(Invited) Photocatalytic Water Splitting Using SWCNT/TiO2 Nanohybrids
ECS Meeting Abstracts(2021)
Abstract
Water splitting photocatalysts based on semiconducting single-walled carbon nanotubes (s-SWCNTs) have been attracted much attention from the viewpoint of solar fuel production.[1-7] Since s-SWCNTs have chirality dependent bandgap covering a majority of the solar spectrum (400 – 2000 nm), s-SWCNTs are expected for an efficient solar-light absorber.[8] However, upon photoirradiation, the large exciton binding energy in s-SWCNTs prevents mobile carrier generation. Then, in order to make a particulate photocatalyst consisting of s-SWCNTs, an electron-extracting material is necessary. In this context, we have developed CNT-photocatalysts that contain a s-SWCNT/C60 coaxial heterojunction fabricated by a physical modification of s-SWCNTs using fullerodendron.[4] Here, the C60 acts as the electron-extracting material to generate a charge-separated state, s-SWCNT•+/C60 •–, followed by electron transfer to a co-catalyst that produces hydrogen from water. Z-scheme photocatalyst system employing BiVO4 as an oxygen evolution photocatalyst (OEP) and s-SWCNT/C60 coaxial hybrids as a hydrogen evolution photocatalyst (HEP) produced H2 and O2 from water, of which solar-to-hydrogen conversion efficiency (STH) was 0.089%.[7] We conducted an H2 evolution reaction using s-SWCNT/C60/Ru(III) upon monochromatic irradiation at 570, 650, and 680 nm, which are E22 absorption of (6,5), (7,5), and (8,3)tubes, respectively, and estimated the external quantum yields to be 0.22% for (6,5), 0.16% for (7,5), and 0.70% for (8,3). These EQY values were considerably lower than that using 1000-nm-light (E11 absorption of (6,5) and (8,3)tubes) irradiation, 12.8%.[7] The result might be attributed to branching pathways from the higher exciton (E22 excitation) to other than the lower exciton (E11 excitation). Blackburn and co-workers reported such a branching ratio is crucial for the PL quantum yield of s-SWCNTs.[9] Cui and co-workers describe E22 excitation makes interfacial electrotransfer inhibited in the (6,5)tube/C60 heterojunction, although ultrafast electron transfer process can be seen in E11 excitation by a DFT-based nonadiabatic dynamics simulation.[10] In this context, direct electron extraction from E22 excitation, which is faster than other branching pathways, is expected to improve the photocatalytic activity of CNT-photocatalysts. Recently, Blackburn reported higher-energy second excitonic s-SWCNT transitions produce more photocurrent by the use of submonolayer coverages of s-SWCNTs on single crystal TiO2 electrode, demonstrating carrier injection rates are competitive with fast hot-exciton relaxation processes.[11] These backgrounds prompt us to investigate a CNT-photocatalyst containing the s-SWCNT/TiO2 heterojunction. This paper describes fabrication of s-SWCNT/TiO2/Pt nanohybrids and their photocatalytic activity for H2 evolution from water. Interestingly, their EQYs of H2 evolution reaction using E22 excitation, 12% for (6,5)tube/TiO2/Pt and 43% for (8,3)tube/TiO2/Pt is much higher than those of (6,5)tube/C60/Ru(III) and (8,3)tube/C60/Ru(III), 0.22% and 0.70%. References [1] T. Tajima, W. Sakata, T. Wada, A. Tsutsui, S. Nishimoto, M. Miyake, Y. Takaguchi, Adv. Mater. 2011, 23, 5750-5754. [2] Y. Sasada, T. Tajima, T. Wada, T. Uchida, M. Nishi, T. Ohkubo, Y. Takaguchi, New J. Chem. 2013, 37, 4214-4219. [3] K. Kurniawan, T. Tajima, Y. Kubo, H. Miyake, W. Kurashige, Y. Negishi, Y. Takaguchi, RSC Adv. 2017, 7, 31767-31770. [4] N. Murakami, Y. Tango, H. Miyake, T. Tajima, Y. Nishina, W. Kurashige, Y. Negishi, Y. Takaguchi, Sci. Rep. 2017, 7, 43445: 1-7. [5] N. Murakami, H. Miyake, T. Tajima, K. Nishikawa, R. Hirayama, Y. Takaguchi, J. Am. Chem. Soc. 2018, 140, 3821-3824. [6] K. Ishimoto, T. Tajima, H. Miyake, M. Yamagami, W. Kurashige, Y. Negishi, Y. Takaguchi, Chem. Commun. 2018, 54, 393-396. [7] T. Izawa, V. Kalousek, D. Miyamoto, N. Murakami, H. Miyake, T. Tajima, W. Kurashige, Y. Negishi, K. Ikeue, T. Ohkubo, Y. Takaguchi, Chem. Lett. 2019, 48, 410-413. [8] M. Pfohl, D. D. Tune, A. Graf, J. Zaumseil, R. Krupke, B. S. Flavel, ACS Omega 2017, 2, 1163-1171. [9] J. L. Blackburn, T. J. McDonald, W. K. Metzger, C. Engtrakul, G. Rumbles, M. J. Heben, Nano Lett. 2008, 8, 1047-1054. [10] J.-J. Yang, Z.-W. Li, X.-Y. Liu, W.-H. Fanga, G. Cui, Phys. Chem. Chem. Phys. 2020, 22, 19542-19548. [11] L. Kubie, K. J. Watkins, R. Ihly, H. V. Wladkowski, J. L. Blackburn, W. D. Rice, B. A. Parkinson, J. Phys. Chem. Lett. 2018, 9, 4841-4847.
MoreTranslated text
Key words
Water Splitting,Photocatalysts,Selective Hydrogenation
AI Read Science
Must-Reading Tree
Example
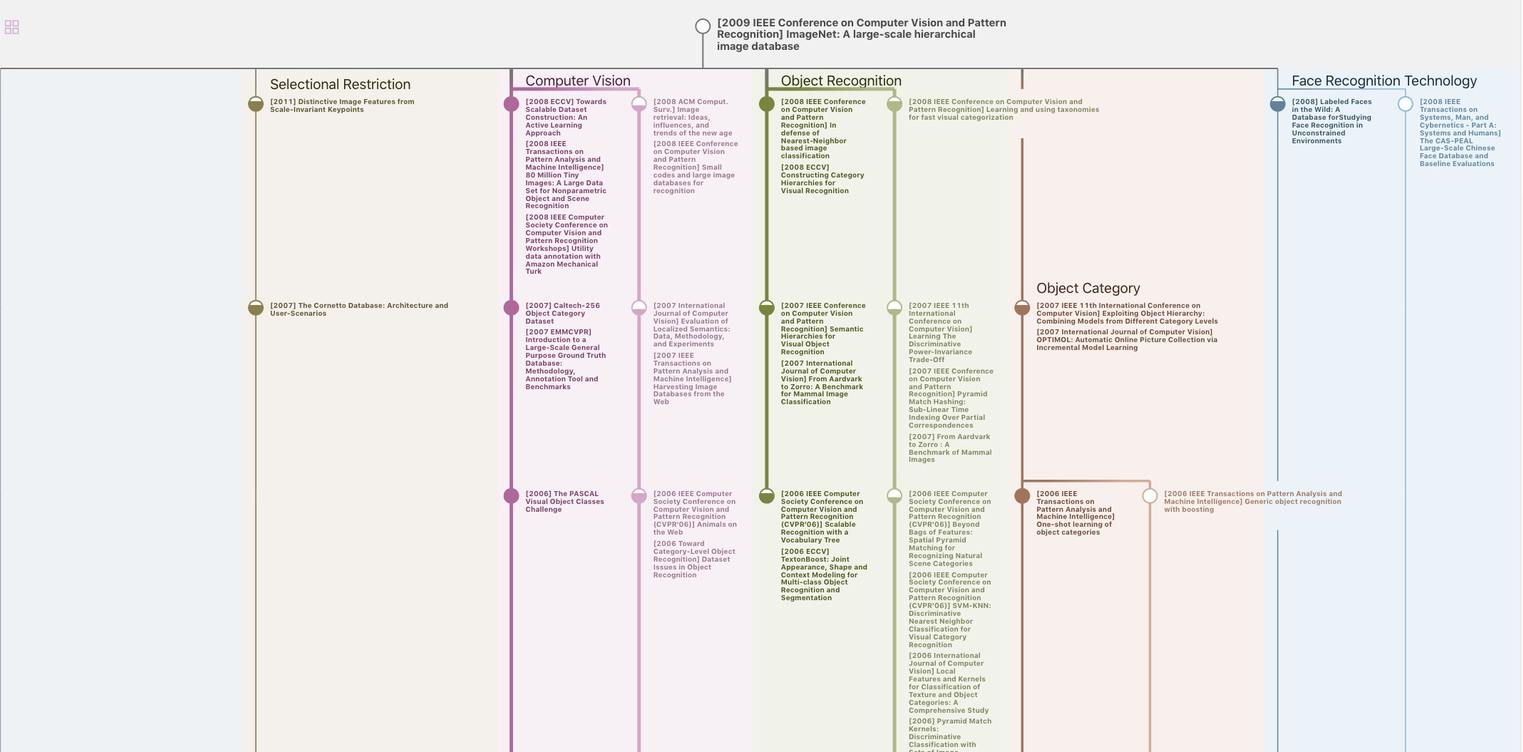
Generate MRT to find the research sequence of this paper
Chat Paper
Summary is being generated by the instructions you defined