Biotechnology for a sustainable future: biomass and beyond
Trends in Biotechnology(2022)
Abstract
Biomass is the most abundant renewable energy source; its energy is stored in chemical bonds during photosynthetic CO2 reduction [1.Ragauskas A.J. et al.Lignin valorization: improving lignin processing in the biorefinery.Science. 2014; 344: 1246843Crossref PubMed Scopus (2365) Google Scholar]. Lignocellulosic biomass is also the largest source of renewable polymers [2.Zhang W. et al.Design of biomass-based renewable materials for environmental remediation.Trends Biotechnol. 2022; 40: 1519-1534Abstract Full Text Full Text PDF Scopus (2) Google Scholar]. Innovations in using this abundant natural resource have profoundly impacted the advancement of human civilization. Two million years ago, humans first learned to set fire with biomass and used it as an energy source. With the advancement of human society, lignocellulosic biomass has been widely used for construction, furniture, animal feed, and energy. About 2000 years ago, paper was first manufactured from biomass: early papermaking was a story of how to best obtain and use biomass fibers [3.Biermann C.J. Handbook of Pulping and Papermaking. Elsevier, 1996Google Scholar]. Modern pulping technologies were developed about 200 years ago, when we began to think about biomass as three major cell wall components: cellulose, hemicellulose, and lignin [3.Biermann C.J. Handbook of Pulping and Papermaking. Elsevier, 1996Google Scholar]. The essence of the paper and pulping industry is to produce high-quality cellulose pulp, while removing lignin either as waste or, hopefully, as a by-product. Compared with glucose-based cellulose, lignin is the most abundant aromatic polymer, with a higher carbon and energy content [1.Ragauskas A.J. et al.Lignin valorization: improving lignin processing in the biorefinery.Science. 2014; 344: 1246843Crossref PubMed Scopus (2365) Google Scholar,2.Zhang W. et al.Design of biomass-based renewable materials for environmental remediation.Trends Biotechnol. 2022; 40: 1519-1534Abstract Full Text Full Text PDF Scopus (2) Google Scholar]. Despite its unique structure, though, only 2% of lignin from the paper and pulping industry is used commercially as valorized products. Furthermore, the lignin in black liquor also imposes environmental hazards [2.Zhang W. et al.Design of biomass-based renewable materials for environmental remediation.Trends Biotechnol. 2022; 40: 1519-1534Abstract Full Text Full Text PDF Scopus (2) Google Scholar]. In more recent years, lignocellulosic biomass was also proposed to produce cellulosic ethanol, with the potential to replace over 30% of transportation fuels. However, the cellulosic biorefinery has never taken off commercially. The rise of the electric vehicle (EV) has further questioned whether producing ethanol as fuel from cellulose has a future. In fact, the modern paper and pulping industry and lignocellulosic biorefineries face the same challenge of how to valorize all biomass components toward valuable products [1.Ragauskas A.J. et al.Lignin valorization: improving lignin processing in the biorefinery.Science. 2014; 344: 1246843Crossref PubMed Scopus (2365) Google Scholar,4.Liu Z.-H. et al.Creative biological lignin conversion routes toward lignin valorization.Trends Biotechnol. 2022; 40: 1550-1566Abstract Full Text Full Text PDF Scopus (1) Google Scholar]. Besides a good strategy to utilize cellulose, we also need to develop strategies to best utilize lignin and hemicellulose. This special issue aims to answer the question from a biotechnology perspective and further examine the options beyond lignocellulosic biomass. The historical perspectives and societal needs inform us of several key considerations for biomass utilization toward a sustainable future. First, lignocellulosic biorefinery needs to utilize all biomass components, including cellulose, hemicellulose, and lignin [4.Liu Z.-H. et al.Creative biological lignin conversion routes toward lignin valorization.Trends Biotechnol. 2022; 40: 1550-1566Abstract Full Text Full Text PDF Scopus (1) Google Scholar,5.Li F. et al.Microbial lignin valorization through depolymerization to aromatics conversion.Trends Biotechnol. 2022; 40: 1469-1487Abstract Full Text Full Text PDF Scopus (1) Google Scholar]. Liu and colleagues review lignin utilization in the context of lignocellulosic biorefinery settings [4.Liu Z.-H. et al.Creative biological lignin conversion routes toward lignin valorization.Trends Biotechnol. 2022; 40: 1550-1566Abstract Full Text Full Text PDF Scopus (1) Google Scholar]. Considering the utilization of all three cell wall components, there is a debate regarding ‘lignin-first’ or carbohydrate-focused. Recent studies have indicated it is possible to design a lignocellulosic biorefinery to synergistically improve the carbohydrate saccharification efficiency and lignin bioconversion [4.Liu Z.-H. et al.Creative biological lignin conversion routes toward lignin valorization.Trends Biotechnol. 2022; 40: 1550-1566Abstract Full Text Full Text PDF Scopus (1) Google Scholar,6.Liu Z.-H. et al.Transforming biorefinery designs with ‘plug-in processes of lignin’ to enable economic waste valorization.Nat. Commun. 2021; 12: 3912Crossref PubMed Scopus (37) Google Scholar]. Regardless of the priority, a lignocellulosic biorefinery needs to create both an efficiently fermentable sugar stream and lignin in a processible form [4.Liu Z.-H. et al.Creative biological lignin conversion routes toward lignin valorization.Trends Biotechnol. 2022; 40: 1550-1566Abstract Full Text Full Text PDF Scopus (1) Google Scholar,7.Chen X. et al.DMR (deacetylation and mechanical refining) processing of corn stover achieves high monomeric sugar concentrations (230 g L−1) during enzymatic hydrolysis and high ethanol concentrations (>10% v/v) during fermentation without hydrolysate purification or concentration.Energy Environ. Sci. 2016; 9: 1237-1245Google Scholar]. When the lignin is depolymerized, aromatic monomers can be converted by chemical catalysis into lignin fuel and other products [8.Wang H. et al.Production of jet fuel-range hydrocarbons from hydrodeoxygenation of lignin over super Lewis acid combined with metal catalysts.ChemSusChem. 2018; 11: 285-291Crossref PubMed Scopus (74) Google Scholar, 9.Liu W. et al.High-efficiency electrochemical hydrodeoxygenation of bio-phenols to hydrocarbon fuels by a superacid-noble metal particle dual-catalyst system.Energy Environ. Sci. 2020; 13: 917-927Crossref Google Scholar, 10.Liu W. et al.Ambient-pressure and low-temperature upgrading of lignin bio-oil to hydrocarbons using a hydrogen buffer catalytic system.Nat. Energy. 2020; 5: 759-767Crossref Scopus (38) Google Scholar]. However, the challenges of the catalysis route for lignin fuels lie in the heterogeneity of the monomers, the need of hydrogenation upgrade, difficult downstream purification, and high catalyst cost. Bioconversion can funnel various aromatics into value-added products [4.Liu Z.-H. et al.Creative biological lignin conversion routes toward lignin valorization.Trends Biotechnol. 2022; 40: 1550-1566Abstract Full Text Full Text PDF Scopus (1) Google Scholar,5.Li F. et al.Microbial lignin valorization through depolymerization to aromatics conversion.Trends Biotechnol. 2022; 40: 1469-1487Abstract Full Text Full Text PDF Scopus (1) Google Scholar,11.Borchert A.J. et al.Challenges and opportunities in biological funneling of heterogeneous and toxic substrates beyond lignin.Curr. Opin. Biotechnol. 2022; 73: 1-13Crossref PubMed Scopus (16) Google Scholar]. In this special issue, we have included two reviews to systemically discuss the depolymerization-bioconversion route for lignin valorization [4.Liu Z.-H. et al.Creative biological lignin conversion routes toward lignin valorization.Trends Biotechnol. 2022; 40: 1550-1566Abstract Full Text Full Text PDF Scopus (1) Google Scholar,5.Li F. et al.Microbial lignin valorization through depolymerization to aromatics conversion.Trends Biotechnol. 2022; 40: 1469-1487Abstract Full Text Full Text PDF Scopus (1) Google Scholar]. Liu and colleagues focus more on the biorefinery design and its integration with bioconversion [4.Liu Z.-H. et al.Creative biological lignin conversion routes toward lignin valorization.Trends Biotechnol. 2022; 40: 1550-1566Abstract Full Text Full Text PDF Scopus (1) Google Scholar], while Li and coworkers focus more on the lignin depolymerization mechanisms and design of microorganisms using synthetic biology to valorize depolymerized lignin to different products [5.Li F. et al.Microbial lignin valorization through depolymerization to aromatics conversion.Trends Biotechnol. 2022; 40: 1469-1487Abstract Full Text Full Text PDF Scopus (1) Google Scholar]. In addition to engineering single microorganisms for lignin conversion, synthetic microbiota can also be exploited to convert carbohydrates and lignin into diverse and valuable bioproducts [12.Moon T.S. SynMADE: synthetic microbiota across diverse ecosystems.Trends Biotechnol. 2022; 40: 1405-1414Abstract Full Text Full Text PDF Scopus (1) Google Scholar]. While lignin depolymerization and conversion could be a viable route, the value of lignin polymer could also be in the polymer itself. In other words, with proper chemical modification, lignin and cellulose polymers could be used to produce value-added products, as discussed by several reviews in this special issue [2.Zhang W. et al.Design of biomass-based renewable materials for environmental remediation.Trends Biotechnol. 2022; 40: 1519-1534Abstract Full Text Full Text PDF Scopus (2) Google Scholar,13.Jia R. et al.Renewable plant-derived lignin for electrochemical energy systems.Trends Biotechnol. 2022; 40: 1425-1438Abstract Full Text Full Text PDF Scopus (2) Google Scholar, 14.Li J. et al.Feedstock design for quality biomaterials.Trends Biotechnol. 2022; 40: 1535-1549Abstract Full Text Full Text PDF Scopus (1) Google Scholar, 15.Fu X. et al.Biorefining waste into nanobiotechnologies can revolutionize sustainable agriculture.Trends Biotechnol. 2022; 40: 1503-1518Abstract Full Text Full Text PDF Scopus (1) Google Scholar, 16.Adler A. et al.Lignin-first biorefining of Nordic poplar to produce cellulose fibers could displace cotton production on agricultural lands.Joule. 2022; 6: 1845-1858Abstract Full Text Full Text PDF Scopus (2) Google Scholar]. Jia and colleagues review how lignin could be used for electrochemical storage purposes [13.Jia R. et al.Renewable plant-derived lignin for electrochemical energy systems.Trends Biotechnol. 2022; 40: 1425-1438Abstract Full Text Full Text PDF Scopus (2) Google Scholar]. Zhang and coauthors review how cellulose and lignin could be employed to manufacture materials for environmental remediation [2.Zhang W. et al.Design of biomass-based renewable materials for environmental remediation.Trends Biotechnol. 2022; 40: 1519-1534Abstract Full Text Full Text PDF Scopus (2) Google Scholar]. Li and colleagues summarize lignin-based materials in their discussion of feedstock design for biomaterials [14.Li J. et al.Feedstock design for quality biomaterials.Trends Biotechnol. 2022; 40: 1535-1549Abstract Full Text Full Text PDF Scopus (1) Google Scholar]. Fu and coworkers review the use of biorefinery waste to derive nanomaterials for agricultural applications [15.Fu X. et al.Biorefining waste into nanobiotechnologies can revolutionize sustainable agriculture.Trends Biotechnol. 2022; 40: 1503-1518Abstract Full Text Full Text PDF Scopus (1) Google Scholar]. These reviews provide a comprehensive analysis of emerging frontiers in the complete utilization of lignocellulosic biomass. The reviews in this special issue discuss the production and applications of many of these products [2.Zhang W. et al.Design of biomass-based renewable materials for environmental remediation.Trends Biotechnol. 2022; 40: 1519-1534Abstract Full Text Full Text PDF Scopus (2) Google Scholar,4.Liu Z.-H. et al.Creative biological lignin conversion routes toward lignin valorization.Trends Biotechnol. 2022; 40: 1550-1566Abstract Full Text Full Text PDF Scopus (1) Google Scholar,5.Li F. et al.Microbial lignin valorization through depolymerization to aromatics conversion.Trends Biotechnol. 2022; 40: 1469-1487Abstract Full Text Full Text PDF Scopus (1) Google Scholar,13.Jia R. et al.Renewable plant-derived lignin for electrochemical energy systems.Trends Biotechnol. 2022; 40: 1425-1438Abstract Full Text Full Text PDF Scopus (2) Google Scholar, 14.Li J. et al.Feedstock design for quality biomaterials.Trends Biotechnol. 2022; 40: 1535-1549Abstract Full Text Full Text PDF Scopus (1) Google Scholar, 15.Fu X. et al.Biorefining waste into nanobiotechnologies can revolutionize sustainable agriculture.Trends Biotechnol. 2022; 40: 1503-1518Abstract Full Text Full Text PDF Scopus (1) Google Scholar]. The development of biomass-based materials can be integrated with biorefining, where pretreatment conditions can be optimized to produce lignin polymers for biomaterial applications [14.Li J. et al.Feedstock design for quality biomaterials.Trends Biotechnol. 2022; 40: 1535-1549Abstract Full Text Full Text PDF Scopus (1) Google Scholar]. In other processes, thermochemical conversion, the pulping process, and various fractionation technologies can be used [2.Zhang W. et al.Design of biomass-based renewable materials for environmental remediation.Trends Biotechnol. 2022; 40: 1519-1534Abstract Full Text Full Text PDF Scopus (2) Google Scholar,11.Borchert A.J. et al.Challenges and opportunities in biological funneling of heterogeneous and toxic substrates beyond lignin.Curr. Opin. Biotechnol. 2022; 73: 1-13Crossref PubMed Scopus (16) Google Scholar]. Overall, this special issue explains various strategies to process lignocellulosic biomass so that all components can be processed into products for broad applications. Beyond complete biomass utilization, it is also critical to consider the economics and sustainability of each of these applications and to assess their potential in the bioeconomy. Second, biomass utilization needs to consider value proposition, market penetration, and societal needs. From the fuel perspective, the growth of EVs will substantially reduce the consumption of light vehicle fuels. The priority for biofuels is gradually shifting toward aviation fuels and long-range vehicle fuels. Recent advancements have demonstrated the feasibility to convert sugar into jet fuel precursors like 2,3-BDO (butanediol), monoterpenes, and polycyclopropanated (POP) fuels at high titer and conversion rates [17.Yang S. et al.Metabolic engineering of Zymomonas mobilis for 2, 3-butanediol production from lignocellulosic biomass sugars.Biotechnol. Biofuels. 2016; 9: 189Crossref PubMed Scopus (73) Google Scholar,18.Cruz-Morales P. et al.Biosynthesis of polycyclopropanated high energy biofuels.Joule. 2022; 6: 1590-1160Abstract Full Text Full Text PDF Scopus (4) Google Scholar]. However, it remains challenging to derive highly fermentable sugar from biomass to feed into more diverse microbial strains for aviation fuel production. It is thus critical to optimize pretreatment conditions to generate a sugar stream that can enable a similar performance to that of pure sucrose or glucose [7.Chen X. et al.DMR (deacetylation and mechanical refining) processing of corn stover achieves high monomeric sugar concentrations (230 g L−1) during enzymatic hydrolysis and high ethanol concentrations (>10% v/v) during fermentation without hydrolysate purification or concentration.Energy Environ. Sci. 2016; 9: 1237-1245Google Scholar]. As aforementioned, recent efforts also explored the catalytic upgrading of aromatics for jet fuels, yet various challenges exist to lower the costs [8.Wang H. et al.Production of jet fuel-range hydrocarbons from hydrodeoxygenation of lignin over super Lewis acid combined with metal catalysts.ChemSusChem. 2018; 11: 285-291Crossref PubMed Scopus (74) Google Scholar, 9.Liu W. et al.High-efficiency electrochemical hydrodeoxygenation of bio-phenols to hydrocarbon fuels by a superacid-noble metal particle dual-catalyst system.Energy Environ. Sci. 2020; 13: 917-927Crossref Google Scholar, 10.Liu W. et al.Ambient-pressure and low-temperature upgrading of lignin bio-oil to hydrocarbons using a hydrogen buffer catalytic system.Nat. Energy. 2020; 5: 759-767Crossref Scopus (38) Google Scholar]. Biomass-derived biomaterials could improve the value proposition of biorefinery substantially, considering that fuels remain to be bulk and low-value products. The special issue includes several reviews to discuss the potential of biomass-based biomaterials in improving biorefinery economics. These materials include bioplastics [4.Liu Z.-H. et al.Creative biological lignin conversion routes toward lignin valorization.Trends Biotechnol. 2022; 40: 1550-1566Abstract Full Text Full Text PDF Scopus (1) Google Scholar,5.Li F. et al.Microbial lignin valorization through depolymerization to aromatics conversion.Trends Biotechnol. 2022; 40: 1469-1487Abstract Full Text Full Text PDF Scopus (1) Google Scholar,14.Li J. et al.Feedstock design for quality biomaterials.Trends Biotechnol. 2022; 40: 1535-1549Abstract Full Text Full Text PDF Scopus (1) Google Scholar], carbon fibers [14.Li J. et al.Feedstock design for quality biomaterials.Trends Biotechnol. 2022; 40: 1535-1549Abstract Full Text Full Text PDF Scopus (1) Google Scholar], supercapacitors [13.Jia R. et al.Renewable plant-derived lignin for electrochemical energy systems.Trends Biotechnol. 2022; 40: 1425-1438Abstract Full Text Full Text PDF Scopus (2) Google Scholar], nanofertilizers [15.Fu X. et al.Biorefining waste into nanobiotechnologies can revolutionize sustainable agriculture.Trends Biotechnol. 2022; 40: 1503-1518Abstract Full Text Full Text PDF Scopus (1) Google Scholar], biosorbents [2.Zhang W. et al.Design of biomass-based renewable materials for environmental remediation.Trends Biotechnol. 2022; 40: 1519-1534Abstract Full Text Full Text PDF Scopus (2) Google Scholar,14.Li J. et al.Feedstock design for quality biomaterials.Trends Biotechnol. 2022; 40: 1535-1549Abstract Full Text Full Text PDF Scopus (1) Google Scholar], and pavement and construction materials [14.Li J. et al.Feedstock design for quality biomaterials.Trends Biotechnol. 2022; 40: 1535-1549Abstract Full Text Full Text PDF Scopus (1) Google Scholar]. Furthermore, feedstock design has the potential to simplify biorefinery procedures and improve saccharification efficiency and sugar fermentability [19.Yang Y. et al.Plant synthetic biology innovations for biofuels and bioproducts.Trends Biotechnol. 2022; 40: 1454-1468Abstract Full Text Full Text PDF Scopus (2) Google Scholar]. Recent advancements in synthetic biology could substantially empower such feedstock design [19.Yang Y. et al.Plant synthetic biology innovations for biofuels and bioproducts.Trends Biotechnol. 2022; 40: 1454-1468Abstract Full Text Full Text PDF Scopus (2) Google Scholar]. Feedstock design can also be used to improve biomaterial quality and thus enhance the value proposition of the biorefinery [14.Li J. et al.Feedstock design for quality biomaterials.Trends Biotechnol. 2022; 40: 1535-1549Abstract Full Text Full Text PDF Scopus (1) Google Scholar]. Third, besides economics, sustainability remains the primary focus for biomass utilization. Biomass utilization can contribute to sustainability in multiple ways. A lignocellulosic biorefinery could produce biofuels to substitute fossil fuels and reduce carbon emissions substantially. A biorefinery’s impact on global climate change will depend on the level of fossil fuel replacement, and synthetic biology studies on aviation and long-range fuel conversion will empower the biorefinery to maximize the reduction of greenhouse gas (GHG) emissions [8.Wang H. et al.Production of jet fuel-range hydrocarbons from hydrodeoxygenation of lignin over super Lewis acid combined with metal catalysts.ChemSusChem. 2018; 11: 285-291Crossref PubMed Scopus (74) Google Scholar, 9.Liu W. et al.High-efficiency electrochemical hydrodeoxygenation of bio-phenols to hydrocarbon fuels by a superacid-noble metal particle dual-catalyst system.Energy Environ. Sci. 2020; 13: 917-927Crossref Google Scholar, 10.Liu W. et al.Ambient-pressure and low-temperature upgrading of lignin bio-oil to hydrocarbons using a hydrogen buffer catalytic system.Nat. Energy. 2020; 5: 759-767Crossref Scopus (38) Google Scholar,17.Yang S. et al.Metabolic engineering of Zymomonas mobilis for 2, 3-butanediol production from lignocellulosic biomass sugars.Biotechnol. Biofuels. 2016; 9: 189Crossref PubMed Scopus (73) Google Scholar,18.Cruz-Morales P. et al.Biosynthesis of polycyclopropanated high energy biofuels.Joule. 2022; 6: 1590-1160Abstract Full Text Full Text PDF Scopus (4) Google Scholar]. One approach to further decrease the carbon footprint of lignocellulose is to engineer the rhizosphere (the root microbiota) to promote underground biomass growth and reduce fertilizer utilization [12.Moon T.S. SynMADE: synthetic microbiota across diverse ecosystems.Trends Biotechnol. 2022; 40: 1405-1414Abstract Full Text Full Text PDF Scopus (1) Google Scholar]. Lignin has been widely studied to promote plant growth, and processing biorefinery residues into nanofertilizers could also reduce fertilizer usage and improve root growth, both of which will contribute to lowering the carbon footprint in biomass utilization [15.Fu X. et al.Biorefining waste into nanobiotechnologies can revolutionize sustainable agriculture.Trends Biotechnol. 2022; 40: 1503-1518Abstract Full Text Full Text PDF Scopus (1) Google Scholar]. Biomaterials could also mitigate global climate change as carbon sequestration means [20.Scown C. Prospects for carbon-negative biomanufacturing.Trends Biotechnol. 2022; 40: 1415-1424Abstract Full Text Full Text PDF Scopus (1) Google Scholar]. In this issue, Scown reviews how biomanufacturing and biorefinery could contribute to decarbonization [20.Scown C. Prospects for carbon-negative biomanufacturing.Trends Biotechnol. 2022; 40: 1415-1424Abstract Full Text Full Text PDF Scopus (1) Google Scholar]. Besides reducing GHG emissions, biomaterials also address a wider range of challenges, as discussed in the reviews of this special issue [4.Liu Z.-H. et al.Creative biological lignin conversion routes toward lignin valorization.Trends Biotechnol. 2022; 40: 1550-1566Abstract Full Text Full Text PDF Scopus (1) Google Scholar,5.Li F. et al.Microbial lignin valorization through depolymerization to aromatics conversion.Trends Biotechnol. 2022; 40: 1469-1487Abstract Full Text Full Text PDF Scopus (1) Google Scholar,14.Li J. et al.Feedstock design for quality biomaterials.Trends Biotechnol. 2022; 40: 1535-1549Abstract Full Text Full Text PDF Scopus (1) Google Scholar]. Carbon fibers and energy storage materials could contribute to advancements in EVs, wind turbines, and other equipment to improve the efficiency of the transportation and energy sectors and reduce carbon footprints [13.Jia R. et al.Renewable plant-derived lignin for electrochemical energy systems.Trends Biotechnol. 2022; 40: 1425-1438Abstract Full Text Full Text PDF Scopus (2) Google Scholar,14.Li J. et al.Feedstock design for quality biomaterials.Trends Biotechnol. 2022; 40: 1535-1549Abstract Full Text Full Text PDF Scopus (1) Google Scholar]. Bioplastics and lignin–plastic blends could provide compostable and biodegradable alternatives to petrochemical plastics, addressing the plastic waste challenge [4.Liu Z.-H. et al.Creative biological lignin conversion routes toward lignin valorization.Trends Biotechnol. 2022; 40: 1550-1566Abstract Full Text Full Text PDF Scopus (1) Google Scholar,5.Li F. et al.Microbial lignin valorization through depolymerization to aromatics conversion.Trends Biotechnol. 2022; 40: 1469-1487Abstract Full Text Full Text PDF Scopus (1) Google Scholar,14.Li J. et al.Feedstock design for quality biomaterials.Trends Biotechnol. 2022; 40: 1535-1549Abstract Full Text Full Text PDF Scopus (1) Google Scholar]. Biomass-based materials have been widely used for bioremediation, and recent breakthroughs have demonstrated that biomass can be used to design a 3D nanomaterial serving as a sorbent for the ‘forever chemical’, perfluoroalkyl and polyfluoroalkyl substances (PFAS) [2.Zhang W. et al.Design of biomass-based renewable materials for environmental remediation.Trends Biotechnol. 2022; 40: 1519-1534Abstract Full Text Full Text PDF Scopus (2) Google Scholar]. The study highlighted how biopolymers from biomass have unique advantages in biomaterial design, as they can both serve as sorbent components and as the substrate to support and stimulate microorganisms for bioremediation [2.Zhang W. et al.Design of biomass-based renewable materials for environmental remediation.Trends Biotechnol. 2022; 40: 1519-1534Abstract Full Text Full Text PDF Scopus (2) Google Scholar,21.Li J. et al.Sustainable environmental remediation via biomimetic multifunctional lignocellulosic nano-framework.Nat. Commun. 2022; 13: 4368Crossref PubMed Scopus (3) Google Scholar]. In all these applications, the common feature is that biomass-based chemicals and materials can replace petrochemical ones to reduce GHG emissions and mitigate the environmental hazards caused by petrochemical products. Overall, our perspectives on lignocellulosic biomass utilization need to go beyond the traditional cellulosic bioethanol to capture the broad opportunities that biomass can present. We hope this special issue has systemically presented and discussed those opportunities from a biotechnology perspective. Despite the potential of lignocellulosic biomass, the solar-to-molecule energy efficiency for biomass-based products is still limited by the low photosynthesis efficiency of terrestrial land plants. Despite a higher predicted potential, the photosynthesis efficiency for most of the land plants is lower than 1%, which substantially limits the solar energy conversion efficiency to end-products. Algae biofuels and bioproducts have been proposed to address these challenges, considering the relative higher photosynthesis efficiency and productivity [22.Tan C. et al.Carbon-negative synthetic biology: challenges and emerging trends of cyanobacterial technology.Trends Biotechnol. 2022; 40: 1488-1502Abstract Full Text Full Text PDF Scopus (1) Google Scholar,23.Ma Z. et al.Microalgae-based biotechnological sequestration of carbon dioxide for net zero emissions.Trends Biotechnol. 2022; 40: 1439-1453Abstract Full Text Full Text PDF Scopus (2) Google Scholar]. We include two reviews in this special issue to discuss the potential of algal bioproduction [22.Tan C. et al.Carbon-negative synthetic biology: challenges and emerging trends of cyanobacterial technology.Trends Biotechnol. 2022; 40: 1488-1502Abstract Full Text Full Text PDF Scopus (1) Google Scholar,23.Ma Z. et al.Microalgae-based biotechnological sequestration of carbon dioxide for net zero emissions.Trends Biotechnol. 2022; 40: 1439-1453Abstract Full Text Full Text PDF Scopus (2) Google Scholar]. Tan and colleagues focus on the synthetic biology design of cyanobacteria to convert CO2 to a broad range of products [22.Tan C. et al.Carbon-negative synthetic biology: challenges and emerging trends of cyanobacterial technology.Trends Biotechnol. 2022; 40: 1488-1502Abstract Full Text Full Text PDF Scopus (1) Google Scholar], while Ma and coworkers focus on the potential of algae biomass and bioproducts as means of carbon sequestration [23.Ma Z. et al.Microalgae-based biotechnological sequestration of carbon dioxide for net zero emissions.Trends Biotechnol. 2022; 40: 1439-1453Abstract Full Text Full Text PDF Scopus (2) Google Scholar]. While algal bioproduction has the potential to promote sustainability, algal biofuels and commodity products are not yet commercially viable due to some inherent challenges, including low light penetration, dilute cell concentrations, and high harvest cost [24.Long B. et al.Machine learning-informed and synthetic biology-enabled semi-continuous algal cultivation (SAC) to unleash renewable fuel productivity.Nat. Commun. 2022; 13: 541Crossref PubMed Scopus (10) Google Scholar]. Recent breakthroughs in artificial intelligence-guided process optimization addressed some of these challenges, yet commercial platforms are not developed [24.Long B. et al.Machine learning-informed and synthetic biology-enabled semi-continuous algal cultivation (SAC) to unleash renewable fuel productivity.Nat. Commun. 2022; 13: 541Crossref PubMed Scopus (10) Google Scholar]. Recent advancements aim to address this challenge via decoupling light-driven carbon fixation with bioproduction [25.Zhang P. et al.Producing the “molecules of life” from carbon dioxide.Chem. 2021; 7: 3200-3202Abstract Full Text Full Text PDF Scopus (2) Google Scholar]. Both thermocatalysis and electrocatalysis have been proposed to reduce CO2 to intermediates like methanol, ethanol, or acetate, which can be subsequently converted to end-products [26.Zhang P. et al.Chem-bio interface design for rapid conversion of CO2 to bioplastics in an integrated system.Chem. 2022; (Published online September 28, 2022)https://doi.org/10.1016/j.chempr.2022.09.005Abstract Full Text Full Text PDF Google Scholar,27.Cai T. et al.Cell-free chemoenzymatic starch synthesis from carbon dioxide.Science. 2021; 373: 1523-1527Crossref PubMed Scopus (100) Google Scholar]. Recent studies have highlighted that coupling the electrochemical CO2 reduction reaction (CO2RR) to produce more biocompatible ethanol and acetate with downstream bioconversion can deliver a solar-to-biomass conversion rate of 4.6%, much higher than any photosynthetic systems [26.Zhang P. et al.Chem-bio interface design for rapid conversion of CO2 to bioplastics in an integrated system.Chem. 2022; (Published online September 28, 2022)https://doi.org/10.1016/j.chempr.2022.09.005Abstract Full Text Full Text PDF Google Scholar]. Bioplastics production was demonstrated, yet strain engineering could empower a much broader range of bioproducts [26.Zhang P. et al.Chem-bio interface design for rapid conversion of CO2 to bioplastics in an integrated system.Chem. 2022; (Published online September 28, 2022)https://doi.org/10.1016/j.chempr.2022.09.005Abstract Full Text Full Text PDF Google Scholar]. Overall, lignocellulosic biomass, algal bioproduction, and the new electro-bio systems could all provide innovative routes for decarbonized biomanufacturing. The selection of proper products and routes will help to maximize the reduction in carbon footprint [22.Tan C. et al.Carbon-negative synthetic biology: challenges and emerging trends of cyanobacterial technology.Trends Biotechnol. 2022; 40: 1488-1502Abstract Full Text Full Text PDF Scopus (1) Google Scholar]. Biotechnology advancements in these platforms hold great potential to promote the future sustainability of human society.
MoreTranslated text
AI Read Science
Must-Reading Tree
Example
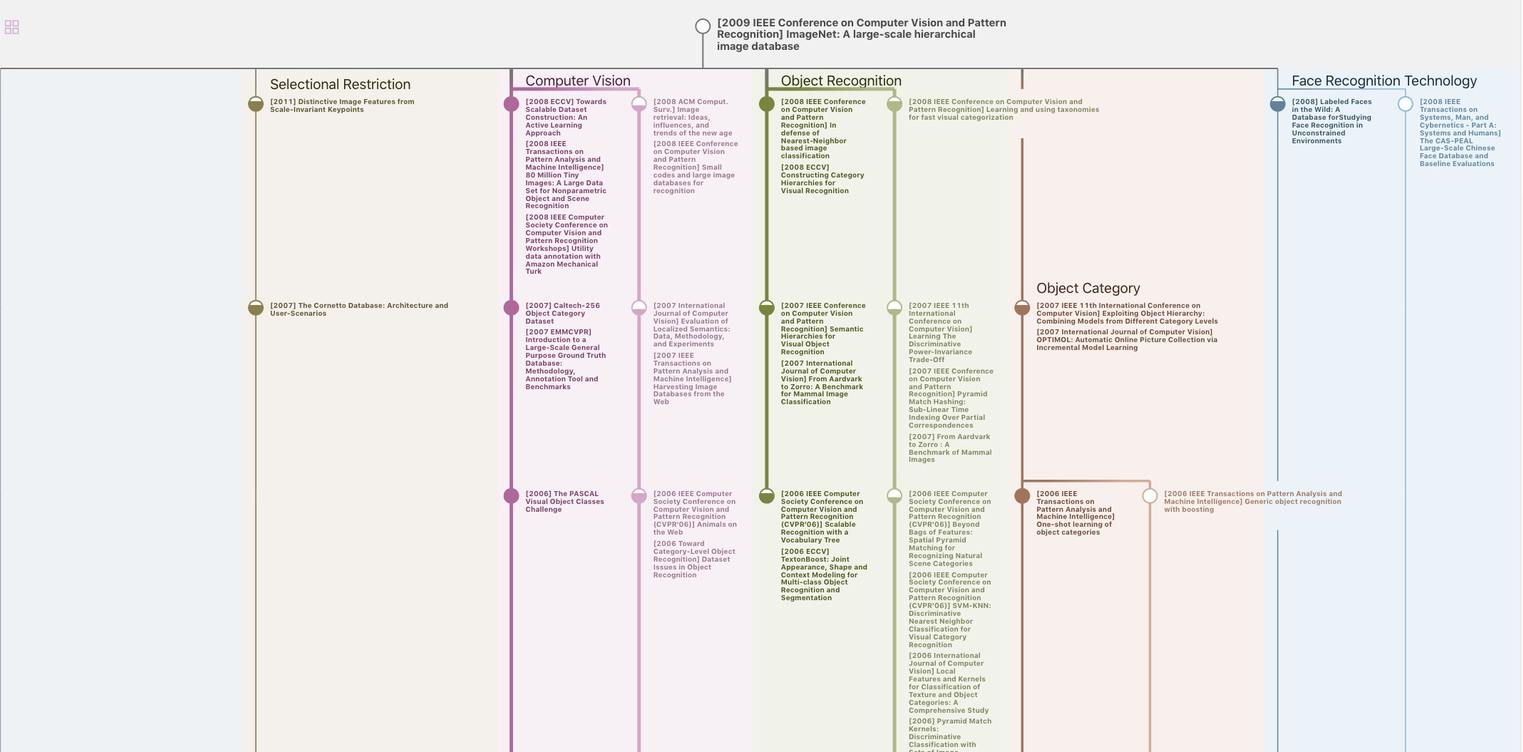
Generate MRT to find the research sequence of this paper
Chat Paper
Summary is being generated by the instructions you defined