TET2 deficiency cooperates with CBFB-MYH11 to induce acute myeloid leukaemia and represents an early leukaemogenic event
British Journal of Haematology(2022)
Abstract
Acute myeloid leukaemia (AML) represents an aggressive cancer entity, which develops through clonal evolution, propagated by accumulating independent somatic molecular and chromosomal aberrations in haematopoietic stem and progenitor cells (HSPCs).1 Consistent with the significant heterogeneity and complexity of AML genomes, AML patients demonstrate highly variable response rates and overall survival when treated with conventional therapeutic regimens. Recurrent AML aberrations can emerge in pre-malignant HSPCs that propagate a state of clonal haematopoiesis before the HSPCs acquire additional driver aberrations, promoting definitive malignant transformation into overt AML.2 Loss-of-function (LOF) mutations of the epigenetic regulator tet methylcytosine dioxygenase 2 (TET2) are frequently observed in healthy patients with clonal haematopoiesis and concur with other genetic aberrations in patients with myelodysplastic syndrome (MDS), myeloproliferative neoplasm (MPN), and AML.3, 4 Mutations identified in the TET2 gene frequently confer a LOF, resulting in preferential hypermethylation of enhancers and silencing of tumour suppressors as well as genes critical for haematopoietic development, including key transcriptional regulators of myeloid differentiation such as RUNX1 and PU1.5 Consistently, TET2 LOF mutations promote clonal haematopoiesis and predispose to malignant myeloid transformation.5, 6 In mouse models, TET2 deficiency alone leads to enhanced self-renewal of HSPCs and, over time, to a chronic myelomonocytic leukaemia-like disease rather than AML, and thus requires cooperating genetic driver aberrations for full transformation into overt AML.4-7 The inversion of chromosome 16 [inv(16)] is among the most frequent chromosomal aberrations in adult and paediatric AML and defines a prognosis-relevant genetic AML subclass. Inv(16) juxtaposes the five N-terminal exons of the CBFB gene in frame to variable lengths of the C-terminal region of the MYH11 gene. The resultant CBFB-MYH11 fusion protein exerts its leukaemogenic potential through aberrant transcriptional activation and repression. More specifically, CBFB-MYH11 proteins oligomerize into high molecular weight filamentous complexes, which sequestrate the CBFB binding partner RUNX1 in the cytosol, leading to repression of RUNX1 target genes, such as CEBPA and PU1, that are essential for proper myeloid differentiation.8 CBFB-MYH11 sequestration of RUNX1 also abrogates recruitment of DNMT3A to RUNX1 target gene promotors, leading to impaired promotor methylation/silencing of RUNX1 target genes in AML.9 In addition, CBFB-MYH11 co-localizes to RUNX1 bound promotors and confers transcriptional repression through its association with co-repressor complexes and recruitment and activation of histone deacetylases.10 Finally, CBFB-MYH11 also operates in a complex with other transcription factors at promotors conferring transcriptional activation of genes associated with HSC self-renewal.10 Genetic studies in patients and mouse models have demonstrated that inv(16) can emerge as the initial or secondary event in the course of leukaemic transformation, and requires cooperation with other recurrent mutations, such as KIT, FLT3-ITD, and RAS, to generate overt AML with high penetrance (2, 8–10).2, 11-13 Hence, in alignment with TET2 mutations, inv(16) alone generally fails to induce overt AML in patients.8 However, mice with inv(16) can develop haematological malignancies with low penetrance and substantial latency, indicating that inv(16) mice exhibit an inherent predisposition and requirement for acquisition of additional spontaneous genetic aberrations for full leukaemic transformation.14, 15 Given that TET2 LOF mutations have been identified as rare concurrent ‘partners in crime’ in approximately 3% of inv(16) AML patients, we set out to explore whether expression of the CBFB-MYH11 fusion protein in TET2 deficient HSPCs would confer leukaemic transformation in mice.12 In order to investigate whether inv(16) promotes leukaemic transformation in a TET2 deficient background, primitive KIT+ HSPCs were purified from the bone marrow (BM) of Tet2−/− mice and transduced with a retroviral vector co-expressing the human CBFB-MYH11 fusion protein and green fluorescent protein (GFP) (Figure 1A; Figure S1). GFP+ cells were subsequently fluorescence-activated cell-sorted (FACS)- and transplanted into lethally irradiated primary recipient mice. Strikingly, all mice developed overt AML within a period of 34–59 days (median survival, 53 days) demonstrating that inv(16) and Tet2−/− cooperatively promote leukaemic transformation with 100% penetrance and a short latency (Figure 1B). The latter is consistent with a high cooperative leukaemogenic activity of inv(16) and Tet2−/−, given that Tet2−/− deficient HSPCs, or HSPCs retrovirally transduced with the CBFB-MYH11 vector applied in the present study, have a more than eightfold longer latency (400–600 days) and more than eightfold lower penetrance (0–40%) for malignant transformation after transplantation into primary recipient mice.7, 15 Primary recipient mice sacrificed after early, median, and late onset of AML, all exhibited similar high frequencies of GFP+ inv(16)/Tet2−/− AML cells in the BM and spleen (Figure 1C), and substantial splenomegaly (Figure 1D) with loss of splenic architecture due to a marked infiltration of AML cells (Figure 1F). Clonogenic GFP+ inv(16)/Tet2−/− AML cells from primary recipient mice with overt AML demonstrated a high replicative potential upon serial re-plating in colony-forming cell (CFC) assays, as compared to clonogenic wild-type (wt) HSPCs (Figure 1E). AML cells from mice with early, median, and late onset of AML did not show any difference in replicative potential in our serial re-plating assays. Consistently, transplantation of primary GFP+ inv(16)/Tet2−/− AML cells into secondary recipient mice gave rise to AML with a markedly accelerated onset of disease after 12–15 days, independent of the latency of primary AML development (Figure 1G). Together, these results strongly support that inv(16) cooperates with TET2 deficiency to generate overt AML in mice similar to what is observed in patients with AML harbouring cooperating inv(16) and TET2 LOF mutations. Significantly, the full penetrance and the very short latency for AML development compared to other inv(16) AML mouse models with concurrent KIT, FLT3-ITD, and RAS mutations suggest that concurrent TET2mut in inv(16) AML confers a more aggressive leukaemic phenotype.11, 13 Immunophenotypic analyses of murine inv(16)/Tet2−/− AML HSPC hierarchies demonstrated high abundance of leukaemic granulocyte-monocyte progenitors (L-GMPs) in nine out of 11 primary AML mice (Figure 1H; Figures S2–S4A; Table S1), which recapitulated the immunophenotypic HSPC hierarchies observed in six out of eight inv(16) AML patients (Figure 1I; Figure S4B; Table S2). Consistently, inv(16)/Tet2−/− AML mice also demonstrated a high abundance of immunophenotypic monocytes at the expense of mature granulocytes, which mimics the monocytic phenotype of blast cells in inv(16) AML patients (Figure S4C,D). In order to identify a transcriptional programme shared by the inv(16)/Tet2−/− AML mouse model and inv(16) AML patients, FACS-sorted L-GMPs and normal GMPs from secondary inv(16)/Tet2−/− AML and wt mice as well as inv(16) AML patients and healthy donors were subjected to microarray gene expression profiling. Subsequent comparative gene expression analysis of AML samples with their corresponding normal counterpart (Figures S5 and S6) identified a total of 1112 differentially expressed genes (DEGs) in L-GMPs versus normal GMPs for the inv(16) AML patients and 473 DEGs for the inv(16)/Tet2−/− AML model (Figure S7A; Table S3).16 After filtering for gene orthologs among species, we identified 63 commonly DEGs in murine and patient-derived inv(16) AML, corresponding to threefold more DEGs than expected by random distribution (63 vs. 19 genes, Figure S7B). This implicates that inv(16) launches a core set of DEGs in L-GMPs versus normal GMPs, which is independent of species and the type of concurrent mutation. Among the shared DEG in murine and patient-derived inv(16) AMLs, we identified monocytic markers (i.e., CD52, CD68, Figure 1K) and aberrantly high expression of the CD73 (NT5E) enzyme (Figure S7C), which is a recognized tumour-promoting factor in solid cancers that can be targeted by small molecule inhibitor and antibody therapies.17 Complementary gene set enrichment analyses (GSEA) of DEG in L-GMPs versus normal GMPs (AMLvN) demonstrated that the inv(16)/Tet2−/− AML mouse model and inv(16) AML patients share common functional AMLvN gene expression signatures that reflect activation of an inflammatory/infection response, activation of “oncogenic” signalling pathways (RAS, PI3K/AKT, TGFB, NFKB, STAT5A), hypoxia, and a low proliferative activity (Figure S8; Table S8). In addition, GSEA identified enrichment of an inv(16) AML gene expression signature in both our inv(16)/Tet2−/− mouse model and human inv(16) AML patients, and signatures of various other cancer entities as well as functional signatures commonly associated with cancer, such as cellular stress responses (i.e. UV/irradiation signatures), and epigenetic gene regulation (i.e., methylation/polycomb repressor complex signatures) (Figure S8; Table S8). Overall, analyses of DEG and GSEA highlight that our inv(16)/Tet2−/− AML mouse model and inv(16) AML patients share a set of functional gene expression signatures reflecting transcriptional and epigenetic rewiring, which propagates a common leukaemic phenotype. In the context of recent studies, this suggests that TET2 deficiency and CBFB-MYH11 cooperatively rewire gene expression through (i) hypermethylation and silencing of enhancers/promotors and (ii) direct or indirect aberrant activation and repression of RUNX1 occupied promotors, which ultimately confer decreased expression of tumour suppressor and myeloid differentiation genes, and expression of genes associated with HSC self-renewal.4-6, 8-10 Genetic analyses of nine inv(16)/TET2mut AML patients demonstrated variant allele frequencies of TET2mut in the range of 0.36–0.52 (Figure 2A). None of the AML patients had concurrent mutations of other enzymes regulating DNA methylation (i.e., DNMT3A and IDH1/2). Notably, seven out of nine inv(16)/TET2mut AML patients exhibited concurrent mutations in signalling molecules (KIT, KRAS, NRAS, CSF3R, and NF1), whereas only one out nine lacked any detectable concurrent mutations. These findings imply that heterozygous TET2mut is confined in dominant AML founder clones and, consequently, represents an early and potential initiating event of inv(16) AML, which frequently cooperates with mutations in signalling molecules to confer leukaemic transformation. Importantly, one inv(16)/TET2mut AML patient exhibited no detectable co-mutation, suggesting that inv(16) and TET2mut alone can potentially propagate full leukaemic transformation in AML patients. Notably, our cohort of nine inv(16)/TET2mut AML patients demonstrated a slightly higher relapse rate and poorer survival compared to those reported for a larger historic cohort of 201 inv(16) AML patients (Figure 2B,C).18 Although, the prognostic value of rare concurrent TET2mut in inv(16) AML patients remains elusive in the clinical setting, the full penetrance and very short latency of AML development exhibited by the inv(16)/Tet2−/− AML mouse model supports that TET2mut might confer an adverse impact on the clinical outcome of inv(16) AML patients, similar to what has been reported for other favourable risk AML subclasses with frequent concurrent TET2mut (i.e. bi-allelic CEBPAmut, NPM1mut).19 Hence, inv(16)/TET2mut AML patients might potentially benefit from comprehensive minimal residual disease (MRD) monitoring and MRD-guided therapeutic stratification. In conclusion, the present study reports a novel inv(16)/Tet2−/− AML mouse model, which mirrors the phenotype and core transcriptional programme of inv(16) AML patients. Significantly, this AML model provides mechanistic evidence that inv(16) and TET2 LOF mutations cooperatively induce leukaemic transformation in AML. Complementary genetic analyses of AML patients further suggest this to be an initial event in inv(16) AML, which frequently cooperates with mutational activation of signalling molecules. The authors thank Kasper Rasmussen for the Tet2 KO mouse model and Bente Langelung Kristensen for professional animal care. The authors are grateful to all contributing bone marrow donors and AML patients. This work was supported by grants from the Danish Cancer Society (grant no. R167-A10932-17-S2, ME; grant no. R90-A5960-14-S2, KR; grant no. R146-A9228-16-S2, ASH). KTM is supported by a clinical research fellowship and a centre grant from the Novo Nordisk Foundation (grant no. 100191, Novo Nordisk Foundation Center for Stem Cell Biology; DanStem; grant no. NNF17CC0027852) and by grants from the Danish Council for Strategic Research (grant no. 133100153), the Danish Cancer Society (grant no. R72-A4572-13-S2), Børnecancerfonden (2016-0255), and Læge Sofus Carl Emil Friis og Hustru Olga Doris Friis Foundation, and Tømrermester Jørgen Holm og Hustru Elisa, Brødrene Hartmans Fond, F. Hansen's Mindelegat. The authors declare no conflict of interest. KR and KTM: design of the study. KR, ME, AM and AH: data collection and conduct of experiments. FR, KD and KTM: collection of patient samples and provision of clinical data. KR, ME, MA, KJW, FR, KD and KTM: analysis and interpretation of the data. KR and KTM: writing of the manuscript. All authors read and approved the final manuscript. Please note: The publisher is not responsible for the content or functionality of any supporting information supplied by the authors. Any queries (other than missing content) should be directed to the corresponding author for the article.
MoreTranslated text
Key words
acute myeloid leukaemia,early leukaemogenic event,deficiency
AI Read Science
Must-Reading Tree
Example
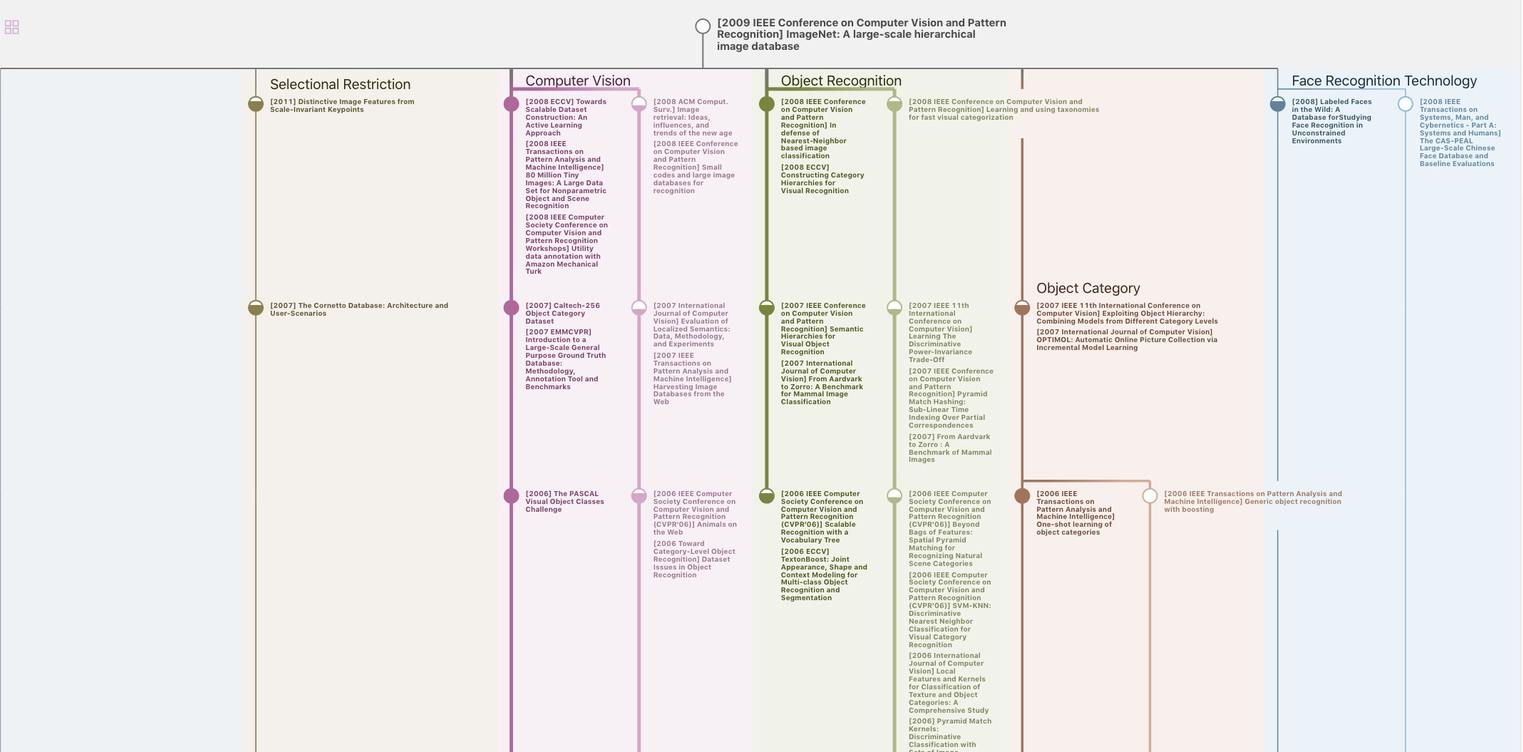
Generate MRT to find the research sequence of this paper
Chat Paper
Summary is being generated by the instructions you defined