(Invited) Volatile Products during Thermal Atomic Layer Etching of Metal Oxides and Elemental Metals
ECS Meeting Abstracts(2021)
摘要
Atomic layer etching (ALE) utilizes sequential, self-limiting reactions to remove material with atomic level control. The first reaction modifies the surface and the second reaction volatilizes the modified surface layer. Thermal ALE removes the modified surface layer using thermal reactions. Thermal Al2O3 ALE was first reported based on fluorination and ligand-exchange reactions [1]. HF was used to fluorinate Al2O3 and Sn(acac)2 was employed to remove fluorinated Al2O3 by ligand-exchange reactions [1]. Subsequent studies revealed that Al2O3 could also be etched with other metal precursors via ligand-exchange such as trimethylaluminum (TMA) [2]. Many other oxide and nitride materials have also been etched using the fluorination and ligand-exchange mechanism [3]. Successful thermal ALE requires that the etch products be stable and volatile. However, what are the volatile products? The most direct way to observe volatile etch products is to employ quadrupole mass spectrometry (QMS). Recently, QMS was used to study the thermal ALE of Al2O3 using HF for fluorination and TMA for ligand-exchange [4]. Gas-phase species were monitored after each reactant exposure. The volatile etch products were consistent with fluorination and ligand-exchange reactions. The ligand-exchange product was AlF(CH3)2 (dimethylaluminum fluoride (DMAF)). DMAF was observed as a dimer with itself (DMAF-DMAF) or with TMA (DMAF-TMA) [4]. The QMS signals in these previous studies of thermal Al2O3 ALE required integration over time. To measure the signals in real time, a new QMS reactor was designed with several improvements. First, the etch products in a N2 carrier gas were allowed to form a molecular beam through expansion. This molecular beam entrained the volatile etch products and directed the etch products with line-of-sight to the QMS ionizer. A skimmer also ensured that the QMS ionizer could be maintained at low pressure in a differentially pumped chamber. This new QMS reactor was then employed to examine different thermal ALE reactions. Recent work using quartz crystal microbalance (QCM) has shown that thermal Ni ALE can be performed using chlorination and ligand-addition reactions [5]. The Ni was first chlorinated using SO2Cl2(sulfuryl chloride). The chlorinated Ni surface was then volatilized by ligand-addition using P(CH3)3 (trimethyl phosphine). To reveal the volatile etch products, NiCl2 powder was used to mimic the chlorinated Ni. NiCl2was exposed to P(CH3)3 and QMS was used to identify the volatile etch products. These studies revealed that the major volatile product was NiCl2(P(CH3)3)2. The isotopic abundance of Cl and Ni helped to confirm this identification. NiCl2(P(CH3)3)2 was observed with peak intensity at 92°C. Motivated by the successful identification of NiCl2(P(CH3)3)2, other metals were examined such as Pd and Pt. PdCl2 and PtCl2 were again used to model chlorination of Pd and Pt using SO2Cl2. As expected from a ligand-addition mechanism, exposure of P(CH3)3 to PdCl2 and PtCl2 led to the observation of PdCl2(P(CH3)3)2 and PtCl2(P(CH3)3)2, respectively. PdCl2(P(CH3)3)2 and PtCl2(P(CH3)3)2 were observed with peak intensities at 150°C and 198°C, respectively. The observation of these metal complexes suggests that Pd and Pt could both be etched using chlorination and ligand-addition reactions. Thermodynamic calculations were also performed to understand the ligand-addition reactions. These calculations revealed that the ligand-addition of P(CH3)3 to NiCl2, PdCl2 and PtCl2 was favorable. The addition of the first P(CH3)3 to NiCl2, PdCl2 and PtCl2 led to increased stability by 2.3, 1.7 and 2.9 eV, respectively. The addition of the second P(CH3)3 to NiCl2P(CH3)3, PdCl2P(CH3)3, and PtCl2P(CH3)3 further increased the stability by 1.9, 1.5 and 1.3 eV, respectively. The formation of metal complexes with Cl and P(CH3)3 ligands is also predicted by the 16 or 18 electron rule for inorganic complexes. The Cl and P(CH3)3ligands add electrons to the outer shell of the metals to form complexes with 16 or 18 electrons. In the case of NiCl2(P(CH3)3)2, PdCl2(P(CH3)3)2 and PtCl2(P(CH3)3)2, the metal complexes all have 16 electrons in their outer shells. Y. Lee and S.M. George, “Atomic Layer Etching of Al2O3 Using Sequential, Self-Limiting Thermal Reactions with Sn(acac)2 and HF”, ACS Nano 9, 2061 (2015). Y. Lee, J.W. DuMont and S.M. George, “Trimethylaluminum as the Metal Precursor for the Atomic Layer Etching of Al2O3 Using Sequential, Self-Limiting Thermal Reactions”, Chem. Mater. 28, 2994 (2016). S.M. George, “Mechanisms of Thermal Atomic Layer Etching”, Acc. Chem. Res. 53, 1151 (2020). J.W. Clancey, A.S. Cavanagh, J.E.T. Smith, S. Sharma and S.M. George, “Volatile Etch Species Produced During Thermal Al2O3 Atomic Layer Etching”, J. Phys. Chem. C 124, 287 (2020). J.A. Murdzek and S.M. George, “Thermal Atomic Layer Etching of Nickel Using SO2Cl2 and P(CH3)3”, Presentation at AVS ALE2020 Workshop, June 29-30, 2020.
更多查看译文
AI 理解论文
溯源树
样例
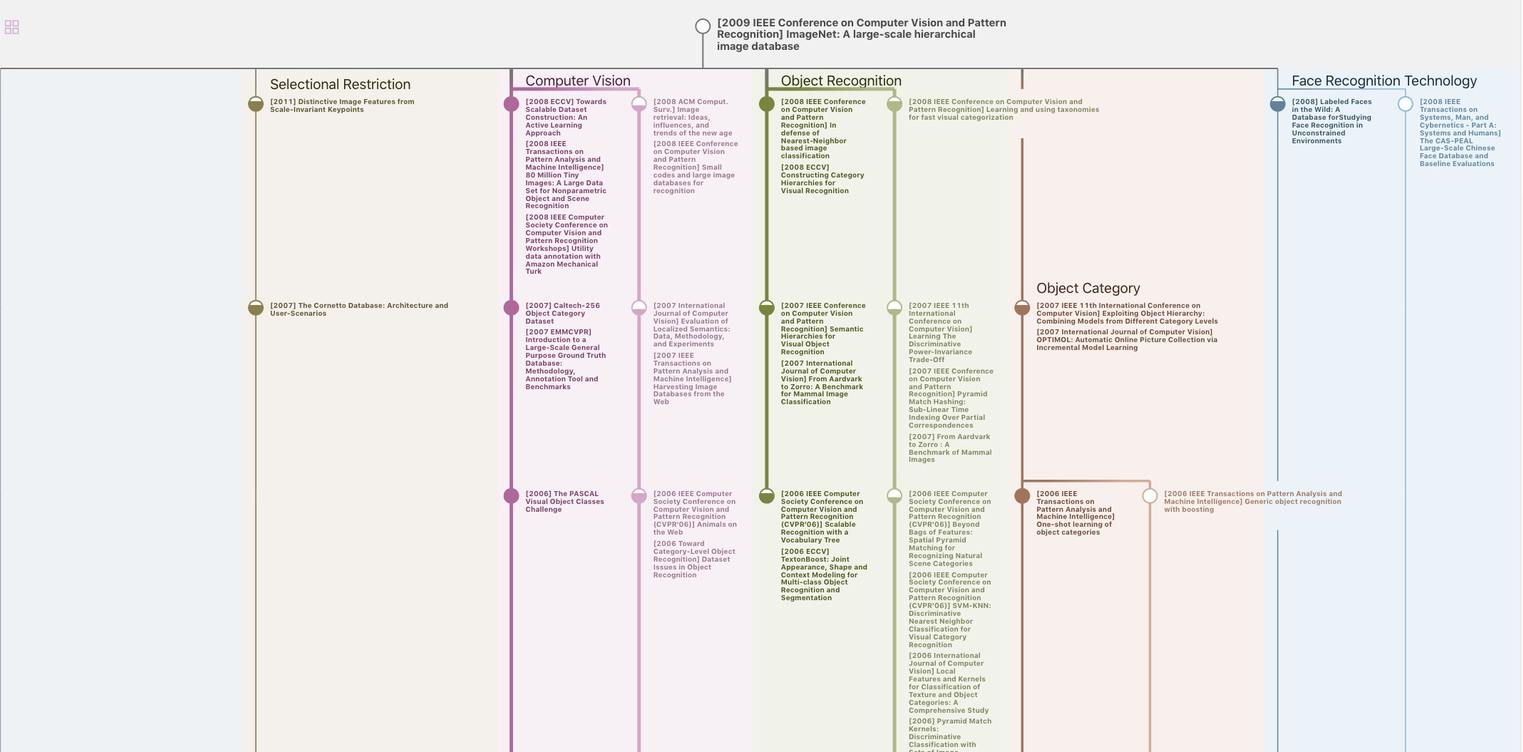
生成溯源树,研究论文发展脉络
Chat Paper
正在生成论文摘要