, which produce larger poly-N-acetyllactosamine chains. The expression level of β3Gn-T8 in the differentiated cells was 2.6-fold higher than in the untreated cells. Overexpression of β3Gn-T8, but not β3Gn-T2, induced an increase in poly-N-acetyllactosamine chains in HL-60 cells. These results raise a possibility that up-regulation of β3Gn-T8 in differentiated HL-60 cells increases poly-N-acetyllactosamine chains by activating intrinsic β3Gn-T2. Glycosyltransferases are present in the endoplasmic reticulum/Golgi membranes, cytoplasm, cell surface, and body fluids. In the presence of appropriate sugar donors, they work for the biosynthesis of various glycoconjugates. Recently, it has been shown that some glycosyltransferases form protein complexes with other glycosyltransferases and/or non-glycosyltransferase proteins (reviewed in Ref. 1Seko A. Trends Glycosci. Glycotechnol. 2006; 18: 209-230Crossref Scopus (7) Google Scholar). Complex formation contributes to enzymatic activation, stable expression in the Golgi apparatus, correct localization in intracellular vesicles, efficient biosynthesis of glycan chains, and modification of substrate specificities. Enzymatic activation has been proven for protein O-mannosyltransferases (2Gentzsch M. Immervoll T. Tanner W. FEBS Lett. 1995; 377: 128-130Crossref PubMed Scopus (43) Google Scholar, 3Manya H. Chiba A. Yoshida A. Wang X. Chiba Y. Jigami Y. Margolis R.U. Endo T. Proc. Natl. Acad. Sci. U. S. A. 2004; 101: 500-505Crossref PubMed Scopus (312) Google Scholar, 4Ichimiya T. Manya H. Ohmae Y. Yoshida H. Takahashi K. Ueda R. Endo T. Nishihara S. J. Biol. Chem. 2004; 279: 42638-42647Abstract Full Text Full Text PDF PubMed Scopus (87) Google Scholar), N-acetylglucosaminyltransferases and glucuronyltransferases involved in heparan sulfate biosynthesis (5McCormick C. Duncan G. Goutsos K.T. Tufaro F. Proc. Natl. Acad. Sci. U. S. A. 2000; 97: 668-673Crossref PubMed Scopus (363) Google Scholar, 6Senay C. Lind T. Muguruma K. Tone Y. Kitagawa H. Sugahara K. Lidholt K. Lindahl U. Kusche-Gullberg M. EMBO Rep. 2000; 1: 282-286Crossref PubMed Scopus (139) Google Scholar, 7Kim B.-T. Kitagawa H. Tanaka J. Tamura J. Sugahara K. J. Biol. Chem. 2003; 278: 41618-41623Abstract Full Text Full Text PDF PubMed Scopus (67) Google Scholar, 8Han C. Belenkaya T.Y. Khodoun M. Tauchi M. Lin X. Lin X. Development. 2004; 131: 1563-1575Crossref PubMed Scopus (186) Google Scholar, 9Izumikawa T. Egusa N. Taniguchi F. Sugahara K. Kitagawa H. J. Biol. Chem. 2006; 281: 1929-1934Abstract Full Text Full Text PDF PubMed Scopus (26) Google Scholar), ST8Sia-I (GD3 synthase) and β4GalNAc-T1 (GM2/GD2 synthase) (10Bieberich E. MacKinnon S. Silva J. Li D.D. Tencomnao T. Irwin L. Kapitonov D. Yu R.K. Biochemistry. 2002; 41: 11479-11487Crossref PubMed Scopus (42) Google Scholar), and chondroitin synthase (11Kitagawa H. Izumikawa T. Uyama T. Sugahara K. J. Biol. Chem. 2003; 278: 23666-23671Abstract Full Text Full Text PDF PubMed Scopus (140) Google Scholar, 12Izumikawa T. Kitagawa H. Mizuguchi S. Nomura K.H. Nomura K. Tamura J. Gengyo-Ando K. Mitani S. Sugahara K. J. Biol. Chem. 2004; 279: 53755-53761Abstract Full Text Full Text PDF PubMed Scopus (59) Google Scholar, 13Izumikawa T. Uyama T. Okuura Y. Sugahara K. Kitagawa H. Biochem. J. 2007; 403: 545-552Crossref PubMed Scopus (84) Google Scholar). In these cases, glycosyltransferases exhibit little enzymatic activity when expressed alone, but their catalytic activities emerge if their respective cofactor proteins are simultaneously expressed. Because the activation does not occur by in vitro mixing of the glycosyltransferases and cofactor proteins, the process of complex formation appears to involve intermolecular disulfide bond formation or complicated interactions during early stages of polypeptide synthesis. In contrast, we previously found that β1,3-N-acetylglucosaminyltransferase-2 (β3Gn-T2) 2The abbreviations used are: β3Gn-T, β1,3-N-acetylglucosaminyltransferase; Fuc, fucose; Gal, galactose; GlcNAc, N-acetylglucosamine; GnT, β-N-acetylglucosaminyltransferase; lamps, lysosomal membrane glycoproteins; Man, mannose; polyLacNAc, poly-N-acetyllactosamine; tetraGP, Galβ1→4GlcNAcβ1→2(Galβ1→4GlcNAcβ1→4)Manα1→3[Galβ1→4GlcNAcβ1→2(Galβ1→4GlcNAcβ1→6)Manα1→6]Manβ1→4GlcNAc. 2The abbreviations used are: β3Gn-T, β1,3-N-acetylglucosaminyltransferase; Fuc, fucose; Gal, galactose; GlcNAc, N-acetylglucosamine; GnT, β-N-acetylglucosaminyltransferase; lamps, lysosomal membrane glycoproteins; Man, mannose; polyLacNAc, poly-N-acetyllactosamine; tetraGP, Galβ1→4GlcNAcβ1→2(Galβ1→4GlcNAcβ1→4)Manα1→3[Galβ1→4GlcNAcβ1→2(Galβ1→4GlcNAcβ1→6)Manα1→6]Manβ1→4GlcNAc. and β3Gn-T8 can form a heterodimer in vitro and that the enzymatic activity of the dimer is much higher than the sum of the individual activities (14Seko A. Yamashita K. Glycobiology. 2005; 15: 943-951Crossref PubMed Scopus (48) Google Scholar). Because in vitro mixing of individually expressed fractions of β3Gn-T2 and -T8 is sufficient for enzymatic activation, dimer formation and enzymatic activation should occur with the completely folded proteins. However, it has been unclear whether the two enzymes associate with each other in vivo and which of the one or more enzymes are catalytically activated in the complex.Poly-N-acetyllactosamine (polyLacNAc) is a linear glycan chain consisting of repeating N-acetyllactosamine units (Galβ1–4GlcNAcβ1–3)n. The glycan chains occur in glycosphingolipids and N-linked/O-linked glycan chains of specific glycoproteins. In some cases, the 3-OH and/or 6-OH of galactose (Gal) and N-acetylglucosamine (GlcNAc) residues are modified by sialic acids, fucose (Fuc), and/or sulfate residues, which serve as determinants for various carcinoembryonic antigens and ligands for various cell recognition-associated lectins (15Varki A. Cummings R. Esko J. Freeze H. Hart G. Marth J. Essentials of Glycobiology. Cold Spring Harbor Laboratory Press, Cold Spring Harbor, NY1999: 211-253Google Scholar). In HL-60 cells, polyLacNAc chains exist primarily on lysosomal membrane glycoproteins (lamps) (16Carlsson S.R. Roth J. Piller F. Fukuda M. J. Biol. Chem. 1988; 263: 18911-18919Abstract Full Text PDF PubMed Google Scholar). HL-60 cells can differentiate to granulocytic cells in the presence of DMSO. Lee et al. (17Lee N. Wang W.-C. Fukuda M. J. Biol. Chem. 1990; 265: 20476-20487Abstract Full Text PDF PubMed Google Scholar) showed that polyLacNAc chains increase in the DMSO-treated differentiated HL-60 cells. They also showed that β3Gn-T activities in the differentiated HL-60 cells are 1.5-fold higher than those in the undifferentiated cells, suggesting that β3Gn-T is a rate-limiting enzyme for the biosynthesis of longer polyLacNAc chains. This linear glycan is biosynthesized by the repeating action of β1,4-galactosyltransferase and β3Gn-T. β3Gn-T1, -T2, -T3, -T4, -T7, and -T8 have been shown to possess the ability to synthesize polyLacNAc chains (14Seko A. Yamashita K. Glycobiology. 2005; 15: 943-951Crossref PubMed Scopus (48) Google Scholar, 18Sasaki K. Kurata-Miura K. Ujita M. Angata K. Nakagawa S. Sekine S. Nishi T. Fukuda M. Proc. Natl. Acad. Sci. U. S. A. 1997; 94: 14294-14299Crossref PubMed Scopus (133) Google Scholar, 19Shiraishi N. Natsume A. Togayachi A. Endo T. Akashima T. Yamada Y. Imai N. Nakagawa S. Koizumi S. Sekine S. Narimatsu H. Sasaki K. J. Biol. Chem. 2001; 276: 3498-3507Abstract Full Text Full Text PDF PubMed Scopus (116) Google Scholar, 20Ishida H. Togayachi A. Sakai T. Iwai T. Hiruma T. Sato T. Okubo R. Inaba N. Kudo T. Gotoh M. Shoda J. Tanaka N. Narimatsu H. FEBS Lett. 2005; 579: 71-78Crossref PubMed Scopus (85) Google Scholar, 21Seko A. Yamashita K. FEBS Lett. 2004; 556: 216-220Crossref PubMed Scopus (38) Google Scholar). However, it is unclear which of the one or more enzymes are responsible for the increase in polyLacNAc chains in the differentiated HL-60 cells.In this study, we have addressed the following issues: whether β3Gn-T2 and -T8 associate with each other in vivo; whether β3Gn-T2 and/or -T8 are catalytically activated in a complexed state; and which one or more β3Gn-Ts are associated with the increase in polyLacNAc chains in differentiated HL-60 cells.EXPERIMENTAL PROCEDURESMaterials—UDP-[6-3H]GlcNAc (2.2 TBq/mmol) was purchased from American Radiolabeled Chemicals Inc. (St. Louis, MO). A tetra-antennary oligosaccharide, Galβ1→4GlcNAcβ1→2(Galβ1→4GlcNAcβ1→4)Manα1→3[Galβ1→4GlcNAcβ1→2(Galβ1→4GlcNAcβ1→6)Manα1→6]Manβ1→4GlcNAc (tetraGP), was obtained from the urine of GM1 gangliosidosis patients (22Yamashita K. Ohkura T. Okada S. Yabuuchi H. Kobata A. J. Biol. Chem. 1981; 256: 4789-4798Abstract Full Text PDF PubMed Google Scholar).Expression of Tagged and Mutated Human β3Gn-T2 and β3Gn-T8—An expression vector for C-terminally FLAG-tagged β3Gn-T2 was prepared from the p3XFLAG-CMV™-14 vector (Sigma-Aldrich) and the cDNA fragment for full-length β3Gn-T2 without a stop codon. The fragment was amplified by PCR from pcDNA3-β3Gn-T2 (21Seko A. Yamashita K. FEBS Lett. 2004; 556: 216-220Crossref PubMed Scopus (38) Google Scholar). The oligonucleotide primers used were 5′-tttaagcttGAGAAATGAGTGTTGGA-3′ (forward primer) and 5′-ttttctagaGCATTTTAAATGAGCACT-3′ (reverse primer). The cDNA was cloned into p3XFLAG-CMV™-14 between the HindIII and XbaI sites. The resulting plasmid (pFLAG-T2) was sequenced with a Prism 310 Genetic Analyzer. C-terminally myc-tagged β3Gn-T8 (pMyc-T8) was prepared similarly. The cDNA fragment was amplified by PCR from pcDNA3-β3Gn-T8 (14Seko A. Yamashita K. Glycobiology. 2005; 15: 943-951Crossref PubMed Scopus (48) Google Scholar). The oligonucleotide primers used were 5′-tttaagctt-GGGTCATGCGCTGCCCCAAGTG-3′ (forward primer) and 5′-ttttctagaGCACTGGAGCCTTGGGT-3′ (reverse primer). The cDNA was cloned into the pcDNA4/myc-His® expression vector (Invitrogen) between the HindIII and XbaI sites. Expression vectors for C-terminally FLAG-tagged β3Gn-T8 (pFLAG-T8) and C-terminally myc-tagged β3Gn-T2 (pMyc-T2) were obtained from pMyc-T8 and pFLAG-T2, respectively, by HindIII and XbaI digestion and ligation into the respective plasmids.Pichia pastoris expression vectors for the truncated forms of human β3Gn-T2 and β3Gn-T8, lacking cytoplasmic and transmembrane domains, were prepared previously (14Seko A. Yamashita K. Glycobiology. 2005; 15: 943-951Crossref PubMed Scopus (48) Google Scholar). β3Gn-T2 and β3Gn-T8 mutated in their DXD motifs were prepared using a QuikChange™ site-directed mutagenesis kit (Stratagene). The mutagenic oligonucleotide primers used were 5′-CTGAGTTTGTTTTCAAGGGCGCTGACGATGTTTTTGTG-3′ (forward primer for β3Gn-T2), 5′-CACAAAAACATCGTCAGCGCCCTTGAAAACAAACTCAG-3′ (reverse primer for β3Gn-T2), 5′-GAGTTTTGTCTTGCGAGCTGCGGACGATGCCTTTGTAC-3′ (forward primer for β3Gn-T8), and 5′-GTACAAAGGCATCGTCCGCAGCTCGCAAGACAAAACTC-3′ (reverse primer for β3Gn-T8). Mutated plasmids were used for the transformation of P. pastoris KM71 cells, and the recombinant proteins were purified from the culture media as described previously (14Seko A. Yamashita K. Glycobiology. 2005; 15: 943-951Crossref PubMed Scopus (48) Google Scholar). The yields for recombinant human β3Gn-T2, β3Gn-T8, mutated β3Gn-T2 (T2-DA), and mutated β3Gn-T8 (T8-QA) from 100 ml of buffered methanol-complex media were 17, 130, 2.1, and 94 μg, respectively.The cDNA fragments for the truncated forms of murine β3Gn-T2 and β3Gn-T8 were amplified by PCR from the genomic DNA from NIH 3T3 cells, because both were encoded in each single exon. The oligonucleotide primers used were 5′-TTTGAATTCTCCAAAAACAGTAGCCAAGAC-3′ (forward primer for β3Gn-T2), 5′-TTTGCGGCCGCAGCTCATGTCTATTTCAGCA-3′ (reverse primer for β3Gn-T2), 5′-TTTGAATTCAAAAAGGCTGAACCCCGGGGC-3′ (forward primer for β3Gn-T8), and 5′-TTTGCGGCCGCCGGTCAGCACTGGAGCTC-3′ (reverse primer for β3Gn-T8). The cDNA was cloned into pPIC9 expression vector (Invitrogen). The recombinant proteins were prepared as described previously (14Seko A. Yamashita K. Glycobiology. 2005; 15: 943-951Crossref PubMed Scopus (48) Google Scholar). The yields for recombinant murine β3Gn-T2 and β3Gn-T8 from 100 ml of buffered methanol-complex media were 5.6 and 8.6 μg, respectively. Peptide:N-glycosidase F (Takara Bio Inc., Otsu, Japan) digestion was performed according to the manufacturer's instructions.Assay of β3Gn-T Enzymatic Activity—The assay conditions were as described previously (21Seko A. Yamashita K. FEBS Lett. 2004; 556: 216-220Crossref PubMed Scopus (38) Google Scholar). Briefly, the reaction mixtures (20 μl) consisted of 50 mm HEPES-NaOH (pH 7.2), 10 mm MnCl2, 0.1% (w/v) Triton X-100, 0.3 mm tetraGP, 2.5 μm UDP-[3H]GlcNAc (6.7 × 106 dpm), 50 μg/ml protamine chloride, 0.5 mm spermine, and appropriately diluted enzyme fractions. The mixtures were incubated at 37 °C for 1 h. The 3H-labeled products were separated by paper electrophoresis (pyridine:acetic acid:water, 3:1:387, pH 5.4) and then by paper chromatography (pyridine:ethyl acetate:acetic acid:water, 5:5:1:3). After drying, the paper was monitored for radioactivity with a radiochromatogram scanner.Co-immunoprecipitation—The plasmids (5 μg) pFLAG-T2 and pFLAG-T8 were transfected into semi-confluent COS-7 cells on 10-cm dishes using 20 μg of Lipofectamine 2000 (Invitrogen) according to the manufacturer's instructions. Stable transfectants were isolated by selection using 400 μg/ml G418 sulfate (Calbiochem, Darmstadt, Germany). A second transfection for transient expression was performed with 10 μg of the appropriate plasmids and Lipofectamine 2000. The cells were harvested after 24 h and washed twice with phosphate-buffered saline. After adding 1 ml of lysis buffer (50 mm Tris-HCl (pH 7.5), 0.15 m NaCl, 1% (v/v) Nonidet P-40, 1 mm phenylmethanesulfonyl fluoride, 1 μg/ml pepstatin, and 1 μg/ml leupeptin), the cell pellets were suspended and lysed on ice for 20 min. After centrifugation, the supernatants were collected and incubated with 1 μg of anti-myc antibody (Invitrogen) and 15 μl of Protein G-Sepharose™ 4 Fast Flow (GE Healthcare, Buckinghamshire, England) at 4 °C for 1 h. The resins were washed with the lysis buffer four times. Equal aliquots of the resins were used for SDS-PAGE, and the proteins were transferred onto a nitrocellulose membrane (Bio-Rad Trans-Blot® Transfer Medium, Hercules, CA). Tag-conjugated proteins on the membranes were treated with horseradish peroxidase)-conjugated anti-FLAG-M2 antibody (0.3 μg/ml) (Sigma-Aldrich) or horseradish peroxidase-conjugated anti-myc antibody (1 μg/ml, Invitrogen) and detected with ECL Western blotting Detection Reagents (GE Healthcare).Reverse Transcription-PCR for β3Gn-Ts—HL-60 cells (Health Science Research Resources Bank, Sennan, Japan) were differentiated by 1.5%-DMSO treatment for 3 days. The cDNAs were synthesized from total RNA using SuperScript III reverse transcriptase (Invitrogen). Oligonucleotide primers used for PCR were 5′-AGGTCTTTGACAAGCTAGCCAGG-3′ (forward primer for β3Gn-T1), 5′-TCCTGCCACGTAGAATGGCTCC-3′ (reverse primer for β3Gn-T1), 5′-CCACCCCGACCTTTCAGATATGC-3′ (forward primer for β3Gn-T2), 5′-ACCTGGTCAGTGATATGGTACAGC-3′ (reverse primer for β3Gn-T2), 5′-CACGAGGCCCGCAAGGTCAAC-3′ (forward primer for β3Gn-T3), 5′-GACAGGCGTTGCGATGGAGCC-3′ (reverse primer for β3Gn-T3), 5′-GATCCGCTCCCCCAGCCCAG-3′ (forward primer for β3Gn-T4), 5′-CATCTTCCATGATAGCCTGGAGG-3′ (reverse primer for β3Gn-T4), 5′-TCCACTGGAGGGAGAAGAACTAC-3′ (forward primer for β3Gn-T5), 5′-GCAGGGATGATAAGGAGTTTTACC-3′ (reverse primer for β3Gn-T5), 5′-GGGGCAAGAGCGCAGCTACGG-3′ (forward primer for β3Gn-T6), 5′-CTGTGCGCCAGGCAGCTGCAC-3′ (reverse primer for β3Gn-T6), 5′-AGGAGCGCACGCACTACCAGC-3′ (forward primer for β3Gn-T7), 5′-CAGGGTGTCGCAGGCATGGTG-3′ (reverse primer for β3Gn-T7), 5′-GGCCTGACCTAGACTCACTAGTG-3′ (forward primer for β3Gn-T8), and 5′-CGCAGTGCGGTCTGCTGGCCAG-3′ (reverse primer for β3Gn-T8). PCR was performed under following conditions: 28 cycles consisting of denaturation at 94 °C for 30 s, annealing at 55 °C for 1 min, and elongation at 72 °C for 2 min. After agarose-gel electrophoresis, the amplified products were quantified using an FLA-2000 multi-imager (Fuji Photo Film, Japan).Tomato-lectin Blotting—The plasmids (10 μg) were transfected into HL-60 cells (1 × 106) using 20 μg of Lipofectamine 2000 according to the manufacturer's instructions. After 48 h, the cells were collected and washed with phosphate-buffered saline three times. Aliquots of cell homogenates (3 μg of protein) were used for SDS-PAGE, and the proteins were transferred onto a nitrocellulose membrane. Parts of membranes were digested with Escherichia freundii endo-β-galactosidase (Seikagaku Co., Tokyo, Japan) (50 milliunits of enzyme in 0.1% bovine serum albumin-50 mm sodium acetate buffer (pH 5.5) at 25 °C for 16 h). After blocking with 1% bovine serum albumin/phosphate-buffered saline, the membrane was treated with 10 μg/ml biotin-conjugated lectin from Lycopersicon esculentum (tomato, Sigma-Aldrich) in phosphate-buffered saline/0.1% Tween 20 at 4 °C for 2 h. After washing, the membrane was treated with horseradish peroxidase-conjugated streptavidin (GE Healthcare) at 4 °C for 1 h. The detection was performed using ECL Western blotting Detection Reagents. Chemiluminescence was quantified using a LAS-1000 multi-imager (Fuji Photo Film, Japan).RESULTSβ3Gn-T2 Associates with β3Gn-T8 in Vivo—We previously found that the mixture of β3Gn-T2 and β3Gn-T8 exhibited much higher enzymatic activity than either enzyme alone and that the two enzymes could form a heterodimer in vitro (14Seko A. Yamashita K. Glycobiology. 2005; 15: 943-951Crossref PubMed Scopus (48) Google Scholar). However, it remained unclear whether the two enzymes interacted with each other in vivo. To assess this, co-immunoprecipitation was performed. β3Gn-T2 FLAG tagged at the C terminus (T2-FL) and β3Gn-T8 myc tagged at the C terminus (T8-myc) were simultaneously expressed in COS-7 cells, and the cell lysates were immunoprecipitated by anti-myc antibody. The precipitates were analyzed by Western blotting (Fig. 1A). T2-FL co-immunoprecipitated with T8-myc and was detected by anti-FLAG antibody, indicating that β3Gn-T2 associates with β3Gn-T8 in vivo.β3Gn-T2 Is Activated by β3Gn-T8—Increased enzymatic activity in the mixture of β3Gn-T2 and -T8 indicated that the enzyme(s) were activated in the complex. To assess which β3Gn-T was activated, we prepared mutated proteins that were enzymatically inactive, but could form the complex. Glycosyltransferases generally have a DXD motif (23Wiggins C.A.R. Munro S. Proc. Natl. Acad. Sci. U. S. A. 1998; 95: 7945-7950Crossref PubMed Scopus (315) Google Scholar), which is involved in binding to divalent cations and sugar nucleotides and is thus essential for their catalytic activities. β3Gn-T2 and -T8 contain 245DDD247 and 246QDD248, respectively, as DXD motifs. We constructed expression vectors with mutated β3Gn-T2 (T2-DA) and -T8 (T8-QA), which had 245ADD247 and 246ADD248, respectively. The ability of T2-DA and T8-QA to form a complex in vivo was examined by co-immunoprecipitation as above. Fig. 1 (B and C) shows that the substitutions in their DXD motifs did not affect their ability to interact with their wild-type counterpart. Next, we examined the enzymatic activities of T2-DA, T8-QA, and 1:1 mixtures with wild-type β3Gn-T2 and -T8. Soluble forms of (His)6-tagged T2-DA and T8-QA were produced using with the P. pastoris protein expression system, as previously described (14Seko A. Yamashita K. Glycobiology. 2005; 15: 943-951Crossref PubMed Scopus (48) Google Scholar), and purified by Ni-NTA agarose chromatography (Fig. 2). Both mutant proteins electrophoresed as smeared bands, similar to the wild-type proteins. Peptide:N-glycosidase F treatment showed that the polypeptide moieties of T2-DA and T8-QA had the same molecular masses (∼45 kDa) as the wild-type enzymes (Fig. 2). The smeared profiles were attributed to heterogeneity in yeast large N-linked glycans. The enzymatic activities are shown in Fig. 3. Neither T2-DA nor T8-QA had any enzymatic activity, indicating that the DXD motifs are required for catalytic activity. The mixture of wild-type β3Gn-T2 and T8-QA had 6.3-fold higher enzymatic activity (1.9 ± 0.05 pmol/min) than β3Gn-T2 alone (0.30 ± 0.04 pmol/min), whereas the mixture of wild-type β3Gn-T8 and T2-DA had the same level of activity as β3Gn-T8 alone (0.014 ± 0.002 pmol/min). The same results were obtained from another independent experiment using individually prepared enzymes. These results indicate that β3Gn-T2 is activated by β3Gn-T8, and that the mutant T8-QA, which has no enzymatic activity, is also able to activate β3Gn-T2.FIGURE 2SDS-PAGE of soluble forms of β3Gn-T2 (T2), β3Gn-T8 (T8), and the mutated proteins, T2-DA and T8-QA, produced by P. pastoris. Purified proteins were applied to the gel. After electrophoresis, the proteins were detected by Sypro Orange staining. The digests by peptide:N-glycosidase F (PNGase F) are also shown in the right four lanes.View Large Image Figure ViewerDownload Hi-res image Download (PPT)FIGURE 3Specific activities of β3Gn-T2 (T2), β3Gn-T8 (T8), T2-DA, T8-QA, and their combinations. Each protein (7 ng) was assayed for enzymatic activity as described under “Experimental Procedures.” Tetra-antennary N-linked oligosaccharide was used as an acceptor substrate. The enzymatic activities are the means of five independent experiments. The minimal detectable amount of the activity was 0.005 pmol/min.View Large Image Figure ViewerDownload Hi-res image Download (PPT)Next, we examined whether murine β3Gn-T8 could activate murine and human β3Gn-T2. Amino acid similarities for catalytic domains of β3Gn-T2 and β3Gn-T8 between human and murine were 87 and 75%, respectively. As shown in Table 1, the Vmax/Km value of the mixture, murine β3Gn-T2 and murine β3Gn-T8, was approximately twice higher than that of murine β3Gn-T2 alone. Interestingly, murine β3Gn-T8 could activate human β3Gn-T2, although the degree of the activation by murine β3Gn-T8 was lower than that by human β3Gn-T8. In converse, human β3Gn-T8 could also activate murine β3Gn-T2. These results indicate that the ability of β3Gn-T8 to activate β3Gn-T2 is conserved between human and murine.TABLE 1Kinetic analysis of human β3Gn-T2 (H2), human β3Gn-T8 (H8), murine β3Gn-T2 (M2), murine β3Gn-T8 (M8), and the mixtures of each two enzymeH2H2+H8H2+M8M2M2+M8M2+H8Km (mm)0.420.150.250.330.0710.18Vmax (nmol/min/mg of protein)15061039017074360Vmax/Km3604100160052010002000 Open table in a new tab Furthermore, to assess whether β3Gn-T8 could stabilize β3Gn-T2 or not, the stability of the enzymatic activities of human β3Gn-T2 in the presence or absence of human β3Gn-T8 was examined. As shown in Fig. 4, the enzymatic activities of β3Gn-T2 alone and the mixture of β3Gn-T2 and β3Gn-T8 decreased in the same manner. This suggests that β3Gn-T8 does not have the ability to stabilize the enzymatic activity of β3Gn-T2 at least in vitro.FIGURE 4Stability of the enzymatic activities of human β3Gn-T2 (closed circle) and the mixture of human β3Gn-T2 and β3Gn-T8 (open circle). Equal amounts of enzyme proteins were incubated in 10 mm HEPES-NaOH, 0.15 m NaCl (pH 7.2) at 37 °C, and at the indicated time, aliquots of the enzyme solution were assayed for the β3Gn-T activity. Four independent experiments were performed.View Large Image Figure ViewerDownload Hi-res image Download (PPT)β3Gn-T8 Is Involved in Increasing PolyLacNAc Chains in the Differentiated HL-60 Cells—By structural studies for N-linked glycan chains of lamps, Lee et al. (17Lee N. Wang W.-C. Fukuda M. J. Biol. Chem. 1990; 265: 20476-20487Abstract Full Text PDF PubMed Google Scholar) showed that the amount of polyLacNAc chains in HL-60 cells increased with differentiation by DMSO treatment. They also assayed the enzymatic activities of β3Gn-Ts, β1,6-N-acetylglucosaminyltransferase-V, and β,4-galactosyltransferases, and found that only β3Gn-T activity increased 1.6-fold in the differentiated HL-60 cells using a linear substrate Galβ1→4GlcNAcβ1→6Manα1→6Manβ1→R. We assayed β3Gn-T activity in these cells using tetraGP, which is a good substrate for all β3Gn-Ts (14Seko A. Yamashita K. Glycobiology. 2005; 15: 943-951Crossref PubMed Scopus (48) Google Scholar, 20Ishida H. Togayachi A. Sakai T. Iwai T. Hiruma T. Sato T. Okubo R. Inaba N. Kudo T. Gotoh M. Shoda J. Tanaka N. Narimatsu H. FEBS Lett. 2005; 579: 71-78Crossref PubMed Scopus (85) Google Scholar, 21Seko A. Yamashita K. FEBS Lett. 2004; 556: 216-220Crossref PubMed Scopus (38) Google Scholar). As shown in Table 2, the β3Gn-T activities in HL-60 cells and in DMSO-treated HL-60 cells were 6.5 ± 0.7 and 35 ± 1.8 pmol/min/mg of protein, respectively, indicating that the activity increased 5.3-fold in the course of differentiation. To assess which β3Gn-Ts were up-regulated in the process of differentiation of HL-60 cells, we compared expression levels of transcripts for β3Gn-Ts in HL-60 cells and DMSO-treated HL-60 cells. It has been shown previously that β3Gn-T1, -T2, -T3, -T4, -T7, and -T8 are able to synthesize poly-LacNAc chains (14Seko A. Yamashita K. Glycobiology. 2005; 15: 943-951Crossref PubMed Scopus (48) Google Scholar, 18Sasaki K. Kurata-Miura K. Ujita M. Angata K. Nakagawa S. Sekine S. Nishi T. Fukuda M. Proc. Natl. Acad. Sci. U. S. A. 1997; 94: 14294-14299Crossref PubMed Scopus (133) Google Scholar, 19Shiraishi N. Natsume A. Togayachi A. Endo T. Akashima T. Yamada Y. Imai N. Nakagawa S. Koizumi S. Sekine S. Narimatsu H. Sasaki K. J. Biol. Chem. 2001; 276: 3498-3507Abstract Full Text Full Text PDF PubMed Scopus (116) Google Scholar, 20Ishida H. Togayachi A. Sakai T. Iwai T. Hiruma T. Sato T. Okubo R. Inaba N. Kudo T. Gotoh M. Shoda J. Tanaka N. Narimatsu H. FEBS Lett. 2005; 579: 71-78Crossref PubMed Scopus (85) Google Scholar, 21Seko A. Yamashita K. FEBS Lett. 2004; 556: 216-220Crossref PubMed Scopus (38) Google Scholar). As shown in Fig. 5, substantial expression was detected for β3Gn-T1, -T2, -T4, -T5, -T7, and -T8 in HL-60 cells (Fig. 5, U). Among these, transcript levels increased for only β3Gn-T2 and -T8 (1.5- and 2.6-fold, respectively) after DMSO treatment. This result suggests that the increased expression level of either β3Gn-T2 or -T8 is responsible for the increase in β3Gn-T activity. Next we examined whether the expression level of polyLacNAc chains increased with overexpression of β3Gn-T2 or -T8 in HL-60 cells. Expression vectors for β3Gn-T2 and -T8 were transiently transfected into HL-60 cells, and the cell homogenates were subjected to tomato-lectin blotting analysis. Because this lectin recognizes three or more linear units of repeating LacNAc (24Merkle R.K. Cummings R.D. J. Biol. Chem. 1987; 262: 8179-8189Abstract Full Text PDF PubMed Google Scholar), it is suitable for detection of rather long polyLacNAc chain
更多查看译文
关键词
n-acetylglucosaminyltransferase
AI 理解论文
溯源树
样例
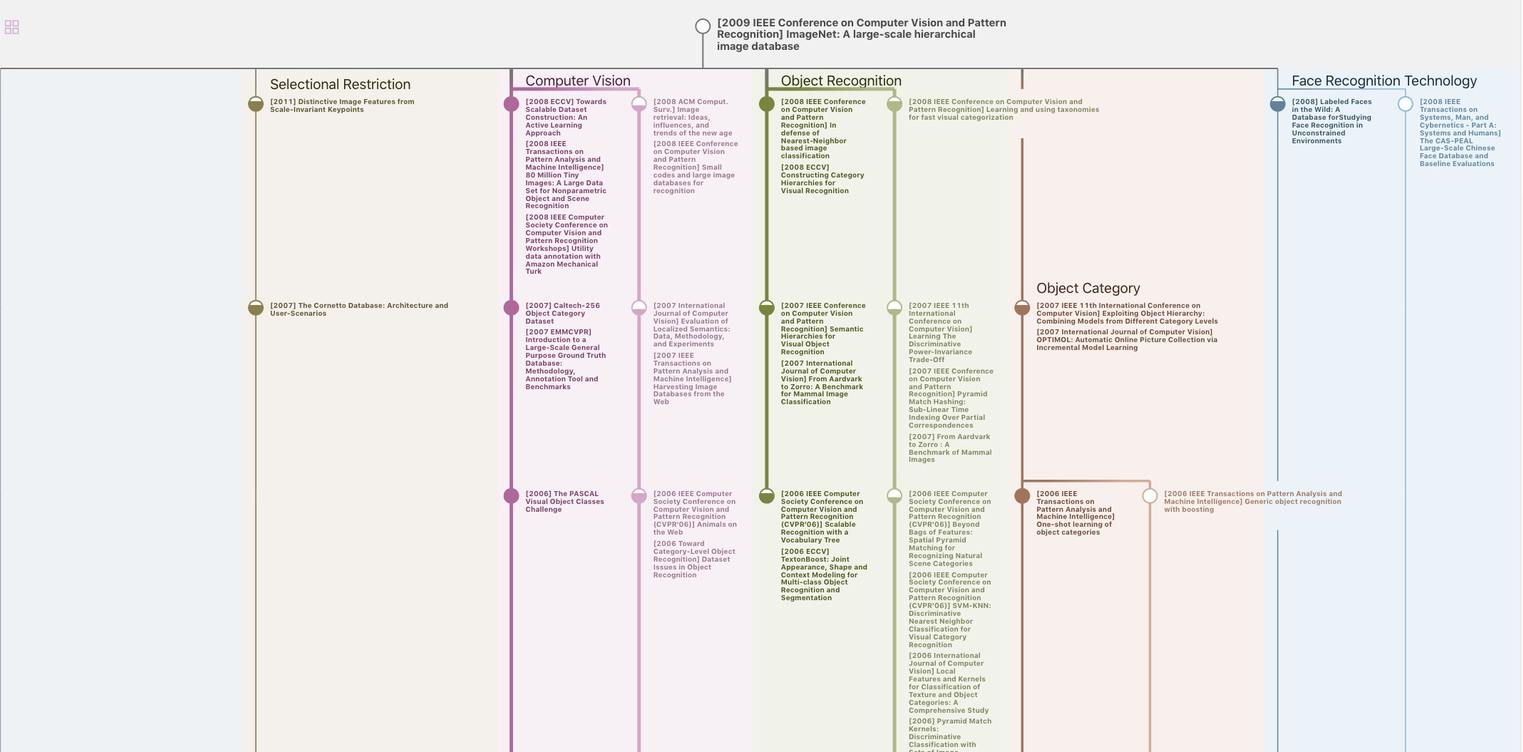
生成溯源树,研究论文发展脉络
Chat Paper
正在生成论文摘要