Conformational dynamics of the
ABC
transporter McjD seen by single‐molecule
FRET
The EMBO Journal(2018)
摘要
Article20 September 2018Open Access Transparent process Conformational dynamics of the ABC transporter McjD seen by single-molecule FRET Florence Husada Florence Husada Molecular Microscopy Research Group, Zernike Institute for Advanced Materials, University of Groningen, Groningen, The Netherlands Search for more papers by this author Kiran Bountra Kiran Bountra Department of Life Sciences, Imperial College London, London, UK Rutherford Appleton Laboratory, Research Complex at Harwell, Didcot, UK Search for more papers by this author Konstantinos Tassis Konstantinos Tassis Molecular Microscopy Research Group, Zernike Institute for Advanced Materials, University of Groningen, Groningen, The Netherlands Search for more papers by this author Marijn de Boer Marijn de Boer Molecular Microscopy Research Group, Zernike Institute for Advanced Materials, University of Groningen, Groningen, The Netherlands Search for more papers by this author Maria Romano Maria Romano Department of Life Sciences, Imperial College London, London, UK Rutherford Appleton Laboratory, Research Complex at Harwell, Didcot, UK Search for more papers by this author Sylvie Rebuffat Sylvie Rebuffat Communication Molecules and Adaptation of Microorganisms Laboratory, (MCAM, UMR 7245 CNRS-MNHN), Muséum National d'Histoire Naturelle, Centre National de la Recherche Scientifique, Sorbonne Universités, Paris, France Search for more papers by this author Konstantinos Beis Corresponding Author Konstantinos Beis [email protected] orcid.org/0000-0001-5727-4721 Department of Life Sciences, Imperial College London, London, UK Rutherford Appleton Laboratory, Research Complex at Harwell, Didcot, UK Search for more papers by this author Thorben Cordes Corresponding Author Thorben Cordes [email protected] orcid.org/0000-0002-8598-5499 Molecular Microscopy Research Group, Zernike Institute for Advanced Materials, University of Groningen, Groningen, The Netherlands Physical and Synthetic Biology, Faculty of Biology, Ludwig-Maximilians-Universität München, Planegg-Martinsried, Germany Search for more papers by this author Florence Husada Florence Husada Molecular Microscopy Research Group, Zernike Institute for Advanced Materials, University of Groningen, Groningen, The Netherlands Search for more papers by this author Kiran Bountra Kiran Bountra Department of Life Sciences, Imperial College London, London, UK Rutherford Appleton Laboratory, Research Complex at Harwell, Didcot, UK Search for more papers by this author Konstantinos Tassis Konstantinos Tassis Molecular Microscopy Research Group, Zernike Institute for Advanced Materials, University of Groningen, Groningen, The Netherlands Search for more papers by this author Marijn de Boer Marijn de Boer Molecular Microscopy Research Group, Zernike Institute for Advanced Materials, University of Groningen, Groningen, The Netherlands Search for more papers by this author Maria Romano Maria Romano Department of Life Sciences, Imperial College London, London, UK Rutherford Appleton Laboratory, Research Complex at Harwell, Didcot, UK Search for more papers by this author Sylvie Rebuffat Sylvie Rebuffat Communication Molecules and Adaptation of Microorganisms Laboratory, (MCAM, UMR 7245 CNRS-MNHN), Muséum National d'Histoire Naturelle, Centre National de la Recherche Scientifique, Sorbonne Universités, Paris, France Search for more papers by this author Konstantinos Beis Corresponding Author Konstantinos Beis [email protected] orcid.org/0000-0001-5727-4721 Department of Life Sciences, Imperial College London, London, UK Rutherford Appleton Laboratory, Research Complex at Harwell, Didcot, UK Search for more papers by this author Thorben Cordes Corresponding Author Thorben Cordes [email protected] orcid.org/0000-0002-8598-5499 Molecular Microscopy Research Group, Zernike Institute for Advanced Materials, University of Groningen, Groningen, The Netherlands Physical and Synthetic Biology, Faculty of Biology, Ludwig-Maximilians-Universität München, Planegg-Martinsried, Germany Search for more papers by this author Author Information Florence Husada1,‡, Kiran Bountra2,3,‡, Konstantinos Tassis1, Marijn Boer1, Maria Romano2,3, Sylvie Rebuffat4, Konstantinos Beis *,2,3 and Thorben Cordes *,1,5 1Molecular Microscopy Research Group, Zernike Institute for Advanced Materials, University of Groningen, Groningen, The Netherlands 2Department of Life Sciences, Imperial College London, London, UK 3Rutherford Appleton Laboratory, Research Complex at Harwell, Didcot, UK 4Communication Molecules and Adaptation of Microorganisms Laboratory, (MCAM, UMR 7245 CNRS-MNHN), Muséum National d'Histoire Naturelle, Centre National de la Recherche Scientifique, Sorbonne Universités, Paris, France 5Physical and Synthetic Biology, Faculty of Biology, Ludwig-Maximilians-Universität München, Planegg-Martinsried, Germany ‡These authors contributed equally to this work *Corresponding author. Tel: +44 1235 567809; E-mail: [email protected] *Corresponding author. Tel: +49 89 2180 74623; E-mail: [email protected] The EMBO Journal (2018)37:e100056https://doi.org/10.15252/embj.2018100056 PDFDownload PDF of article text and main figures. Peer ReviewDownload a summary of the editorial decision process including editorial decision letters, reviewer comments and author responses to feedback. ToolsAdd to favoritesDownload CitationsTrack CitationsPermissions ShareFacebookTwitterLinked InMendeleyWechatReddit Figures & Info Abstract ABC transporters utilize ATP for export processes to provide cellular resistance against toxins, antibiotics, and harmful metabolites in eukaryotes and prokaryotes. Based on static structure snapshots, it is believed that they use an alternating access mechanism, which couples conformational changes to ATP binding (outward-open conformation) and hydrolysis (inward-open) for unidirectional transport driven by ATP. Here, we analyzed the conformational states and dynamics of the antibacterial peptide exporter McjD from Escherichia coli using single-molecule Förster resonance energy transfer (smFRET). For the first time, we established smFRET for an ABC exporter in a native-like lipid environment and directly monitor conformational dynamics in both the transmembrane- (TMD) and nucleotide-binding domains (NBD). With this, we unravel the ligand dependences that drive conformational changes in both domains. Furthermore, we observe intrinsic conformational dynamics in the absence of ATP and ligand in the NBDs. ATP binding and hydrolysis on the other hand can be observed via NBD conformational dynamics. We believe that the progress made here in combination with future studies will facilitate full understanding of ABC transport cycles. Synopsis ABC transporters facilitate export of toxic compounds from eukaryotic and bacterial cells in an ATP-dependent manner. While structural studies have increased our understanding of ABC transporter organization, the application of single-molecule FRET provides the first detailed view of the dynamics associated with substrate export. Single-molecule FRET of ABC transporter McjD reveals conformational dynamics associated with ATP and substrate binding. The ligand-binding site in the transmembrane domain (TMD) is occluded in the apo form but opens up in the presence of ATP and the antibacterial peptide MccJ25. Intrinsic conformational dynamics in the absence of ATP and ligand are observed in the Nucleotide Binding Domain (NBD) but not in the TMDs. ATP binding is coupled to conformational dynamics in the NBDs while both ATP and peptide MccJ25 are necessary to drive conformational changes in the TMDs. Introduction ABC transporters are essential membrane proteins found in both eukaryotic and bacterial cells that facilitate the uphill transport of ions and chemically diverse compounds in an ATP-dependent manner (Holland et al, 2003). They are involved in numerous cellular processes including nutrient import, metal homeostasis, detoxification, and antigen processing. The ABC exporter family plays a major role for the extrusion of toxic compounds and has relevance for human diseases, tumor resistance, and bacterial virulence. All ABC transporters share a common architecture consisting of a transmembrane domain (TMD) for substrate recognition and transport, and a nucleotide-binding domain (NBD) that converts the chemical energy of ATP into conformational changes for transport (Beis, 2015). The structures of several homodimeric (Dawson & Locher, 2006; Ward et al, 2007; Perez et al, 2015) and heterodimeric ABC transporters (Hohl et al, 2012; Noll et al, 2017) revealed distinct conformations and suggest, in combination with biophysical studies (e.g., EPR and NMR; Dong et al, 2005; Zou et al, 2009; Bountra et al, 2017; Timachi et al, 2017; Barth et al, 2018), that they undergo large conformational changes during transport. Their complex architecture is, however, a fundamental hurdle to fully understand the coupling between conformational changes, substrate binding, ATP binding and hydrolysis, and transport. Such detailed mechanistic models of transport would require not only the knowledge of conformational states from static snapshots, but also their interconversion dynamics. Based on the available structural, biophysical, and biochemical data for exporters, two transport mechanisms have been proposed, the alternating access mechanism (Dawson & Locher, 2006; Ward et al, 2007; Choudhury et al, 2014; Bountra et al, 2017) and the outward-only mechanism (Perez et al, 2015). ABC exporters that use the alternating access mechanism switch between inward- and outward-facing states with transmembrane helices intertwining, which exposes the ligand binding site alternatively to the inside or outside of the membrane, a process that is believed to be coupled to ATP binding to hydrolysis. The outward-only mechanism was proposed in light of the structural and functional data of the lipid-linked oligosaccharide flippase PglK from Campylobacter jejuni (Perez et al, 2015); here the inward-facing conformation is not required for substrate translocation since it can be directly recruited from the membrane and ATP hydrolysis at the NBDs is transmitted to the TMD to drive substrate release. ABC transporters that utilize the alternating access mechanism undergo large conformational changes during the transport cycle suggested by crystal structures (Ward et al, 2007) and EPR-based Double Electron Electron Resonance (DEER) measurements (Dong et al, 2005; Borbat et al, 2007; Mishra et al, 2014) of the lipid A flippase MsbA from Escherichia coli. Although the DEER measurements proposed that ATP triggers the opening of the TMD to the periplasmic side for release of the substrate, it is unclear how precisely these changes are coupled to ATP binding and hydrolysis. It has been proposed that only homodimeric transporters employ this mechanism. Opening of the TMD of heterodimeric ABC transporters is coupled to ATP hydrolysis rather than binding due to the nature of their NBDs that contain a consensus and degenerate site for ATP hydrolysis (Mishra et al, 2014; Timachi et al, 2017; Barth et al, 2018). In contrary, we have previously shown that the antibacterial peptide ABC transporter McjD, which confers bacterial cells with self-immunity against the antibacterial peptide MccJ25, adopts distinct occluded conformations (Choudhury et al, 2014; Bountra et al, 2017) to other bacterial exporters including MsbA (Ward et al, 2007). The TMD is occluded to either side of the membrane in the presence or absence of nucleotides that was also supported by Pulsed Electron-Electron Double Resonance (PELDOR) measurements in bicelles (Bountra et al, 2017). Occluded conformations have also been reported for other ABC transporters (Lin et al, 2015; Perez et al, 2015; Morgan et al, 2017). We have biochemically shown that McjD adopts a transient outward-open conformation that it is probably not long-lived or well populated to be trapped by PELDOR (Bountra et al, 2017). Although both MsbA and McjD utilize the alternating access mechanism for export of their substrate, the overall mechanism is not fully conserved and requires further characterization. So far, however, all the conformations that have been reported for ABC transporters are static and do not provide insights into the conformational changes and dynamics that govern the movement of NBDs upon ATP binding and hydrolysis or that of the TMDs during substrate transport across the membrane bilayer. In recent years, it has, however, become apparent that transporters show conformational dynamics and that those have to be understood and characterized (Locher, 2016). For this, single-molecule Förster resonance energy transfer (smFRET; Lerner et al, 2018) has been used to monitor these conformational dynamics of transporters, e.g., the P-type Ca2+-ATPase (LMCA1) from Listeria monocytogenes (Dyla et al, 2017), secondary transporters such as the aspartate/Na+ symporter from Pyrococcus horikoshii (Akyuz et al, 2013), and the leucine/Na+ symporter LeuT from Aquifex aeolicus (Zhao et al, 2010). While there are also smFRET studies of ABC importers regarding the conformational dynamics of the substrate binding domains (SBDs; Gouridis et al, 2015) or interactions of SBD-TMD (Goudsmits et al, 2017a; Yang et al, 2018), there are no studies of conformational dynamics and crosstalk between TMDs and NBDs in ABC exporters in native-like lipid environment (Verhalen et al, 2012). Here, we have analyzed the antibacterial peptide exporter McjD from E. coli in proteoliposomes using smFRET via labeling of specific residues in the TMD and NBDs to monitor the conformational dynamics during the transport cycle. The developed assay has the potential to provide full understanding of the transport mechanism and can be used for both ABC importers and exporters. In this study, it was used to answer the following mechanistic questions regarding McjD: (i) How is ATP binding or hydrolysis coupled to the opening of the TMD? In McjD, crystal structures and PELDOR data in the presence of the ATP-analogue adenosine 5′-(β,γ-imido)triphosphate (AMPPNP), mimicking ATP binding, and ADP-vanadate (mimicking ATP hydrolysis) could not reveal an open cavity (Choudhury et al, 2014; Bountra et al, 2017). (ii) What are the intrinsic and ligand-induced conformational dynamics of McjD as a model system for ABC exporters and on what timescales do they occur? To answer these questions, we have studied McjD reconstituted in liposomes under equilibrium conditions to obtain a picture of the stable conformational states and non-equilibrium conditions to understand how conformational changes are triggered by substrates using smFRET. We demonstrate that the NBDs have intrinsic conformational dynamics in their apo state on the 100 ms timescale, while the TMDs remain static. ATP binding and hydrolysis on the other hand take much longer and it is observed via NBD conformational dynamics on the timescale of several seconds, which is a value compatible with reported biochemical data on McjD. Our assays also show that the TMD remains occluded in the presence of nucleotides and only opens when both the substrate MccJ25 and ATP are present. Our study represents major advances in using smFRET for mechanistic studies of ABC transporters in a native-like lipid environment. Furthermore, this is the first report showing that an ABC exporter requires both ATP and substrate binding to drive the formation of the outward-open conformation. Thus, we propose that opening of the McjD cavity is tightly coupled to both ATP and MccJ25 binding and present a refined model for the transport cycle. Results Single-molecule FRET monitors the conformational states of the ABC exporter McjD smFRET has emerged as a tool for investigating conformational dynamics of biomacromolecules over the past 20 years (Lerner et al, 2018). This approach relies on mapping a relevant reaction coordinate, i.e., for ABC transporters spatial separation of characteristic residues between TMD and NBD domains, and provides “low-resolution” structural information in the form of a distance. On the other hand, however, it is a very powerful technique to measure real-time conformational changes and dynamics unlike other biophysical techniques such as EPR, where the sample has to be frozen. In this paper, we have established smFRET to directly monitor the conformational states of ABC transporters. Our assay is tailored to monitor conformational states and dynamics during substrate export and relies on the use of single cysteines at the periplasmic side of the TMD, Y64C, and L67C (Fig 1A) and the cytosolic NBDs, native C547, of McjD (Fig 1A); all single mutants were introduced to cys-less McjD (via mutation of native cysteines to serine). Figure 1. Biochemical characterization of McjD and smFRET assay design Schematic representation of the smFRET assay using crystal structure snapshots of McjD. In the crystal structure, nucleotides and ATP-Mg are in orange sticks, and the peptide MccJ25 is in pink. Each half transporter is colored in dark and light gray. McjD is shown in a view along the plane of the membrane which is depicted in light gray. Colored balls show the position of the cysteines used for probing conformational changes. PBD codes for McjD in inward-occluded conformation are 5OFP (left; Bountra et al, 2017) and in the outward-occluded conformation are 4PL0 (right; Choudhury et al, 2014). Coomassie-stained SDS–PAGE of McjD-C547 reconstituted in liposomes. The amount reconstituted was evaluated against detergent-purified protein. Chromatogram of McjD-C547 (black) labeled with Alexa 555 (blue) and Alexa 647 (red)-maleimide fluorophore using a Superdex 200 size-exclusion column. The fraction containing the highest degree of labeling is marked by a square. The ATPase activity of all labeled variants C547, Y64C, and L67C was determined in liposomes and compared to wild-type, wt. All labeled McjD variants show basal- and ligand-induced ATPase activities in liposomes comparable to that of wild-type protein, indicating that labeling does not interfere with their activity. Error bars were calculated from two replicates from two independent reconstitutions (mean ± standard deviation, n = 2). The ruler character of FRET using the fluorophore pair Alexa555/Cy5 was supported by data of dsDNA in Fig EV1B, where different base-pair distances between both dyes correlate directly with the apparent FRET efficiency. Download figure Download PowerPoint It can be clearly seen that, due to the homodimeric nature of McjD, introduction of one cysteine results in the possibility to label the protein with two fluorophores. This fulfills the requirement of FRET to introduce both donor and acceptor fluorophore. Furthermore, structural analysis suggests that the apo conformation of McjD has distinct distances between the two labeled positions compared to the nucleotide-bound conformation for McjD C547 (but not for Y64C and L67C), a fact that should allow assessment of the biochemical and conformational states of McjD via smFRET. The FRET efficiency changes are related to the distance of the respective variants and thus indicate the changes that occur upon addition of ATP or the substrate MccJ25. For example, the crystal structure estimates that the nucleotide-free form of McjD should show a larger NBD separation (low FRET), while this should be shorter in the nucleotide-bound form (high FRET; Choudhury et al, 2014; Bountra et al, 2017). McjD was purified in detergent and reconstituted into proteoliposomes as previously described (Fig 1B; Choudhury et al, 2014; Bountra et al, 2017). For smFRET, McjD and variants were labeled stochastically with Alexa555 and Alexa647 (see “Materials and Methods”). The gel-filtration purification of donor–acceptor-labeled McjD is shown in Fig 1C. The detergent-solubilized protein runs as a mono-disperse peak with significant absorption at both the donor and acceptor wavelengths at 532 and 640 nm, respectively (Fig 1C). The labeling efficiency of the two fluorophores for McjD-C547 was determined to be ~60%. All variants were biochemically characterized using a previously published ATPase assay (Choudhury et al, 2014; Bountra et al, 2017). Neither the introduction of cysteines nor the addition of fluorescent labels affected the activity of the transporter in proteoliposomes; this is indicated by similar basal and ligand-induced ATPase activity compared to wild-type McjD (Fig 1D). All interpretations given below are supported by comparison of the observed E* values of McjD with respect to static dsDNA ruler structures (Fig EV1A and B) that we have previously described (Ploetz et al, 2016). Click here to expand this figure. Figure EV1. Static dsDNA control experiments Schematic representation of the smFRET assay using labeled double-stranded DNA with Alexa 555 and Cy5 fluorophores as indicated. Distance estimates can be derived by multiplication of 0.34 nm times the number of base pairs; these provide only an inaccurate approximation of the distance between donor and acceptor dye. Experimental data similar to those shown in Fig 2 are shown, which support the validity of experimental results and interpretations given in the main text. Download figure Download PowerPoint We first assessed the conformational states of McjD under equilibrium conditions in the detergent-solubilized state. We used three different labeling positions in McjD to monitor the conformational states of the periplasmic TMD region and the cytosolic ATPase domain (Figs 1A and 2A). Since detergent-solubilized McjD diffuses freely in solution, we used confocal microscopy with alternating laser excitation (ALEX) to relate FRET efficiency values of single McjD molecules to their respective conformational states (Fig 2B). From the available crystal structures, we extracted Ca-Ca distances between the two labeling positions. It is worthwhile to note that, in our smFRET assays, we do not determine absolute distances, but only use relative distance changes via the setup-dependent apparent FRET efficiency E*. The qualitative relation between E* and the Ca-Ca distances do, however, allow us to assess whether FRET experiments relate well to distances expected from the crystal structures. For the periplasmic TMD mutants, Y64C and L67C, the crystal structures predict Ca-Ca distances of 2.6 and 2.9 nm, respectively (without taking any fluorophore properties into account). The Förster radius of Alexa555/647 is R0 = 5.1 nm. At this distance, FRET efficiency is theoretically expected to be 0.5; interprobe distances above this value are thus expected to show smaller values of E*, while shorter distances are expected to show larger E* values. Since Ca-Ca distances of both TMD mutants are shorter than R0, we expect high-FRET efficiency values significantly above 0.5. The data are coherent with this expectation, based on crystal structure predictions as seen from Fig 2C. FRET distribution of apo-McjD center is around 0.95 for L67C and ~1.07 for Y64C (see Table 1 and Fig EV2 for mean FRET values). The distance extracted for C547, located at the NBDs, from the crystal structure of the apo-McjD is 4.8 nm, in agreement with the mean observed FRET efficiency of apo-McjD of ~0.31 when also considering added flexibility of the dyes by their linkers. Figure 2. Freely diffusing McjD in detergent studied by smFRET A, B. Schematic overview of McjD labeling schemes (A) and diffusion-based experiments (B) using confocal microscopy. Stochastic labeling is performed on the NBD (native cysteine C547) and TMD mutants (Y64C; L67C). C. Confocal single-molecule analysis with ALEX of labeled McjD in detergent under different conditions as indicated. D. Apparent ligand binding affinity values of McjD for AMPPNP (top), ATP (middle), and ADP-vanadate (bottom). Data points were obtained from the areas of fits from the E* histograms considering the ratio ligand-free/(ligand-free + ligand-bound) at the indicated substrate concentrations. Extraction of Kd values from a fit to the data is described in the Materials and Methods section. Data for detergent-solubilized McjD for additional biochemical conditions are shown in Fig EV2. Download figure Download PowerPoint Table 1. Mean apparent FRET values from a 1D Gaussian fit Detergent Liposomes L67C Y64C C547 L67C Y64C C547 Apo 0.95 1.07 0.31 0.69 0.81 & 0.60 0.32 ATP 0.95 1.01 0.56 n.d. n.d. 0.495 ATP + Vanadate n.d. n.d. 0.56 n.d. n.d. n.d. ATP + MccJ25 0.83 0.91 n.d. 0.51 0.82 & 0.52 n.d. ADP 0.95 0.99 0.31 n.d. n.d. n.d. ADP + Vanadate 0.95 0.99 0.30 & 0.56 n.d. n.d. n.d. Vanadate n.d. n.d. 0.31 n.d. n.d. n.d. AMPPNP 0.95 1.03 0.54 n.d. n.d. n.d. The table provides an overview of mean apparent FRET values E* (setup dependent) for different environmental conditions. It has to be noted that values in detergent were derived from a different microscope setup than for liposomes and can thus not be compared directly; n.d., not determined. Click here to expand this figure. Figure EV2. Additional data for detergent-solubilized McjD A, B. Confocal single-molecule analysis of labeled McjD and variants in detergent using ALEX. Mean values of ligand-free conditions are shown as a reference with a solid marker, while ligand-bound mean values (A) are shown dashed (compare Fig 2). (B) Mean values of ligand-free conditions are shown as a reference with a solid marker, while ligand-bound mean values are shown dashed. Note that the two panels cannot be compared directly due to slightly different microscope alignment. Download figure Download PowerPoint Having established that our smFRET assays are coherent with structural predictions, we tested conditions that mimic relevant stages of the ABC transport cycle. From diffusion-based experiments, these show the statistically relevant states and related conformations of McjD for the following: (i) ATP binding, hydrolysis, and turnover with/without substrate MccJ25; (ii) the pre-hydrolysis state using the ATP-analogue AMPPNP; (iii) the transition state during ATP hydrolysis with ATP-Vanadate; and finally (iv) the post-hydrolysis state using either ADP or ADP-vanadate (Figs 2 and EV2). For both TMD mutants, the addition of ATP, its non-hydrolyzable analogue AMPPNP, or transition-state mimic such as ATP-vanadate did not alter their conformational states (Fig 2C). Interestingly, the addition of MccJ25 and ATP triggered opening of the periplasmic gate as observed by a small but significant shift toward lower FRET efficiency values (Fig 2C). Strikingly, the observation of opening occurred under equilibrium conditions and indicates that the outward-open conformation is visited by McjD during the transport cycle and can be triggered by both ATP and the MccJ25 peptide. We observe a slightly smaller opening of the TMDs in the absence of nucleotides but the presence of MccJ25 that suggests the peptide can induce an open TMD during its rebinding (Fig EV2B). We have shown, however, that McjD transports MccJ25 in an ATP-dependent manner. We explain the observations by the fact that ABC exporters can transport their substrates bidirectionally, and this previously described effect manifests in a structural reorientation of the TMD and opening that is similar to that observed during transport with MccJ25 and ATP but only occurs at high concentrations of peptide via rebinding (Grossmann et al, 2014). The ABC transporter associated with antigen processing (TAP) has been shown to be unidirectional despite observation of rebinding of peptide, which is essential for its physiological function (Grossmann et al, 2014). On the contrary, the NBDs were very sensitive to ATP (and analogues) and McjD-C547 showed pronounced conformational changes upon ATP, AMPPNP, ATP-vanadate, and ADP-vanadate addition (Fig 2C). Whenever ATP is already present, MccJ25 did not alter the observed conformational state any further. The only conditions that were incompatible with conformational change are sole addition of either peptide, ADP, or vanadate alone (Tables 1 and EV1, Fig EV2). The conformational changes in McjD-C547 occur with apparent affinities in the 50–100 μM range for ATP, AMPPNP, and ADP-vanadate (Fig 2D), matching reported values for binding affinities of other ABC transporters (Siarheyeva & Sharom, 2009). The data show that binding of the nucleotides (ATP, AMPPNP, and ADP-vanadate) at the NBDs triggers conformational changes into their closed dimer form (Fig 2C). It is only after ATP hydrolysis (ADP-vanadate, Fig EV2; and ADP, Fig 2) that reopening of the NBDs is seen, which we believe is linked to a reset of the transporter. Altogether, the data based on detergent-solubilized McjD suggest an alternating access mechanism, where conformational changes are triggered by ATP (engagement of the NBDs from inward-open to outward-occluded) and substrate binding (opening of the TMD to outward-open). ATP hydrolysis seems to only reset the transporter since ATP binding is coupled to conformational changes in the NBDs and TMD. This interpretation is further supported by the fact that ADP binding does not induce conformational changes. The data also suggest tight coupling between ATP and MccJ25 binding and subsequent substrate transport. Since the detergent environment is, however, not physiologically relevant, we further verified these results (and their interpretations) with surface-immobilized liposome-reconstituted McjD. McjD displays similar conformational states and changes in detergent and proteoliposomes For studies of surface-immobilized liposomes containing one transporter, we used a low protein-to-lipid ratio of ~1:1,000 (w/w). To ve
更多查看译文
关键词
transporter mcjd,conformational dynamics
AI 理解论文
溯源树
样例
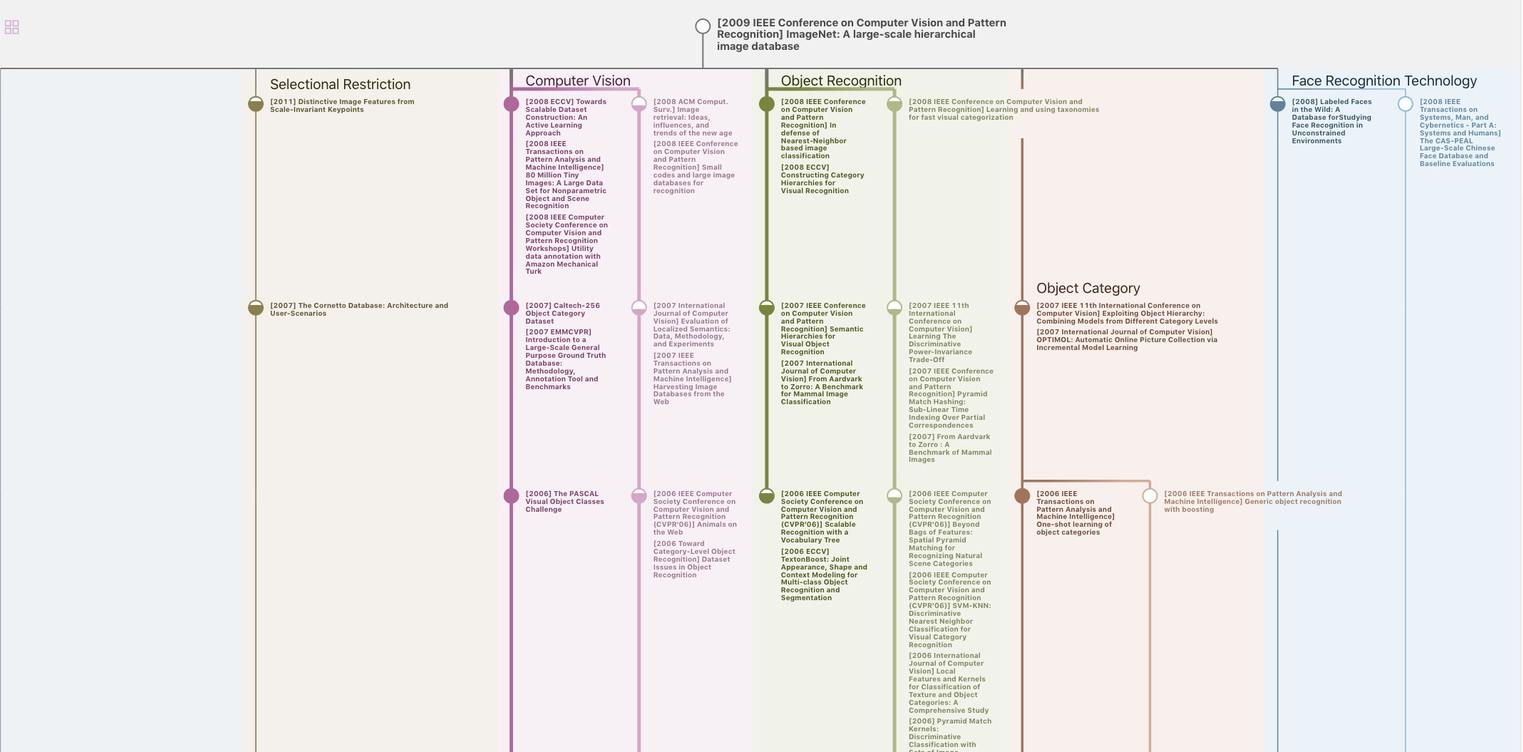
生成溯源树,研究论文发展脉络
Chat Paper
正在生成论文摘要