Distinct and overlapping roles of STAG and PDS5 proteins in cohesin localization and gene expression in embryonic stem cells
semanticscholar(2020)
Abstract
This project identifies and characterizes the pausing of the mitochondrial RNA polymerase (mtRNAP). RNA polymerase pausing is a key regulatory step in the transcription of many nuclear-encoded genes; in contrast, only one promoter-proximal pause for the mtRNAP has been reported. The circular mitochondrial genome has two polycistronic strands, a guanine (G)rich light strand and a G-poor heavy strand. In the nucleus, RNA polymerase II pauses most often in gene promoters, but in the mitochondria, with two polycistronic genes, questions arise as to whether mtRNAP pauses beyond the promoters and how pausing could affect mitochondrial gene expression. With the precision nuclear run-on assay (PRO-seq) in human primary fibroblasts from five individuals, we mapped the locations of transcriptionally active mtRNAP. The results show that there are hundreds of sites where the mtRNAP accumulates, and this includes the one known mtRNAP pause site. In total, with cells from different individuals, we found that as the polymerase transcribes the mitochondrial genome, it pauses over 400 times at consistent locations. These brief stops occur most often after the polymerase has just added a cytosine to the nascent RNA, where the RNA-DNA duplex is thermodynamically stable, and after transcribing through guanine-rich regions. We found there are more than twice as many pause sites on the G-rich strand compared to the G-poor strand. To determine how these G-rich sequences contribute to pausing, we found that they form G-quadruplexes. Stabilization of Gquadruplexes impaired mtRNAP transcription elongation, leading to decreased expression of genes transcribed by mtRNAP, and reduced mitochondrial function. In this presentation, I will discuss how dynamic tuning of nucleic acid secondary structure can regulate transcription and gene expression. 4th Annual Chromatin and Epigenetics Symposium December 3rd, 2020 Investigating the Role of Histone H3 Lysine 4 in Developmental Gene Regulation. Cyril S. Anyetei-Anum and Daniel J. McKay The University of North Carolina at Chapel Hill Histone H3 lysine 4 (H3K4) methylation is an evolutionarily conserved modification of chromatin closely linked to gene activation. The three methylation states of H3K4, monomethylation (me1), dimethylation (me2), and trimethylation (me3), are often used to annotate functional elements of eukaryotic genomes: H3K4me1 marks poised and active enhancers, H3K4me2 is enriched at tissue-specific transcription factor binding sites, enhancers, and promoter edges, and H3K4me3 marks active transcriptional initiation at promoters. These methylation states are mediated by the human methyltransferases MLL3/4, MLL1/2, and SET1A/B, which perform conserved roles in human gene regulation and are commonly mutated in cancers. While these histonemodifying enzymes have been found to be major regulators of development, differentiation, and disease, their enzymatic activity is not limited to histones and they also have non-enzymatic functions. Moreover, the functional study of H3K4 has been difficult because the canonical histone genes in many organisms, including humans, are found at multiple chromosomal locations. Drosophila is an ideal system to study the role of H3K4 in development because its canonical histone genes are clustered at a single chromosomal locus. H3K4 methylation in Drosophila is mediated by the methyltransferase enzymes Trr, Trx, and dSet1 (the human homologs are MLL3/4, MLL1/2, and SET1A/B, respectively). The organization of the canonical histone genes allows for their precise deletion and replacement with transgenic versions that have been mutated to encode for a non-modifiable residue (H3K4R). We previously showed that the wild-type histone replacement transgene supports the propagation of a stable stock lacking all endogenous histone genes (1). By contrast, here we find that the H3K4R replacement transgene is predominantly embryonic lethal, and although H3K4R embryos survive until the end of embryogenesis at expected Mendelian frequencies, they fail to make the transition into larvae. The few H3K4R embryos that hatch have very low survivability and remain stuck in early development. We also find that H3K4R mutant cells survive and appear to proliferate normally with diminished H3K4me1. These data demonstrate a requirement for H3K4 in development, however interpretation is complicated by the fact that H3K4 is subject to multiple methylation states. In future work, we will determine the effects of H3K4 mutation on gene expression through RNA-seq analysis, as well as its impact on the histone post-translational modification landscape through top-down mass spectrometry. Together, this work will extend our understanding of the mechanistic role that histone modifications contribute to developmental gene regulation. Reference: (1) McKay, Daniel J., et al. "Interrogating the function of metazoan histones using engineered gene clusters." Developmental Cell 32.3 (2015): 373-386. The C-terminus of VRK1 governs interactions with nucleosomes to influence histone H3 threonine 3 (H3T3) phosphorylation Authors: Gabrielle Budziszewski1, Yani Zhao2, and Robert K. McGinty1,2,3 1. Biochemistry & Biophysics Department, UNC School of Medicine 2. Division of Chemical Biology & Medicinal Chemistry, UNC School of Pharmacy 3. Lineberger Cancer Center, UNC School of Medicine Vaccinia-related kinase 1 (VRK1) is an understudied nuclear kinase that is known to phosphorylate the histone H3 tail at threonine 3 (H3T3) during mitosis. This modification, also placed by the haspin kinase, is part of a series of events that ensures that daughter cells receive an appropriate amount of DNA following cell division. VRK1 overexpression is correlated with poor survival in breast cancer and glioma, suggesting that the kinase plays a key role in supporting pro-tumorigenic processes. Despite the importance of VRK1 to cell cycle regulation, little is known about the biochemical basis of its activity on nucleosomes. To date, no potent and selective inhibitors for VRK1 exist, highlighting a need to understand how this kinase functions on nucleosomes and may be regulated in the absence of direct inhibitors. Our work reveals that VRK1 directly and stably interacts with nucleosomes with a tight Kd of ~100 nM. VRK1 binds nucleosomes via a C-terminal alternating arginine motif, mutations or truncations of which have been found in human disease. We find that mutation or truncation of this C-terminal arginine motif leads to a 4-50 fold reduction in nucleosome affinity that tracks with mutation severity. Importantly, mutation of the arginine motif results in dissociation of VRK1 from chromatin during mitosis in living cells. VRK1 shows a nucleosome substrate preference and is capable of phosphorylating mononucleosomes in vitro, but not individual histones or histone tetramers bearing the H3 tail. Furthermore, C-terminal arginine mutants are defective in histone H3T3 phosphorylation, suggesting that stable interaction with nucleosomes is required for nucleosome-directed kinase activity at H3T3. The nucleosome acidic patch, a negatively charged region at the dimer interface of H2A/H2B, appears to play some role in binding VRK1 in direct interaction assays, but its presence is negatively correlated with nucleosome-directed kinase activity compared to acidic-patch-neutralized nucleosomes. In contrast, extranucleosomal linker DNA promotes both nucleosome binding and phosphorylation of H3T3. Taken together, this evidence suggests that VRK1 may adopt at least two different binding modes on the nucleosome (acidic patch-governed vs. linker DNA-governed) that either promote or inhibit nucleosome-directed kinase activity based on the position and distance of VRK1 from the H3 tail. Future work will provide further evidence delineating these binding modes and their influence on VRK1 nucleosome-directed kinase activity. Deciphering the histone interactions and reader functions of ASH1L and the PHD-BRD reader domain family in biology and cancer Mutation or dysregulation of the proteins that read, write, and/or erase histone posttranslational modifications are thought to underlie ~50% of all human cancers. One such family of proteins that are frequently altered in cancer is those containing paired histone reading plant homeodomains (PHD) and bromodomains (BRD). ASH1L is a histone H3 lysine-36 (H3K36) dimethylase which is altered in ~7% of cancers and contains a poorly understood triple histone reader PHD-BRD-BAH domain module. Although ASH1L and other PHD-BRD proteins contain commonly studied reader domains and have been linked to cancer, how they regulate normal and cancer development is still not well understood. Thus, there is a critical need to systematically characterize the histone reading interactions and mechanistic processes for ASH1L and the rest of the PHD-BRD family, especially with respect to how these domains in combination can form multivalent interactions with nucleosomes. To address how ASH1L and PHD-BRD readers function, I will employ a suite of innovative histone-binding assays, structural methodologies, and cellular-based analyses. Specifically, I will use modernized histone peptide and nucleosome screening assays, X-ray crystallography and Cryo-EM in parallel, and a knockdown/complementation system of ASH1L in normal and breast cancer cell lines. I hypothesize that ASH1L targets specific acetylated and methylated histone tails via its BRDPHD-BAH module to regulate homeotic gene expression, but that aberrant expression of ASH1L promotes breast cancer through increased expression of pro-growth and survival genes. Through this examination, this work aims to further decipher the “Histone Code” and provide new insights into cancer biology Title: A cohesin cancer mutation reveals a role for the hinge domain in genome organization and gene expression The cohesin complex functions in the spatial organization of the genome in
MoreTranslated text
AI Read Science
Must-Reading Tree
Example
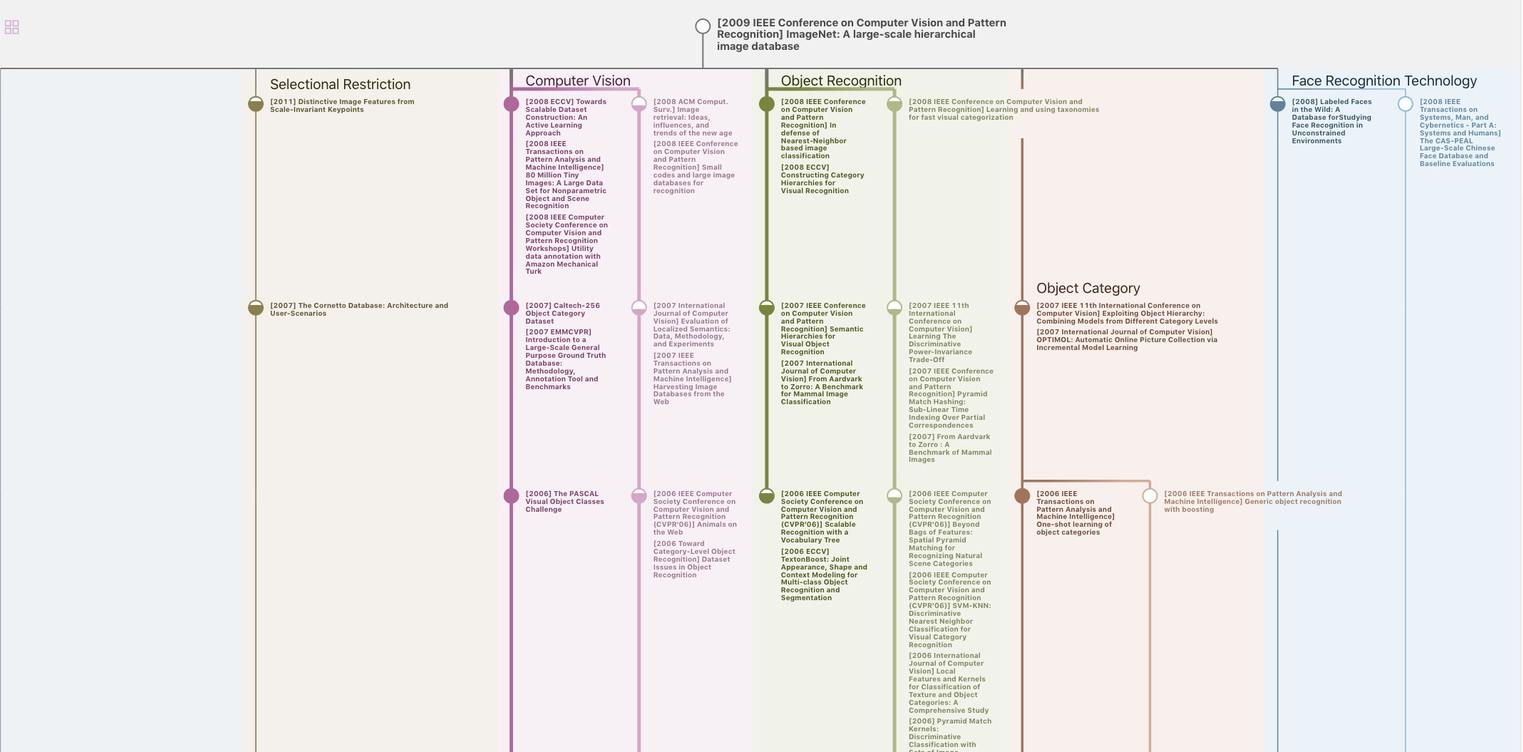
Generate MRT to find the research sequence of this paper
Chat Paper
Summary is being generated by the instructions you defined