Genome Sequence Resource of Colletotrichum horii, an Important Pathogenic Fungus Threatening Persimmon Production
PLANT DISEASE(2022)
Abstract
HomePlant DiseaseVol. 106, No. 3Genome Sequence Resource of Colletotrichum horii, an Important Pathogenic Fungus Threatening Persimmon Production PreviousNext RESOURCE ANNOUNCEMENT OPENOpen Access licenseGenome Sequence Resource of Colletotrichum horii, an Important Pathogenic Fungus Threatening Persimmon ProductionHanyue Fan, Yongkuan Li, Sadaruddin Chachar, Yong Yang, and Changfei GuanHanyue FanState Key Laboratory of Crop Stress Biology for Arid Areas, College of Horticulture, Northwest A&F University, Yangling, Shaanxi 712100, ChinaSearch for more papers by this author, Yongkuan LiState Key Laboratory of Crop Stress Biology for Arid Areas, College of Horticulture, Northwest A&F University, Yangling, Shaanxi 712100, ChinaSearch for more papers by this author, Sadaruddin ChacharState Key Laboratory of Crop Stress Biology for Arid Areas, College of Horticulture, Northwest A&F University, Yangling, Shaanxi 712100, ChinaSearch for more papers by this author, Yong YangState Key Laboratory of Crop Stress Biology for Arid Areas, College of Horticulture, Northwest A&F University, Yangling, Shaanxi 712100, ChinaSearch for more papers by this author, and Changfei Guan†Corresponding author: C. Guan; E-mail Address: guanchangfei@nwafu.edu.cnhttps://orcid.org/0000-0002-0486-1321State Key Laboratory of Crop Stress Biology for Arid Areas, College of Horticulture, Northwest A&F University, Yangling, Shaanxi 712100, ChinaSearch for more papers by this author AffiliationsAuthors and Affiliations Hanyue Fan Yongkuan Li Sadaruddin Chachar Yong Yang Changfei Guan † State Key Laboratory of Crop Stress Biology for Arid Areas, College of Horticulture, Northwest A&F University, Yangling, Shaanxi 712100, China Published Online:8 Mar 2022https://doi.org/10.1094/PDIS-11-21-2417-AAboutSectionsView articlePDFSupplemental ToolsAdd to favoritesDownload CitationsTrack Citations ShareShare onFacebookTwitterLinked InRedditEmailWechat View articleGenome AnnouncementColletotrichum horii causes anthracnose disease on persimmon. It is an important fungus that seriously threatens persimmon production worldwide. To date, the genome of C. horii has not been reported, although various genomic sequences are available for the C. gloeosporioides species complex to which the pathogen belongs. Here, we isolated C. horii FJ-1 from persimmon in China and presented the high-quality and completed genome of C. horii with a total size of 74.32 Mb by using Nanopore sequencing technology. The genome of C. horii FJ-1 was assembled into 42 contigs with an N50 of 3.1 Mb. In total, 15,529 genes were predicted, among which 358 genes encoded the candidate effector proteins. Our results provide the first genome resource for determining the pathogenic mechanism of C. horii to plant and identifying potential resistant candidates for persimmon-breeding programs.The genus Colletotrichum comprises nine major clades (Cannon et al. 2012) and includes 252 species and 15 complexes (Talhinhas and Baroncelli 2021). Colletotrichum spp. are important plant pathogens that can cause anthracnose in economic crops such as persimmon (Zhang and Xu 2003), banana (Chillet et al. 2006), mango (Li et al. 2019), chili (Than et al. 2008), tomato (Barksdale 1972), and strawberry (Xiao et al. 2004). The genus has been voted as one of the top 10 fungal plant pathogens in molecular plant pathology (Dean et al. 2012).Persimmon (Diospyros kaki Thunb.), one of the most important fruit trees in the world, is primarily cultivated in East Asia (Kanzaki and Nara 2016; Luo and Wang 2008). Persimmon anthracnose is a serious disease occurring in many countries where persimmon is cultivated. Based on molecular and morphological analyses, the pathogen of persimmon anthracnose was renamed C. horii by Weir and Johnston (2010) and belongs to the C. gloeosporioides species complex. Anthracnose symptoms first appear in the spring as spots; then, the spots develop into dark lesions. Under favorable conditions, adjacent lesions may coalesce, increasing in size until the entire twig is infected (Xie et al. 2010). As a destructive disease of persimmon trees, C. horii can cause leaf defoliation, fruit rot, and even the death of the whole plant (Zhang and Xu 2005). In some growing areas of persimmon in China, the anthracnose could reduce the yield of persimmon by more than 50% (Deng et al. 2019). However, due to a lack of the reference genome, the molecular pathogenic mechanism of C. horii to the plant remains unknown. Hence, the genome analysis of C. horii is a significant step to understand its pathogenic mechanism and persimmon–C. horii interactions, thereby providing a reference for the study of effective management strategies.The strain FJ-1 was isolated from diseased persimmon branches (D. kaki L. ‘Fuping Jianshi’) from the National Field Genebank for Persimmon, Yangling, Shaanxi, China (34°17′42.80″ N, 108°04′8.21″ E). Genomic DNA was extracted from 7-day-old cultures grown on potato dextrose agar (PDA) using the E.Z.N.A. fungal DNA kit (Omega Bio-Tek). Based on the concatenated dataset of actin, chalcone synthase, glyceraldehyde 3-phosphate dehydrogenase, and β-tubulin-2 gene sequences of C. horii and those of other C. gloeosporioides species complex members (Talhinhas and Baroncelli 2021), a maximum-likelihood phylogenetic tree was constructed with MEGA v7.0 (Kumar et al. 2016), and the bootstrap value was set as 1,000. The phylogenetic tree showed that the isolate FJ-1 clustered with the C. horii strain with high bootstrap support (Supplementary Fig. S1). The genome of C. horii strain FJ-1 was sequenced using Oxford Nanopore Technologies and sequencing was performed at the Beijing Biomarker Technologies Co., Ltd. (Beijing, China). The purity, concentration, and integrity of genomic DNA were examined by Nanodrop, Qubit, and 0.35% agarose gel electrophoresis, respectively. The BluePippin automatic nucleic acid recovery system was used to recover large fragments of DNA. The library was constructed with an SQK-LSK109 kit and sequenced with a Nanopore III sequencer. After filtering out low-quality and short-length subreads (<2,000 bp), the remaining subreads were assembled to contigs without gaps by using NECAT v0.0.1 (https://github.com/xiaochuanle/NECAT) software.In total, 6.9 GB of raw data (92.84× genome coverage) was obtained, and a complete genome was assembled with a total length of 74.32 Mb. The genome contained 42 contigs, with an N50 of 3.1 Mb, a maximum length of 8.8 Mb, and a GC content of 46.79% (Table 1; Supplementary Tables S1 and S2). The completeness of the genome assembly was assessed using BUSCO v4.0 (Manni et al. 2021), resulting in a coverage rate of 97.93% (Supplementary Fig. S2). With the help of LTR_FINDER v1.05 (Xu and Wang 2007), MITE-Hunter (v 2010-09-29) (Han and Wessler 2010), RepeatScout v1.0.5 (Price et al. 2005), and PILER-DF v2.4 (Edgar and Myers 2005), the repetitive sequence database of the fungal genome was constructed based on the principles of structural prediction and de novo prediction. The database was classified by PASTEClassifier v2.0 (Wicker et al. 2007) and then merged with Repbase v14.02 (Jurka et al. 2005) database as the final repetitive sequence database. Based on the constructed repetitive sequence database, the repetitive sequence of this fungus was predicted using RepeatMasker v4.0.6 (Smit et al. 2013-2015) software, and a repetitive sequence of 15.83 Mb with a repetitive sequence ratio of 21.30% was obtained. Genscan v1.0 (Burge and Karlin 1997), Augustus v2.4 (Stanke and Waack 2003), GlimmerHMM v3.0.4 (Majoros et al. 2004), GeneID v1.4 (Blanco et al. 2007), and SNAP (v 2006-07-28) (Korf 2004) were used to predict the gene structure from scratch. GeMoMa v1.3.1 (Keilwagen et al. 2016) was used for prediction based on homologous protein, and EVM v1.1.1 (Haas et al. 2008) was adopted to integrate the prediction results obtained with the above two methods. Consequently, 15,529 genes were obtained, with an average length of 1,863.38 bp. For the prediction of noncoding RNAs, tRNAscan-SE v1.3 software (Lowe and Eddy 1997) was used to predict transfer RNA in the genome, and Infenal1.1 (Nawrocki and Eddy 2013) was used to predict ribosomal RNA in the genome based on the Rfam (Nawrocki et al. 2014) database. By comparing the predicted protein sequences with those in the Swiss-Prot database and using GenBlastA v1.0.4 software (She et al. 2009), we searched the genome for homologous gene sequences (possible genes). Then, GeneWise v2.4.1 (Birney et al. 2004) was used to search for early termination codons and frameshift mutations in the gene sequences; thus, two pseudogenes were obtained (Table 1).Table 1. Statistical features of the Colletotrichum horii FJ-1 genomeFeaturesStatisticsGenome size (bp)74,324,508Genome coverage92.84×Contig number42Contig N50 (bp)3,135,412Maximum contig length (bp)8,827,220GC content (%)46.79Complete BUSCOs (%)97.93Gene number15,529Average gene length (bp)1,863.38Repetitive sequence (%)21.30Ribosomal RNA101Transfer RNA413Pseudogene2Signal peptide2,111Secreted proteins1,613Candidate effectors358Table 1. Statistical features of the Colletotrichum horii FJ-1 genomeView as image HTML Genome function annotation includes general database annotation and proprietary database annotation. The general annotation results were obtained by comparing the predicted gene sequences with functional databases such as EuKaryotic Orthologous Groups (KOG) (Tatusov et al. 2000), Kyoto Encyclopedia of Genes and Genomes (KEGG) (Kanehisa et al. 2004), Swiss-Prot (Boeckmann et al. 2003), TREMBL (Boeckmann et al. 2003), and nonredundant (NR) (Deng et al. 2006). Among all coding genes, 15,449 (99%) genes were annotated by the NR database, 7,218 (46%) were classified using the gene ontology database, 3,620 (23%) were assigned to the KEGG database, and 7,080 (45%) were mapped to the KOG database (Supplementary Figs. S3, S4, and S5; Supplementary Table S3). The functional annotation of 1,034 carbohydrate-active enzyme (CAZy) genes was obtained using HMMER v2.2.1 software (Eddy 1998) in the CAZy database (Cantarel et al. 2009) (Supplementary Fig. S5). In total, 141 transport proteins and 4,964 pathogen–host interaction proteins were annotated by the TCDB (Saier et al. 2006) and PHI (Winnenburg et al. 2006) databases, respectively. Based on the protein sequences of all predicted genes, 2,111 proteins containing signal peptides were predicted using SignalP 4.0 software (Petersen et al. 2011). The remaining 1,613 proteins after removing the protein containing a transmembrane helix from the predicted protein containing a signal peptide are secretory proteins. Then, we predicted 358 fungal candidate effector proteins after further analysis of secreted proteins by EffectorP v1.0 software (Sperschneider et al. 2015).Additionally, we compared the FJ-1 genome assembly statistics with those of seven strains (Cg56, ICMP18580, HC540, CgLH19, Cg01, hn1205-1, and CF75) belonging to C. gloeosporioides complexes in the NCBI database (Supplementary Table S4). The genome assembly statistics of C. horii strain FJ-1 were comparable with those of other C. gloeosporioides species complex.Taken together, these findings provide a significant discovery for the genome analysis of C. horii, which can help us to better understand its pathogenic mechanism and persimmon–C. horii interactions. In turn, such understanding can contribute to the effective control methods of this disease. This Whole-Genome Shotgun project has been deposited at DNA Data Bank of Japan/European Nucleotide Archive/GenBank under the accession JAHZMN000000000 (BioProject: PRJNA748574, BioSample: SAMN20339690). The version described in this article is version JAHZMN010000000.The author(s) declare no conflict of interest.Literature CitedBarksdale, T. H. 1972. Resistance in tomato to six anthracnose fungi. Phytopathology 62:660-663. https://doi.org/10.1094/Phyto-62-660 Crossref, ISI, Google ScholarBirney, E., Clamp, M., and Durbin, R. 2004. GeneWise and Genomewise. Genome Res. 14:988-995. https://doi.org/10.1101/gr.1865504 Crossref, ISI, Google ScholarBlanco, E., Parra, G., and Guigó, R. 2007. Using geneid to identify genes. Curr. Protoc. Bioinf. 4:4.3.1-4.3.28. https://doi.org/10.1002/0471250953.bi0403s18 Google ScholarBoeckmann, B., Bairoch, A., Apweiler, R., Blatter, M. C., Estreicher, A., Gasteiger, E., Martin, M. J., Michoud, K., O’Donovan, C., Phan, I., Pilbout, S., and Schneider, M. 2003. The SWISS-PROT protein knowledgebase and its supplement TrEMBL in 2003. Nucleic Acids Res. 31:365-370. https://doi.org/10.1093/nar/gkg095 Crossref, ISI, Google ScholarBurge, C., and Karlin, S. 1997. Prediction of complete gene structures in human genomic DNA. J. Mol. Biol. 268:78-94. https://doi.org/10.1006/jmbi.1997.0951 Crossref, ISI, Google ScholarCannon, P. F., Damm, U., Johnston, P. R., and Weir, B. S. 2012. Colletotrichum—Current status and future direction. Stud. Mycol. 73:181-213. https://doi.org/10.3114/sim0014 Crossref, ISI, Google ScholarCantarel, B. L., Coutinho, P. M., Rancurel, C., Bernard, T., Lombard, V., and Henrissat, B. 2009. The Carbohydrate-Active EnZymes database (CAZy): An expert resource for Glycogenomics. Nucleic Acids Res. 37:D233-D238. https://doi.org/10.1093/nar/gkn663 Crossref, ISI, Google ScholarChillet, M., Hubert, O., Rives, M. J., and de Lapeyre de Bellaire, L. 2006. Effects of the physiological age of bananas on their susceptibility to wound anthracnose due to Colletotrichum musae. Plant Dis. 90:1181-1185. https://doi.org/10.1094/PD-90-1181 Link, ISI, Google ScholarDean, R., Van Kan, J. A. L., Pretorius, Z. A., Hammond-Kosack, K. E., Di Pietro, A., Spanu, P. D., Rudd, J. J., Dickman, M., Kahmann, R., Ellis, J., and Foster, G. D. 2012. The Top 10 fungal pathogens in molecular plant pathology. Mol. Plant Pathol. 13:414-430. https://doi.org/10.1111/j.1364-3703.2011.00783.x Crossref, ISI, Google ScholarDeng, Q. E., Ding, X. Y., Li, J. A., Cui, L. K., and Xu, J. Q. 2019. Morphological characteristics and genetic diversity of Colletotrichum horii infecting persimmon tree in China. Eur. J. Plant Pathol. 156:437-449. https://doi.org/10.1007/s10658-019-01893-x Crossref, ISI, Google ScholarDeng, Y. Y., Li, J. Q., Wu, S. F., Zhu, Y. P., Chen, Y. W., and He, F. C. 2006. Integrated nr database in protein annotation system and its localization. Comput. Eng. 32:71-74. Google ScholarEddy, S. R. 1998. Profile hidden Markov models. Bioinformatics 14:755-763. https://doi.org/10.1093/bioinformatics/14.9.755 Crossref, ISI, Google ScholarEdgar, R. C., and Myers, E. W. 2005. PILER: Identification and classification of genomic repeats. Bioinformatics 21:i152-i158. https://doi.org/10.1093/bioinformatics/bti1003 Crossref, ISI, Google ScholarHaas, B. J., Salzberg, S. L., Zhu, W., Pertea, M., Allen, J. E., Orvis, J., White, O., Buell, C. R., and Wortman, J. R. 2008. Automated eukaryotic gene structure annotation using EVidenceModeler and the program to assemble spliced alignments. Genome Biol. 9:R7. https://doi.org/10.1186/gb-2008-9-1-r7 Crossref, ISI, Google ScholarHan, Y., and Wessler, S. R. 2010. MITE-Hunter: A program for discovering miniature inverted-repeat transposable elements from genomic sequences. Nucleic Acids Res. 38:e199. https://doi.org/10.1093/nar/gkq862 Crossref, ISI, Google ScholarJurka, J., Kapitonov, V. V., Pavlicek, A., Klonowski, P., Kohany, O., and Walichiewicz, J. 2005. Repbase Update, a database of eukaryotic repetitive elements. Cytogenet. Genome Res. 110:462-467. https://doi.org/10.1159/000084979 Crossref, ISI, Google ScholarKanehisa, M., Goto, S., Kawashima, S., Okuno, Y., and Hattori, M. 2004. The KEGG resource for deciphering the genome. Nucleic Acids Res. 32:D277-D280. https://doi.org/10.1093/nar/gkh063 Crossref, ISI, Google ScholarKanzaki, S., and Nara, N. J. 2016. The origin and cultivar development of Japanese persimmon (Diospyros kaki Thunb.). J. Jpn. Soc. Food Sci. Technol. 63:328-330. https://doi.org/10.3136/nskkk.63.328 Crossref, ISI, Google ScholarKeilwagen, J., Wenk, M., Erickson, J. L., Schattat, M. H., Jan, G., and Frank, H. 2016. Using intron position conservation for homology-based gene prediction. Nucleic Acids Res. 44:e89. https://doi.org/10.1093/nar/gkw092 Crossref, ISI, Google ScholarKorf, I. 2004. Gene finding in novel genomes. BMC Bioinf. 5:59. https://doi.org/10.1186/1471-2105-5-59 Crossref, ISI, Google ScholarKumar, S., Stecher, G., and Tamura, K. 2016. MEGA7: Molecular Evolutionary Genetics Analysis version 7.0 for bigger datasets. Mol. Biol. Evol. 33:1870-1874. https://doi.org/10.1093/molbev/msw054 Crossref, ISI, Google ScholarLi, Q. L., Bu, J. Y., Shu, J., Yu, Z. H., Tang, L. H., Huang, S. P., Guo, T. X., Mo, J. Y., Luo, S. M., Solangi, G. S., and Hsiang, T. 2019. Colletotrichum species associated with mango in southern China. Sci. Rep. 9:18891. https://doi.org/10.1038/s41598-019-54809-4 Crossref, ISI, Google ScholarLowe, T. M., and Eddy, S. R. 1997. tRNAscan-SE: A program for improved detection of transfer RNA genes in genomic sequence. Nucleic Acids Res. 25:955-964. https://doi.org/10.1093/nar/25.5.955 Crossref, ISI, Google ScholarLuo, Z., and Wang, R. 2008. Persimmon in China: Domestication and traditional utilizations of genetic resources. Adv. Hortic. Sci. 22:239-243. Google ScholarMajoros, W. H., Pertea, M., and Salzberg, S. L. 2004. TigrScan and GlimmerHMM: Two open source ab initio eukaryotic gene-finders. Bioinformatics 20:2878-2879. https://doi.org/10.1093/bioinformatics/bth315 Crossref, ISI, Google ScholarManni, M., Berkeley, M. R., Seppey, M., Simão, F. A., and Zdobnov, E. M. 2021. BUSCO update: Novel and streamlined workflows along with broader and deeper phylogenetic coverage for scoring of eukaryotic, prokaryotic, and viral genomes. Mol. Biol. Evol. 38:4647-4654. https://doi.org/10.1093/molbev/msab199 Crossref, ISI, Google ScholarNawrocki, E. P., Burge, S. W., Bateman, A., Bateman, A., Daub, J., Eberhardt, R. Y., Eddy, S. R., Floden, E. W., Gardner, P. P., Jones, T. A., Tate, J., and Finn, R. D. 2014. Rfam 12.0: Updates to the RNA families database. Nucleic Acids Res. 43:D130-D137. https://doi.org/10.1093/nar/gku1063 Crossref, ISI, Google ScholarNawrocki, E. P., and Eddy, S. R. 2013. Infernal 1.1: 100-Fold faster RNA homology searches. Bioinformatics 29:2933-2935. https://doi.org/10.1093/bioinformatics/btt509 Crossref, ISI, Google ScholarPetersen, T. N., Brunak, S., von Heijne, G., and Nielsen, H. 2011. SignalP 4.0: Discriminating signal peptides from transmembrane regions. Nat. Methods 8:785-786. https://doi.org/10.1038/nmeth.1701 Crossref, ISI, Google ScholarPrice, A. L., Jones, N. C., and Pevzner, P. A. 2005. De novo identification of repeat families in large genomes. Bioinformatics 21:i351-i358. https://doi.org/10.1093/bioinformatics/bti1018 Crossref, ISI, Google ScholarSaier, M. H., Tran, C. V., and Barabote, R. D. 2006. TCDB: The Transporter Classification Database for membrane transport protein analyses and information. Nucleic Acids Res. 34:D181-D186. https://doi.org/10.1093/nar/gkj001 Crossref, ISI, Google ScholarShe, R., Chu, J. S. C., Wang, K., Pei, J., and Chen, N. S. 2009. GenBlastA: Enabling BLAST to identify homologous gene sequences. Genome Res. 19:143-149. https://doi.org/10.1101/gr.082081.108 Crossref, ISI, Google ScholarSmit, A. F. A., Hubley, R., and Green, P. 2013-2015. RepeatMasker Open-4.0. http://www.repeatmasker.org Google ScholarSperschneider, J., Gardiner, D. M., Dodds, P. N., Tini, F., Covarelli, L., Singh, K. B., Manners, J. M., and Taylor, J. M. 2015. EffectorP: Predicting fungal effector proteins from secretomes using machine learning. New Phytol. 210:743-761. https://doi.org/10.1111/nph.13794 Crossref, ISI, Google ScholarStanke, M., and Waack, S. 2003. Gene prediction with a hidden Markov model and a new intron submodel. Bioinformatics 19:ii215-ii225. https://doi.org/10.1093/bioinformatics/btg1080 Crossref, ISI, Google ScholarTalhinhas, P., and Baroncelli, R. 2021. Colletotrichum species and complexes: Geographic distribution, host range and conservation status. Fungal Divers. 110:109-198. https://doi.org/10.1007/s13225-021-00491-9 Crossref, ISI, Google ScholarTatusov, R. L., Galperin, M. Y., Natale, D. A., and Koonin, E. V. 2000. The COG database: A tool for genome-scale analysis of protein functions and evolution. Nucleic Acids Res. 28:33-36. https://doi.org/10.1093/nar/28.1.33 Crossref, ISI, Google ScholarThan, P. P., Prihastuti, H., Phoulivong, S., Taylor, P. W. J., and Hyde, K. D. 2008. Chilli anthracnose disease caused by Colletotrichum species. J. Zhejiang Univ. Sci. B 9:764-778. https://doi.org/10.1631/jzus.B0860007 Crossref, ISI, Google ScholarWeir, B. S., and Johnston, P. R. 2010. Characterisation and neo-typification of Gloeosporium kaki Hori as Colletotrichum horii nom. nov. Mycotaxon 111:209-219. https://doi.org/10.5248/111.209 Crossref, ISI, Google ScholarWicker, T., Sabot, F., Hua-Van, A., Bennetzen, J. L., Capy, P., Chalhoub, B., Flavell, A., Leroy, P., Morgante, M., Panaud, O., Paux, E., SanMiguel, P., and Schulman, A. H. 2007. A unified classification system for eukaryotic transposable elements. Nat. Rev. Genet. 8:973-982. https://doi.org/10.1038/nrg2165 Crossref, ISI, Google ScholarWinnenburg, R., Baldwin, T. K., Urban, M., Rawlings, C., Köhler, J., and Hammond-Kosack, K. E. 2006. PHI-base: A new database for pathogen host interactions. Nucleic Acids Res. 34:D459-D464. https://doi.org/10.1093/nar/gkj047 Crossref, ISI, Google ScholarXiao, C. L., Mackenzie, S. J., and Legard, D. E. 2004. Genetic and pathogenic analyses of Colletotrichum gloeosporioides from strawberry and noncultivated hosts. Phytopathology 94:446-453. https://doi.org/10.1094/PHYTO.2004.94.5.446 Link, ISI, Google ScholarXie, L., Zhang, J. Z., Cai, L., and Hyde, K. D. 2010. Biology of Colletotrichum horii, the causal agent of persimmon anthracnose. Mycology 1:242-253. https://doi.org/10.1080/21501203.2010.526644 Crossref, Google ScholarXu, Z., and Wang, H. 2007. LTR_FINDER: An efficient tool for the prediction of full-length LTR retrotransposons. Nucleic Acids Res. 35:W265-W268. https://doi.org/10.1093/nar/gkm286 Crossref, ISI, Google ScholarZhang, J. Z., and Xu, T. 2003. Various stages and amount of Colletotrichum gloeosporiodes on overwintering twigs of persimmon. J. Plant Prot. 30:437-438. Google ScholarZhang, J. Z., and Xu, T. 2005. Cytological characteristics of the infection in different species, varieties and organs of persimmon by Colletotrichum gloeosporioides. Mycosystema 24:116-122. Google ScholarFunding: This research was supported by National Key Research and Development Program of China (2019YFD1000600) and Special Project of Science and Technology Innovation Plan of Shaanxi Academy of Forestry (SXLK2020-0212).The author(s) declare no conflict of interest.DetailsFiguresLiterature CitedRelated Vol. 106, No. 3 March 2022SubscribeISSN:0191-2917e-ISSN:1943-7692 Download Metrics Downloaded 638 times Article History Issue Date: 30 Mar 2022Published: 8 Mar 2022Accepted: 2 Jan 2022 Pages: 1052-1055 Information© 2022 The American Phytopathological SocietyFundingNational Key Research and Development ProgramGrant/Award Number: 2019YFD1000600Special Project of Science and Technology Innovation Plan of Shaanxi Academy of ForestryGrant/Award Number: SXLK2020-0212KeywordsColletotrichum horiidisease managementfungal pathogengenomepersimmontree fruitsThe author(s) declare no conflict of interest.PDF download
MoreTranslated text
Key words
Colletotrichum horii, disease management, fungal pathogen, genome, persimmon, tree fruits
AI Read Science
Must-Reading Tree
Example
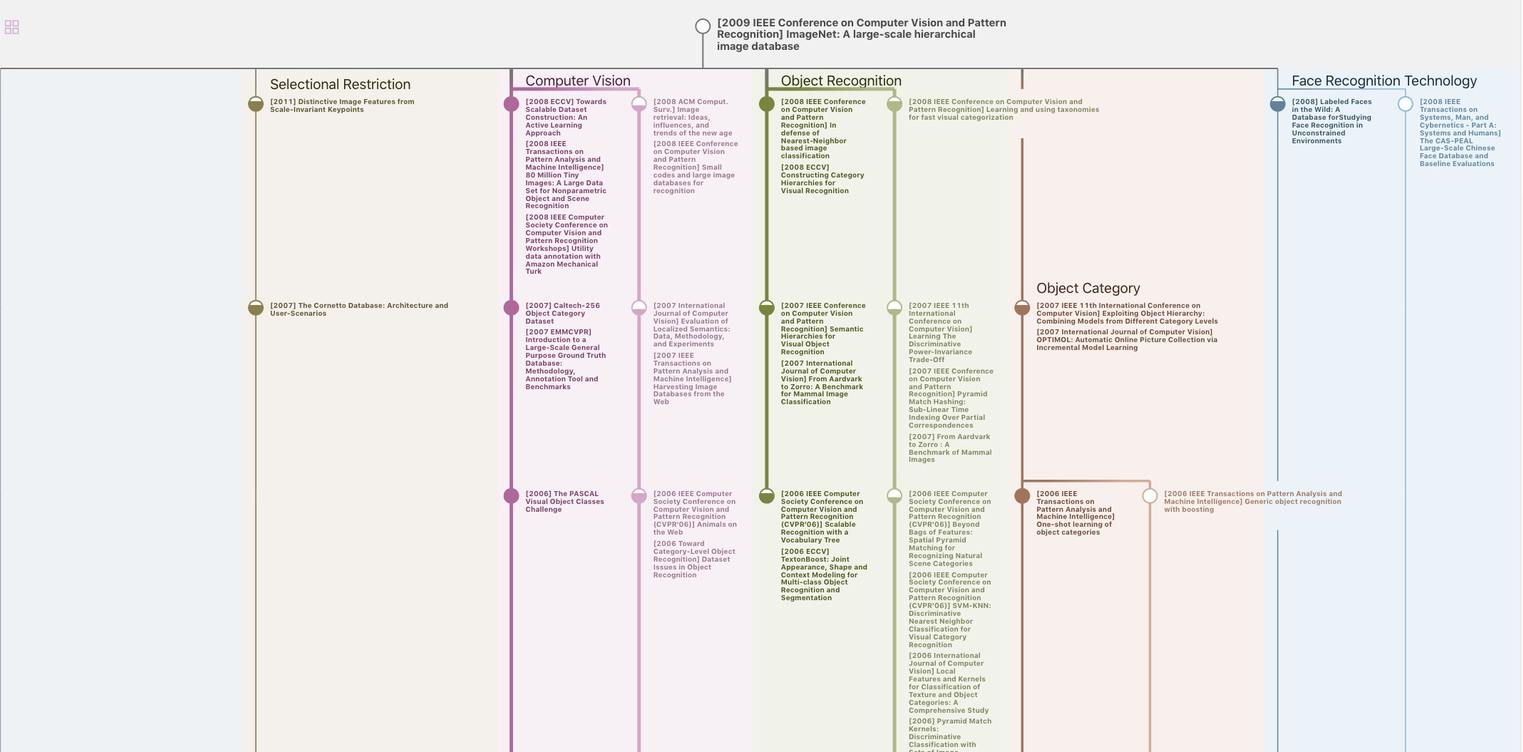
Generate MRT to find the research sequence of this paper
Chat Paper
Summary is being generated by the instructions you defined