Turbulence theories and statistical closure approaches
PHYSICS REPORTS-REVIEW SECTION OF PHYSICS LETTERS(2021)
Abstract
When discussing research in physics and in science more generally, it is common to ascribe equal importance to the three components of the scientific trinity: theoretical, experimental, and computational studies. This review will explore the future of modern turbulence theory by tracing its history, which began in earnest with Kolmogorov's 1941 analysis of turbulence cascade and inertial range [A.N. Kolmogorov, Dokl. Akad. Nauk SSSR, 30, 299, (1941); 32, 19, (1941)]. The 80th Anniversary of Kolmogorov's landmark study is a welcome opportunity to survey the achievements and evaluate the future of the theoretical approach of turbulence research. Over the years, turbulence theories have been critically important in laying the foundation of our understanding of the nature of turbulent flows. In particular, the Direct Interaction Approximation (DIA) [R.H. Kraichnan, J. Fluid Mech., 5, 497 (1959)] and its subsequent development, known as the statistical closure approach, can be identified as perhaps the most profound single advancement. The remarkable success of the statistical closure has furnished a platform to study such essential concepts as the energy transfer process and interacting scales, and the roles of the straining and sweeping motions. More recently, the quasi-Lagrangian formulation of V. L'vov & I. Procaccia and Kraichnan's solvable passive scalar model provided powerful ways to explore another fundamental aspect of turbulent flows, the phenomena of intermittency, and the associated anomalous scaling exponents. In the meantime, the theory of fluid equilibria has been developed to describe the large-scale structures that can emerge from turbulent cascades of two-dimensional and geophysical flows at a later time. And yet, despite all these successes, analytical treatments suffer from mathematical complexities. As a result, the utility of theoretical approaches has been limited to relatively idealized flows. On the other hand, in recent decades, computational abilities and experimental facilities have reached an unprecedented scale. Looking beyond the horizon, the imminent deployment of exascale supercomputers will generate complete datasets of the entire flow field of key benchmark flows, allowing researchers to extract additional measurements concerning fully developed, complex turbulent flow fields far beyond those available from the statistical closure theories. Some other developments that could potentially influence the future course of turbulence theories include the advancement of machine learning, artificial intelligence, and data science; likely disruptions arising from the advent of quantum computation; and the increasingly prominent role of turbulence research in providing more accurate climate scientific data. Turbulence theorists can leverage these developments by asking the right questions and developing advanced, sophisticated frameworks that will be able to predict and correlate vast amounts of data from the other two components of the trinity. (C) 2021 Elsevier B.V. All rights reserved.
MoreTranslated text
Key words
Turbulence theories,Statistical closure approaches,Energy transfer,Interacting scales,Straining and sweeping motions,Anisotropy,Intermittency,Anomalous scaling laws,Turbulence experiments,Theory of fluid equilibria,Geophysical turbulence,Plasma and magnetohydrodynamics (MHD) turbulence,Rotating and unstable stratified flows,Rayleigh-Taylor and Richtmyer-Meshkov instabilities,Turbulence modeling,Direct numerical simulation,Large eddy simulation,Exascale computer,Machine learning,Artificial intelligence,Data science,Quantum computing,Climate prediction
AI Read Science
Must-Reading Tree
Example
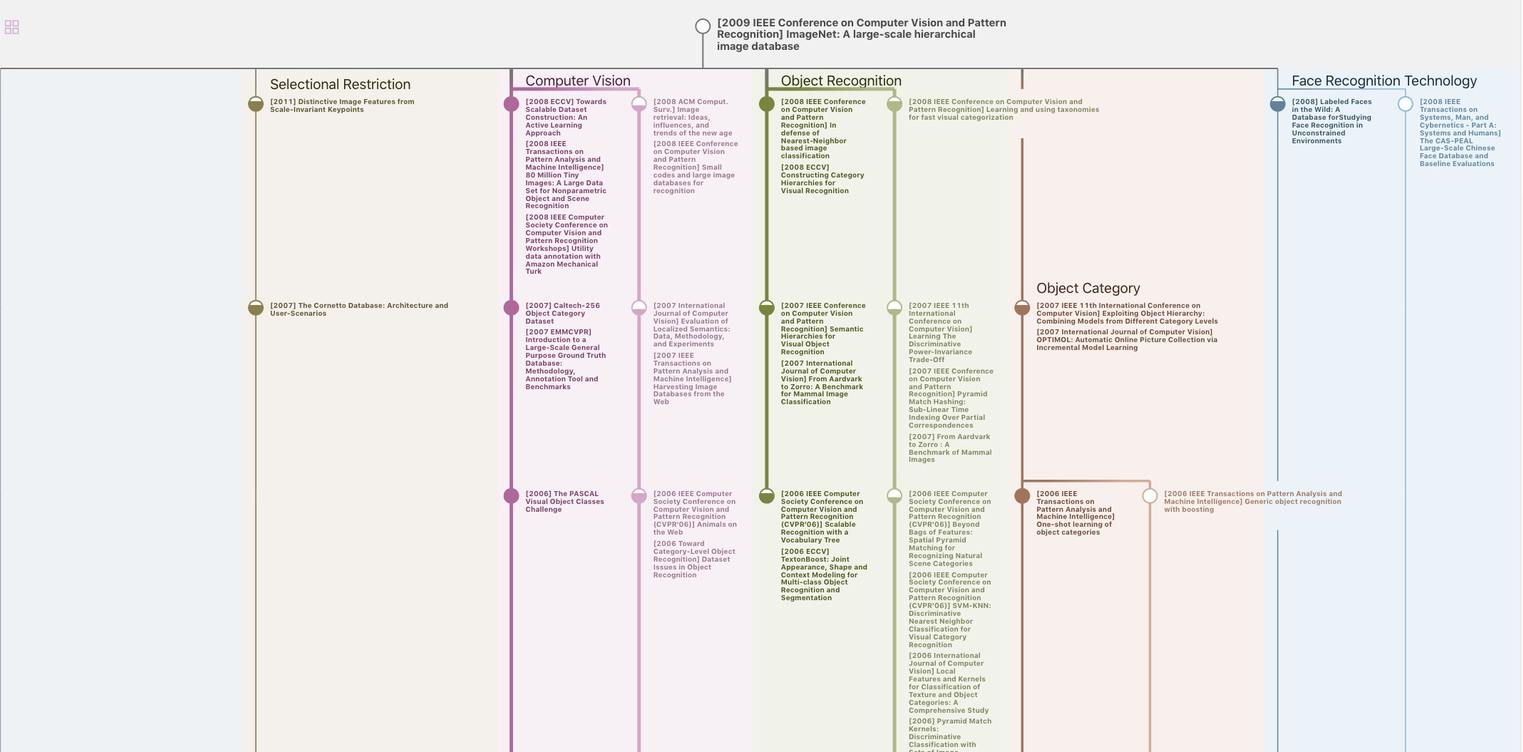
Generate MRT to find the research sequence of this paper
Chat Paper
Summary is being generated by the instructions you defined