Urine trumps the protocol biopsy for subclinical rejection surveillance
Kidney international(2023)
摘要
Patient centricity is the focus of health care in the 21st century. We aim to create a healthier world where patients receive and providers deliver precise, tailored, cost-effective care. Nowhere is this more important than in the transplant field, where a functioning kidney transplant is the most desired and optimal treatment for end-stage kidney disease. Yet, limited organ availability and subclinical and incompletely diagnosed alloimmune injury result in persisting inflammation, scarring, and loss of functioning kidney tissue, leading to reduced graft survival. Today, >90,000 patients are on the kidney transplant waiting list in the United States, and yet ≈22,000—<25% of eligible patients—are anticipated to receive an organ transplant each year, with the remainder facing reduced mortality and increased morbidity on dialysis. As such, our shared priority across the nephrology community is to change this inexorable trajectory of untimely graft loss. As a scientific community, we all recognize that a key action item is to better identify evolving allograft pathology at the earliest opportunity so that prompt treatment can be instituted to reverse tissue injury and thus extend graft survival. Addressing this is anticipated to directly and positively impact organ availability for more eligible candidates on the wait lists. The diagnostic limitations of the allograft biopsy (limited and variable sampling, sampling confounders, such as scar, and reader variability resulting in diagnostic instability) and limited repeatability of an invasive procedure dictate the development of robust, noninvasive, and unbiased biomarkers correlative with rejection biology, yet independent of the variations of tissue sampling and interpretation. Developing biomarkers that can provide an unbiased measure of rejection, before functional decline, will also drive the mandate of personalized and precision immunosuppression, delivering transplant care as a holistic patient-centric approach and extrapolating to improved patient quality of life. The following sections discuss the current standard of care and a summary of why noninvasive biomarkers are poised to detect subclinical rejection and replace the protocol biopsy. At present, rejection following transplantation is primarily evaluated by tracking a trend in the serum creatinine level, reflexing to an indication core kidney biopsy with a threshold serum creatinine increase (usually >20% above "baseline”). Although each of these tools has been used effectively, they have their limitations. The serum creatinine level has been used for decades to assess kidney function and graft status post-transplant but is a nonspecific and nonsensitive biomarker. Increased creatinine level is nonspecific and may be a result of host patient factors unrelated to kidney function or performance, and may contribute to advancing patients to costly, unnecessary, and invasive procedures during a rejection rule-out process. In addition, as a nonsensitive marker, serum creatinine level has limited utility in providing advance indication of evolving rejection and may miss rejection altogether (“subclinical rejection”), although advanced modeling might offer advantage over snapshot evaluations. Thus, serum creatinine level, a marker of kidney function, may provide a false sense of security and mask early injury (rejection). Proteinuria is even less sensitive and specific for ongoing transplant rejection than serum creatinine level. The needle core biopsy is currently the reference standard to obtain diagnostic and prognostic information on kidney allograft dysfunction. The Banff classification schema1Loupy A. Haas M. Roufosse C. et al.The Banff 2019 Kidney Meeting Report (I): updates on and clarification of criteria for T cell- and antibody-mediated rejection.Am J Transplant. 2020; 20: 2318-2331Abstract Full Text Full Text PDF PubMed Scopus (323) Google Scholar offers a descriptive and semiquantitative classification of inflammation and rejection (T cell–mediated rejection [TCMR] and antibody-mediated rejection phenotypes), as well as the state of the tissue as it relates to disease activity, chronicity, tubular injury, infection, and recurrent disease, with the chronicity of injury providing some prognostic value for graft outcomes. Innovative methods are under way to standardize histologic reads and interpretation, to obviate interpathologist manual read subjective variations, with the greatest disagreements in final diagnostic classification relating to the borderline or incomplete histologic phenotypes. The protocol or surveillance biopsy is selected as an allograft monitoring tool, to be done at some institutionally defined time point in the early months or years post-transplant, to detect subclinical rejection, loosely defined as rejection that is undetected by assessment of serum creatinine level or proteinuria. Histologic injury in evolving rejection can be patchy and missed by a needle biopsy core that grabs ≈1 to 2 mm from a kidney that is ≈900 to 1000 mm in length. Today, the utility of the protocol biopsy is up for debate, as procedural risk, cost, and patient inconvenience may outweigh the benefit of diagnosis of subclinical rejection,2Puttarajappa C.M. Mehta R.B. Roberts M.S. et al.Economic analysis of screening for subclinical rejection in kidney transplantation using protocol biopsies and noninvasive biomarkers.Am J Transplant. 2021; 21: 186-197Abstract Full Text Full Text PDF PubMed Scopus (21) Google Scholar reasons why protocol biopsies can also not be done frequently. Although subclinical rejection was seen as snapshot in 20% to 40% of protocol biopsies performed in the first months post-transplantation, more recent studies show a lower incidence of histologic rejection in protocol biopsies.3Rush D.N. Gibson I.W. Subclinical inflammation in renal transplantation.Transplantation. 2019; 103: e139-e145Crossref PubMed Scopus (18) Google Scholar Nevertheless, the true incidence of alloimmune injury in the allograft is higher as molecular rejection preceeds histologic rejection, and approximately one-third of 1154 stable allografts with normal histology were shown to have molecular signals in the tissue identical to those seen with histologic Banff-graded rejection.4Rychkov D. Sur S. Sirota M. et al.Molecular diversity of clinically stable human kidney allografts.JAMA Netw Open. 2021; 4e2035048Crossref Scopus (7) Google Scholar Figure 1 outlines the dynamic state of acute (antibody-mediated) rejection that can have varied injury mechanisms and severity,5Loupy A. Hill G.S. Jordan S.C. The impact of donor-specific anti-HLA antibodies on late kidney allograft failure.Nat Rev Nephrol. 2012; 8: 348-357Crossref PubMed Scopus (288) Google Scholar tempo, and outcomes, and yet carries a common blanket terminology descriptor of acute rejection. The recent study by Rychkov et al. highlighted the unmet need to get to more granular dissection of clinical, histologic, and molecular rejection and the evolution of 2 new categories: one of evolving rejection/molecular rejection without histologic injury, and perhaps one of resolving or mild histologic rejection without molecular signals of rejection (Figure 2).4Rychkov D. Sur S. Sirota M. et al.Molecular diversity of clinically stable human kidney allografts.JAMA Netw Open. 2021; 4e2035048Crossref Scopus (7) Google Scholar Finally, rejection is not a 1-time event, but can persist or wax and wane over time,5Loupy A. Hill G.S. Jordan S.C. The impact of donor-specific anti-HLA antibodies on late kidney allograft failure.Nat Rev Nephrol. 2012; 8: 348-357Crossref PubMed Scopus (288) Google Scholar as was recently shown for both TCMR and antibody-mediated rejection.6Rampersad C. Balshaw R. Gibson I.W. et al.The negative impact of T cell-mediated rejection on renal allograft survival in the modern era.Am J Transplant. 2022; 22: 761-771Abstract Full Text Full Text PDF PubMed Scopus (23) Google Scholar,7Coemans M. Senev A. Van Loon E. et al.The evolution of histological changes suggestive of antibody-mediated injury, in the presence and absence of donor-specific anti-HLA antibodies.Transpl Int. 2021; 34: 1824-1836Crossref PubMed Scopus (7) Google ScholarFigure 2Noninvasive monitoring by blood or urine biomarkers could distinguish a histologic and molecular stable allograft (hSTA/mSTA), allowing for continued monitoring, avoidance of any protocol biopsy, and the clinical option of immunosuppression minimization. The stage shift from the stable allograft to molecular rejection (mAR) can be detected by increasing biomarker scores in the intermediate range. The progression of immunologic injury in the renal allograft, with both molecular and histologic evidence of rejection, could be accompanied by high urine biomarker QSant scores, with the height of the biomarker score strongly correlated with the severity of graft rejection. Adapted from Rychkov D, Sur S, Sirota M, et al. Molecular diversity of clinically stable human kidney allografts. JAMA Netw Open 2021;4:e20350484Rychkov D. Sur S. Sirota M. et al.Molecular diversity of clinically stable human kidney allografts.JAMA Netw Open. 2021; 4e2035048Crossref Scopus (7) Google Scholar under the terms of the CC-BY license. © 2021 Rychkov D et al. JAMA Network Open.View Large Image Figure ViewerDownload Hi-res image Download (PPT) For all these reasons, the protocol biopsy is a suboptimal method as a rejection monitoring tool. If all episodes of subclinical rejection are to be detected, more serial screening for rejection is needed, above and beyond the monitoring of serum creatinine level/proteinuria and the performing of protocol biopsies (Figure 1). Although commercially available blood-based biomarkers, such as donor-derived cell-free DNA (dd-cfDNA) and mRNA expression assays, such as kSORT and TruGraf, are being used to assess graft rejection,8Oellerich M. Sherwood K. Keown P. et al.Liquid biopsies: donor-derived cell-free DNA for the detection of kidney allograft injury.Nat Rev Nephrol. 2021; 17: 591-603Crossref PubMed Scopus (45) Google Scholar, 9Filippone E.J. Farber J.L. The monitoring of donor-derived cell-free DNA in kidney transplantation.Transplantation. 2021; 105: 509-516Crossref PubMed Scopus (27) Google Scholar, 10Huang E. Mengel M. Clahsen–van Groningen M.C. et al.Diagnostic potential of minimally invasive biomarkers: a biopsy-centered viewpoint from the Banff Minimally Invasive Diagnostics Working Group.Transplantation. 2023; 107: 45-52Crossref Scopus (1) Google Scholar, 11Naesens M. Friedewald J. Mas V. et al.A practical guide to the clinical implementation of biomarkers for subclinical rejection following kidney transplantation.Transplantation. 2020; 104: 700-707Crossref PubMed Scopus (14) Google Scholar these assays in independent validation studies have been shown to have variable levels of specificity, negative predictive value, sensitivity, and positive predictive value, and difficulty in assessing all grades and subtypes of rejection, with limited detection of TCMR by some blood dd-cfDNA assays. Blood-based biomarkers reflect activation of alterations of recipients’ circulating immune cells on interaction with donor antigens or injury molecules like dd-cfDNA originating from the allograft itself, with the latter likely being inherently less specific for the mechanism of kidney injury.8Oellerich M. Sherwood K. Keown P. et al.Liquid biopsies: donor-derived cell-free DNA for the detection of kidney allograft injury.Nat Rev Nephrol. 2021; 17: 591-603Crossref PubMed Scopus (45) Google Scholar, 9Filippone E.J. Farber J.L. The monitoring of donor-derived cell-free DNA in kidney transplantation.Transplantation. 2021; 105: 509-516Crossref PubMed Scopus (27) Google Scholar, 10Huang E. Mengel M. Clahsen–van Groningen M.C. et al.Diagnostic potential of minimally invasive biomarkers: a biopsy-centered viewpoint from the Banff Minimally Invasive Diagnostics Working Group.Transplantation. 2023; 107: 45-52Crossref Scopus (1) Google Scholar The gene expression signals detectable in blood might be more specific and reflect those in the transplant kidneys (e.g., for TCMR and antibody-mediated rejection), but signal-to-noise ratio of rejection is lower in blood than in the biopsies,12Van Loon E. Lamarthee B. Barba T. et al.Circulating donor-specific anti-HLA antibodies associate with immune activation independent of kidney transplant histopathological findings.Front Immunol. 2022; 13818569Crossref Scopus (10) Google Scholar resulting in a limited assay dynamic range, potentially capping the sensitivity performance of blood as biofluid for rejection diagnosis. Recipient age, variations in red and white cell indexes, and infection types are some of the clinical indexes that can also make interpretation of transcriptional analyses challenging. Finally, achieving external validity of complex multifactorial tests can be challenging in different ethnic populations, which can lead to low global acceptance of tests. Larger studies with more definite answers on the diagnostic performances of blood biomarkers are needed. At the moment, it is suggested that their clinical utility primarily lies in the evaluation of low risk (i.e., rule-out rejection), although it can be argued that higher negative predictive values of these assays cannot be leveraged in isolation, without considering a high positive predictive assay performance, for avoiding protocol biopsies. It should, however, also be recognized that higher negative predictive values are primarily explained by low disease prevalence.13Anglicheau D. Naesens M. Essig M. et al.Establishing biomarkers in transplant medicine: a critical review of current approaches.Transplantation. 2016; 100: 2024-2038Crossref PubMed Scopus (63) Google Scholar Urine has been a difficult biofluid to harness for generation of biomarkers for clinical translation, despite being an informative biofluid that provides a great window into kidney pathobiology for academic study, where the required personnel and infrastructure exist on site for immediate processing and analysis after a voided collection. Urine requires careful collection, transport, and rapid processing after voiding because the invariant contamination with pathogens and enzymes rapidly degrades its biological content. We have previously published reviews of the current state of play of urine biomarkers for evaluation of graft rejection, the type of biomarker, the stages of development, any clinical validation, and performance criteria. At the transcriptional level, urine RNA, microRNA, and exosomal RNAs provide signals correlative with contemporaneous biopsy rejection histology in the transplanted organ. Without a strict protocol for sample processing, RNA can degrade rapidly and make many samples unusable; this can occur even with the most stringent of studies using urine processing within 1 hour of voiding, as seen in this study of urine samples, where ≈30% of samples had degraded RNA and could not be analyzed further. Also, urine proteomic studies have shown promising fingerprints for graft rejection and polyomavirus-associated nephropathy, and chemokines, such as CXC chemokine ligands 9 and 10, have been widely validated to reflect graft inflammation in rejection.13Anglicheau D. Naesens M. Essig M. et al.Establishing biomarkers in transplant medicine: a critical review of current approaches.Transplantation. 2016; 100: 2024-2038Crossref PubMed Scopus (63) Google Scholar,14Farkona S, Pastrello C, Konvalinka A. Proteomics: its promise and pitfalls in shaping precision medicine in solid organ transplantation. Transplantation. Published online February 20, 2023. https://doi.org/10.1097/TP.0000000000004539Google Scholar Sample processing constraints are less rigorous for urinary proteomics when compared with urine RNA studies, and costs are substantially lower, but urine samples have always required rapid processing soon after a voided collection and storage at cold temperatures, to minimize analyte degradation. Recent developments in urine-stabilizing preservatives have now extended the window of urine processing to 3 days at room temperature after a voided collection.15Yang J.Y.C. Sarwal R.D. Sigdel T.K. et al.A urine score for noninvasive accurate diagnosis and prediction of kidney transplant rejection.Sci Transl Med. 2020; 12eaba2501Crossref Scopus (45) Google Scholar Urine analysis of cell-free DNA in urine shows promise in both rejection and infection, and can now be measured without the requirement of sequencing technologies. A summary of recent reports on these markers in urine and their published performance characteristics for renal allograft rejection diagnostics is presented in Table 1.8Oellerich M. Sherwood K. Keown P. et al.Liquid biopsies: donor-derived cell-free DNA for the detection of kidney allograft injury.Nat Rev Nephrol. 2021; 17: 591-603Crossref PubMed Scopus (45) Google Scholar, 9Filippone E.J. Farber J.L. The monitoring of donor-derived cell-free DNA in kidney transplantation.Transplantation. 2021; 105: 509-516Crossref PubMed Scopus (27) Google Scholar, 10Huang E. Mengel M. Clahsen–van Groningen M.C. et al.Diagnostic potential of minimally invasive biomarkers: a biopsy-centered viewpoint from the Banff Minimally Invasive Diagnostics Working Group.Transplantation. 2023; 107: 45-52Crossref Scopus (1) Google Scholar, 11Naesens M. Friedewald J. Mas V. et al.A practical guide to the clinical implementation of biomarkers for subclinical rejection following kidney transplantation.Transplantation. 2020; 104: 700-707Crossref PubMed Scopus (14) Google Scholar,S1–S19Table 1Overview of selected urinary biomarker studies for diagnosis of kidney transplant injuryReferenceAnalyte assessed in urineCommentsBiomarkersTest designSensitivity, %Specificity, %AUCSuthanthiran et al. (2013)S1https://doi.org/10.1056/NEJMoa1215555Urine pelletUrine mRNA measured by PCRUrine centrifugation with 1 h ideally and processing within 4 h of collectionRNA subject to degradationCD3ε mRNA + IP-10 mRNA + 18S rRNATCMR79780.85Nissaisorakarn et al. (2018)S2https://doi.org/10.1016/j.humimm.2018.01.006CD3ε + CD105 + CD14 + CD46 + 18S rRNATCMR vs. AMRNANA0.81Sigdel et al. (2019)S3https://doi.org/10.1371/journal.pone.0220052Urine common response module (BASP1, CD6, CXCL10, CXCL9, INPP5D, ISG20, LCK, NKG7, PSMB9, RUNX3, TAP1)AR vs. BKVN + bAR + control76.9297.780.97Kaminski et al. (2020)S4https://doi.org/10.1038/s41551-020-0546-5CXCL9 mRNAAR vs. control93760.91Lorenzen et al. (2011)S5https://doi.org/10.1111/j.1600-6143.2011.03679.xmiR-210AR vs. control74520.70Millán et al. (2017)S6https://doi.org/10.1111/bcp.13399CXCL10 mRNAAR vs. non-AR84800.87Nissaisorakarn et al. (2018)S2https://doi.org/10.1016/j.humimm.2018.01.006Urine supernatantUrine metabolites measured by GC-MSUrine centrifugation with 1 h ideally and processing within 4 h of collectionMetabolites more stable than RNA3-Sialyllactose, xanthosine + quinolinate + X-16397 + CD3ε mRNA + IP-10 mRNATCMR vs. non-TCMR90840.93Sigdel et al. (2020)S7https://doi.org/10.3390/jcm9082341Glycine, adipic acid, glutaric acid, N-methylalanine, inulobiose, threose, sulfuric acid, taurine, asparagine, 5-aminovaleric acid, myoinositolAR vs. control92.996.30.98Arabinose, 2-hydroxy-2-methylbutanoic acid, octadecanol, and phosphateBKVN vs. non-BKVN88.9940.94Blydt-Hansen et al. (2014)S8 https://doi.org/10.1111/ajt.12837Proline, kynurenine, sarcosine, methionine sulfoxide, threonine, glutamine, phenylalanine, alanineTCMR vs. non-TCMR83830.88Park et al. (2017)S9https://doi.org/10.1111/ajt.14600Urine supernatantUrine proteins measured by ELISA-based assaysCD3+ extracellular vesicles (iKEA)TCMR vs. non-TCMR92.887.50.84Sigdel et al. (2010)S10; Sigdel et al. (2016)S11https://doi.org/10.1002/prca.200900124https://doi.org/10.1016/j.kint.2015.12.049Tamm-Horsfall protein (UMOD), PEDF (SERPINF1), CD44 + 12 peptide panelAR vs. non-AR, BKVN, CAN, control63.61000.85Lim et al. (2018)S12https://doi.org/10.1371/journal.pone.0204204Tetraspanin-1 (TSPAN1) and hemopexin (HPX)TCMR vs. control64720.74Kanzelmeyer et al. (2019)S13 https://doi.org/10.1111/tri.1336379 peptide panel + CKD273cAMR88920.92Mertens et al. (2020)S14https://doi.org/10.1016/j.ekir.2020.06.018A1BG, AFM, APOA1, APOA4, IGHA1, IGHA4, LRG1, SERPINA1, SERPINC1, TFAMR95760.88Hirt-Minkowski et al. (2012)S15https://doi.org/10.1111/j.1600-6143.2012.03999.xCXCL10 proteinAR61720.69Raza et al. (2017)S16https://doi.org/10.1007/s00011-017-1025-7CXCL10 proteinAR vs. non-AR72710.74Jackson et al. (2011)S17https://doi.org/10.1111/j.1600-6143.2011.03680.xCXCL9 proteinAR or BKV vs. CAN8680NACXCL10 proteinAR or BKV vs. IFTA8076NARabant et al. (2015)S18https://doi.org/10.1681/ASN.2014080797CXCL10 proteinAR5983.372.6TCMR6079.372.6AMR60.383.374.7CXCL9 proteinAR58.484.571.6TCMR808785.7AMR55.284.569.4Yang et al. (2020)S19https://doi.org/10.3390/jcm9082341Urine supernatantMultiple analytes measured by ELISA and reverse ELISA, no amplification, no sequencingDelayed urine processing to 3 d after collection, stability in preservative at room temperature for 3 dcfDNA, m-cfDNA, CXCL10, creatinine, clusterin, total proteinAR vs. no-AR95.899.30.98A1BG, alpha-1-B glycoprotein; AFM, afamin; AMR, antibody-mediated rejection; APOA, apolipoprotein A; AR, (nonspecific) acute rejection; AUC, area under the curve; bAR, borderline acute rejection; BASP, brain abundant, membrane attached signalprotein 1; BKV, BK polyomavirus; BKVN, BK polyomavirus nephropathy; cAMR, chronic antibody-mediated rejection; CAN, chronic allograft nephropathy; cfDNA, cell-free DNA; CXCL, CXC chemokine ligand; ELISA, enzyme-linked immunosorbent assay; GC-MS, gas chromatography–mass spectrometry; IGHA, Immunoglobulin heavy constant alpha; INPP5D, inositol polyphosphate-5-phosphatase; IP-10, CXCL10; ISG20, interferon stimulated exonuclease gene 20kDa; LCK, LCK proto-oncogene, Src family tyrosine kinase; LRG, leucine-rich alpha-2-glycoprotein 1; m-cfDNA, methylated cell-free DNA; NKG7, natural killer cell granule protein 7; PCR, polymerase chain reaction; PSMB9, proteasome subunit, beta type, 9; SERPIN, serpin mRNA binding pseudogene; TAP1, BC transporter, MHC 1; TCMR, T cell–mediated rejection; UMOD, uromodulin.For recent reviews of blood biomarkers, we refer to Oellerich et al.,8Oellerich M. Sherwood K. Keown P. et al.Liquid biopsies: donor-derived cell-free DNA for the detection of kidney allograft injury.Nat Rev Nephrol. 2021; 17: 591-603Crossref PubMed Scopus (45) Google Scholar Filippone and Farber,9Filippone E.J. Farber J.L. The monitoring of donor-derived cell-free DNA in kidney transplantation.Transplantation. 2021; 105: 509-516Crossref PubMed Scopus (27) Google Scholar Huang et al.,10Huang E. Mengel M. Clahsen–van Groningen M.C. et al.Diagnostic potential of minimally invasive biomarkers: a biopsy-centered viewpoint from the Banff Minimally Invasive Diagnostics Working Group.Transplantation. 2023; 107: 45-52Crossref Scopus (1) Google Scholar and Naesens et al.11Naesens M. Friedewald J. Mas V. et al.A practical guide to the clinical implementation of biomarkers for subclinical rejection following kidney transplantation.Transplantation. 2020; 104: 700-707Crossref PubMed Scopus (14) Google Scholar In this table, DOI numbers are provided; full references are available in the Supplementary References (S suffixes). Open table in a new tab A1BG, alpha-1-B glycoprotein; AFM, afamin; AMR, antibody-mediated rejection; APOA, apolipoprotein A; AR, (nonspecific) acute rejection; AUC, area under the curve; bAR, borderline acute rejection; BASP, brain abundant, membrane attached signalprotein 1; BKV, BK polyomavirus; BKVN, BK polyomavirus nephropathy; cAMR, chronic antibody-mediated rejection; CAN, chronic allograft nephropathy; cfDNA, cell-free DNA; CXCL, CXC chemokine ligand; ELISA, enzyme-linked immunosorbent assay; GC-MS, gas chromatography–mass spectrometry; IGHA, Immunoglobulin heavy constant alpha; INPP5D, inositol polyphosphate-5-phosphatase; IP-10, CXCL10; ISG20, interferon stimulated exonuclease gene 20kDa; LCK, LCK proto-oncogene, Src family tyrosine kinase; LRG, leucine-rich alpha-2-glycoprotein 1; m-cfDNA, methylated cell-free DNA; NKG7, natural killer cell granule protein 7; PCR, polymerase chain reaction; PSMB9, proteasome subunit, beta type, 9; SERPIN, serpin mRNA binding pseudogene; TAP1, BC transporter, MHC 1; TCMR, T cell–mediated rejection; UMOD, uromodulin. For recent reviews of blood biomarkers, we refer to Oellerich et al.,8Oellerich M. Sherwood K. Keown P. et al.Liquid biopsies: donor-derived cell-free DNA for the detection of kidney allograft injury.Nat Rev Nephrol. 2021; 17: 591-603Crossref PubMed Scopus (45) Google Scholar Filippone and Farber,9Filippone E.J. Farber J.L. The monitoring of donor-derived cell-free DNA in kidney transplantation.Transplantation. 2021; 105: 509-516Crossref PubMed Scopus (27) Google Scholar Huang et al.,10Huang E. Mengel M. Clahsen–van Groningen M.C. et al.Diagnostic potential of minimally invasive biomarkers: a biopsy-centered viewpoint from the Banff Minimally Invasive Diagnostics Working Group.Transplantation. 2023; 107: 45-52Crossref Scopus (1) Google Scholar and Naesens et al.11Naesens M. Friedewald J. Mas V. et al.A practical guide to the clinical implementation of biomarkers for subclinical rejection following kidney transplantation.Transplantation. 2020; 104: 700-707Crossref PubMed Scopus (14) Google Scholar In this table, DOI numbers are provided; full references are available in the Supplementary References (S suffixes). Despite the promises of all the minimally invasive and noninvasive biomarkers for graft injury processes individually, single analytes, either in blood or urine, do not capture the entire complexity of injury and allograft rejection, as the underlying “health” of the tissue will also determine the extent of the wounding response in rejection, and as diseases are not single states but evolve over time from active to more chronic indolent phenotypes. Several multimodal approaches are being developed and are showing improved accuracy for detection of graft rejection. For instance, the combination of dd-cfDNA testing and donor-specific antibody testing is promising for antibody-mediated rejection diagnosis,16Halloran P.F. Madill-Thomsen K.S. Pon S. et al.Molecular diagnosis of ABMR with or without donor-specific antibody in kidney transplant biopsies: differences in timing and intensity but similar mechanisms and outcomes.Am J Transplant. 2022; 22: 1976-1991Abstract Full Text Full Text PDF PubMed Scopus (16) Google Scholar as well as combining dd-cfDNA testing with a blood mRNA signature for immunologic quiescence, which improved diagnostic performance in comparison to either test separately.17Akalin E. Weir M.R. Bunnapradist S. et al.Clinical validation of an immune quiescence gene expression signature in kidney transplantation.Kidney360. 2021; 2: 1998-2009Crossref PubMed Scopus (4) Google Scholar, 18Al Jurdi A. Gassen R.B. Borges T.J. et al.Non-invasive monitoring for rejection in kidney transplant recipients after SARS-CoV-2 mRNA vaccination.Front Immunol. 2022; 13838985Crossref Scopus (12) Google Scholar, 19Park S. Guo K. Heilman R.L. et al.Combining blood gene expression and cellfree DNA to diagnose subclinical rejection in kidney transplant recipients.Clin J Am Soc Nephrol. 2021; 16: 1539-1551Crossref PubMed Scopus (26) Google Scholar Also in urine, multimodal assessment of graft status, inclusive of the early detection of subclinical rejection, seems promising, where urine can be self-collected by a patient at home and mailed in for laboratory-based composite score of urinary cfDNA quantification with additional measurement of urinary CXC chemokine ligand 10, clusterin, total protein, and creatinine, as observed in the QSant urine test.15Yang J.Y.C. Sarwal R.D. Sigdel T.K. et al.A urine score for noninvasive accurate diagnosis and prediction of kidney transplant rejection.Sci Transl Med. 2020; 12eaba2501Crossref Scopus (45) Google Scholar Such minimally invasive and noninvasive tests can be used serially to predict the evolution of molecular rejection and track the resolution of rejection after immunosuppression intensification (Figure 1). A direct comparison between different tests, developed by different companies and measured on different body fluids and with sometimes different proposed clinical context of use, is complicated. Ideally, the clinical utility of these tests should be evaluated critically with the performance of head-to head studies conducted on different biofluids and assays on the same patient, at the same time point, and with biopsy-matched biosamples. To bring about the necessary change in patient care and the desired improvements in graft outcome, a clear unmet need exists for smart molecular tools that mirror and quantify graft injury, well before any functional decline. Today, minimally invasive and noninvasive monitoring of kidney transplant health/injury is possible in routine practice, and feasible to be integrated with patient care. Further clinical validity and utility of these minimally invasive and noninvasive assays will be a continued requirement by the transplant community. These efforts will require well-designed multicentric international studies, ideally also on decentralized platforms. The high costs of clinical trials and manpower shortages in transplant centers create execution and time barriers to the transplant community to rapidly achieve the goal of having these screening tests validated in unselected prospective multicenter cohorts, in patients with varying sensitization risks and demographics, and in prospective randomized controlled trials. Nevertheless, these types of efforts are under way by the transplant community to conduct such well-designed studies, and as of writing this review, there are 2115 clinical trials in kidney transplantation (www.clinicaltrials.gov), 248 of which use the study of biomarkers and 75 of which use the study of urine biomarkers for studying kidney transplant outcomes. The choice of primary end points and power calculations for such trials is crucial, as exemplified by a recent randomized trial investigating urinary CXC chemokine ligand 10 monitoring.20Hirt-Minkowski P, Handschin J, Stampf S, et al. Randomized trial to assess the clinical utility of renal allograft monitoring by urine CXCL10 chemokine. J Am Soc Nephrol. Published online May 25, 2023. https://doi.org/10.1681/ASN.0000000000000160Google Scholar This study indicates that monitoring subclinical disease not necessarily translates in hard end points, as measured using a complex composite end point (death-censored graft loss, clinical rejection between month 1 and 1 year, acute rejection in 1-year surveillance biopsy, chronic active TCMR in 1-year surveillance biopsy, development of de novo donor-specific human leukocyte antigen antibodies, or estimated glomerular filtration rate <25 ml/min). Next to power issues when using the Banff 2019 classification instead of earlier iterations, the study did not evaluate whether the increased detection and sometimes treatment of subclinical rejection episodes would ultimately result in benefits on the longer-term. To address this, formal testing would be required, along with the systematic implementation of effective treatment for subclinical rejection. Furthermore, clinical utility of a noninvasive biomarker extends beyond enhancing outcomes; it also encompasses the avoidance of unnecessary biopsies. Although such clinical value for avoiding uninformative biopsies has been suggested in retrospective studies on urinary chemokines,21Tinel C. Devresse A. Vermorel A. et al.Development and validation of an optimized integrative model using urinary chemokines for noninvasive diagnosis of acute allograft rejection.Am J Transplant. 2020; 20: 3462-3476Abstract Full Text Full Text PDF PubMed Scopus (24) Google Scholar formal testing of this in randomized studies is not yet available. An ideal future scenario for kidney transplant monitoring would be a noninvasive home-based test for immediate readout for subclinical rejection, rather than the current time-delayed readout for all currently available minimally or noninvasive tests, all of which require the biosample to be shipped to a remote laboratory for analysis. In home, immediate evaluation of graft health status, similar to current blood glucose monitoring methods, is a goal for the transplant community to aspire to, as then, and only then, will we bring in proactive monitoring for subclinical rejection, followed by the critical and actionable titration of immunosuppression to immune risk threshold. Recognizing the fluid, real-time evolution of the alloimmune response as the driver of subclinical rejection, our efforts need to double down to find this holy grail of noninvasive allograft monitoring, to change the trajectory of chronic graft injury and graft and patient survival. MMS is the founder of QSant and NephroSant Inc., which has the commercial license for QSant from the Regents, University of California. MMS has held or holds consulting or research engagements with Novartis, Genentech, Natera, Immucor, Bristol Meyers Squibb, Jazz Pharma, Graticule, Astellas, the Chan-Zuckerberg Initiative, Cystinosis Foundation Ireland, and the Health Research Board, Ireland; and serves on the US Food and Drug Administration Science Board. MN is the founder of an mRNA biomarker panel in peripheral blood for noninvasive diagnosis of antibody-mediated rejection, licensed by KU Leuven to CareDx. MN holds consulting or research engagements with Hansa, Agomab Therapeutics, Argenx, CareDx, and Aiyosyn; and is advisory member of the CPATH-Transplant Therapeutics Consortium. MN is senior clinical investigator at the Research Foundation–Flanders (Fonds Wetenschappelijk Onderzoek [FWO]) under FWO grant 1844019N. The authors express their deepest thanks to Tara Sigdel for help with manuscript preparation and collation of references. Download .docx (.01 MB) Help with docx files Supplementary File (Word) Supplementary References. “One if by land, and two if by sea,” but what is the signal for rejection?Kidney InternationalVol. 104Issue 3PreviewFor many decades, protocol biopsies of the kidney allograft have been the diagnostic reference standards for acute and chronic changes of the allograft, with or without evidence of clinical kidney dysfunction (such as an increase in serum creatinine or presence of proteinuria). Protocol biopsies have the potential to identify acute and detrimental events early, such as subclinical rejection, and appropriate interventions can be implemented before significant graft dysfunction and may improve longer-term outcomes. Full-Text PDF
更多查看译文
关键词
subclinical rejection surveillance,protocol biopsy,urine
AI 理解论文
溯源树
样例
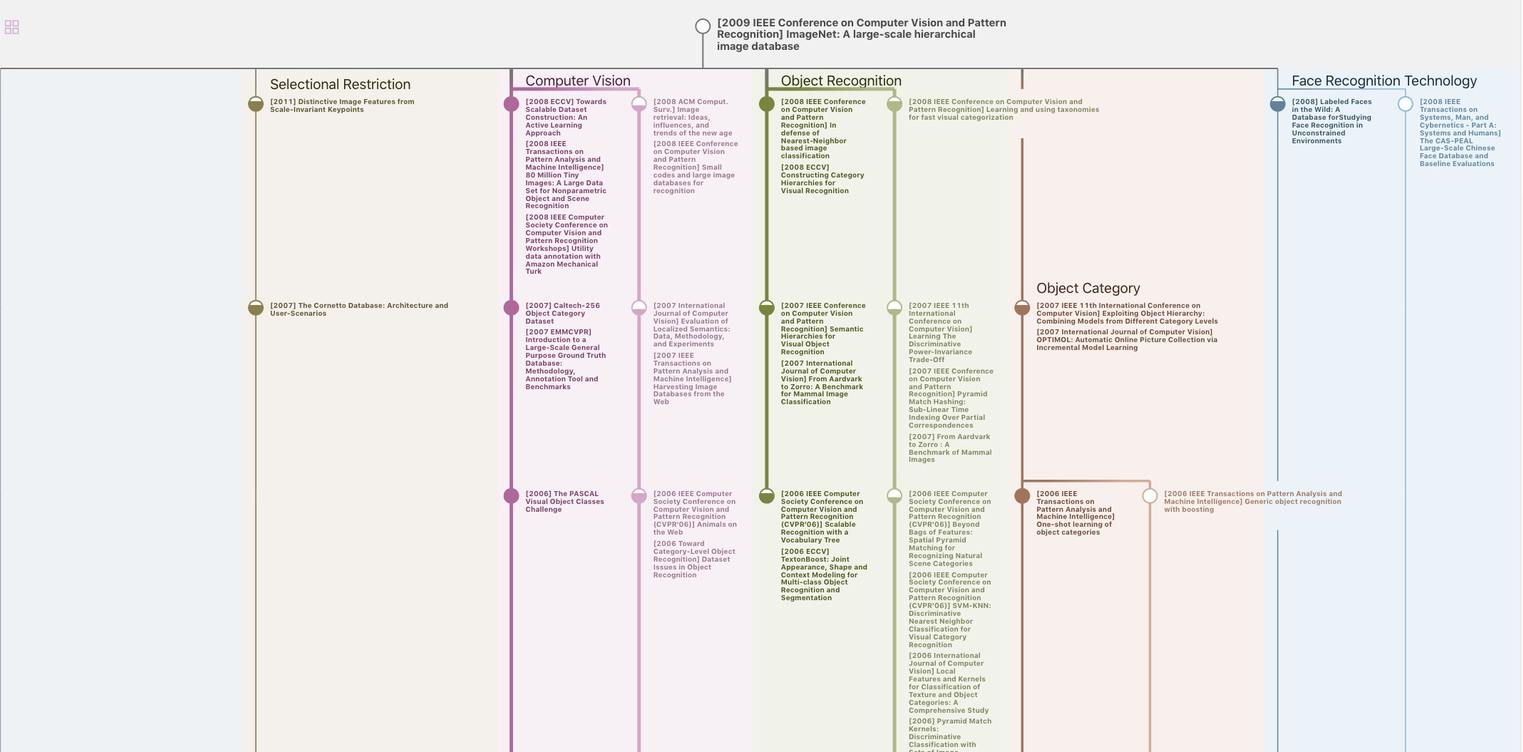
生成溯源树,研究论文发展脉络
Chat Paper
正在生成论文摘要