PTRN
‐1/
CAMSAP
promotes
CYK
‐1/formin‐dependent actin polymerization during endocytic recycling
The EMBO Journal(2018)
Abstract
Article22 March 2018free access Source DataTransparent process PTRN-1/CAMSAP promotes CYK-1/formin-dependent actin polymerization during endocytic recycling Ting Gong Department of Biochemistry and Molecular Biology, School of Basic Medicine and the Collaborative Innovation Center for Brain Science, Tongji Medical College, Huazhong University of Science and Technology, Wuhan, Hubei, China Search for more papers by this author Yanling Yan Department of Biochemistry and Molecular Biology, School of Basic Medicine and the Collaborative Innovation Center for Brain Science, Tongji Medical College, Huazhong University of Science and Technology, Wuhan, Hubei, China Search for more papers by this author Jing Zhang Department of Biochemistry and Molecular Biology, School of Basic Medicine and the Collaborative Innovation Center for Brain Science, Tongji Medical College, Huazhong University of Science and Technology, Wuhan, Hubei, China Search for more papers by this author Shuai Liu Department of Biochemistry and Molecular Biology, School of Basic Medicine and the Collaborative Innovation Center for Brain Science, Tongji Medical College, Huazhong University of Science and Technology, Wuhan, Hubei, China Search for more papers by this author Hang Liu Department of Biochemistry and Molecular Biology, School of Basic Medicine and the Collaborative Innovation Center for Brain Science, Tongji Medical College, Huazhong University of Science and Technology, Wuhan, Hubei, China Search for more papers by this author Jinghu Gao Department of Biochemistry and Molecular Biology, School of Basic Medicine and the Collaborative Innovation Center for Brain Science, Tongji Medical College, Huazhong University of Science and Technology, Wuhan, Hubei, China Search for more papers by this author Xin Zhou Department of Biochemistry and Molecular Biology, School of Basic Medicine and the Collaborative Innovation Center for Brain Science, Tongji Medical College, Huazhong University of Science and Technology, Wuhan, Hubei, China Search for more papers by this author Juan Chen Department of Biochemistry and Molecular Biology, School of Basic Medicine and the Collaborative Innovation Center for Brain Science, Tongji Medical College, Huazhong University of Science and Technology, Wuhan, Hubei, China Search for more papers by this author Anbing Shi Corresponding Author [email protected] orcid.org/0000-0003-1128-2026 Department of Biochemistry and Molecular Biology, School of Basic Medicine and the Collaborative Innovation Center for Brain Science, Tongji Medical College, Huazhong University of Science and Technology, Wuhan, Hubei, China Institute for Brain Research, Huazhong University of Science and Technology, Wuhan, Hubei, China Key Laboratory of Neurological Disease of National Education Ministry, Tongji Medical College, Huazhong University of Science and Technology, Wuhan, Hubei, China Search for more papers by this author Ting Gong Department of Biochemistry and Molecular Biology, School of Basic Medicine and the Collaborative Innovation Center for Brain Science, Tongji Medical College, Huazhong University of Science and Technology, Wuhan, Hubei, China Search for more papers by this author Yanling Yan Department of Biochemistry and Molecular Biology, School of Basic Medicine and the Collaborative Innovation Center for Brain Science, Tongji Medical College, Huazhong University of Science and Technology, Wuhan, Hubei, China Search for more papers by this author Jing Zhang Department of Biochemistry and Molecular Biology, School of Basic Medicine and the Collaborative Innovation Center for Brain Science, Tongji Medical College, Huazhong University of Science and Technology, Wuhan, Hubei, China Search for more papers by this author Shuai Liu Department of Biochemistry and Molecular Biology, School of Basic Medicine and the Collaborative Innovation Center for Brain Science, Tongji Medical College, Huazhong University of Science and Technology, Wuhan, Hubei, China Search for more papers by this author Hang Liu Department of Biochemistry and Molecular Biology, School of Basic Medicine and the Collaborative Innovation Center for Brain Science, Tongji Medical College, Huazhong University of Science and Technology, Wuhan, Hubei, China Search for more papers by this author Jinghu Gao Department of Biochemistry and Molecular Biology, School of Basic Medicine and the Collaborative Innovation Center for Brain Science, Tongji Medical College, Huazhong University of Science and Technology, Wuhan, Hubei, China Search for more papers by this author Xin Zhou Department of Biochemistry and Molecular Biology, School of Basic Medicine and the Collaborative Innovation Center for Brain Science, Tongji Medical College, Huazhong University of Science and Technology, Wuhan, Hubei, China Search for more papers by this author Juan Chen Department of Biochemistry and Molecular Biology, School of Basic Medicine and the Collaborative Innovation Center for Brain Science, Tongji Medical College, Huazhong University of Science and Technology, Wuhan, Hubei, China Search for more papers by this author Anbing Shi Corresponding Author [email protected] orcid.org/0000-0003-1128-2026 Department of Biochemistry and Molecular Biology, School of Basic Medicine and the Collaborative Innovation Center for Brain Science, Tongji Medical College, Huazhong University of Science and Technology, Wuhan, Hubei, China Institute for Brain Research, Huazhong University of Science and Technology, Wuhan, Hubei, China Key Laboratory of Neurological Disease of National Education Ministry, Tongji Medical College, Huazhong University of Science and Technology, Wuhan, Hubei, China Search for more papers by this author Author Information Ting Gong1,‡, Yanling Yan1,‡, Jing Zhang1, Shuai Liu1, Hang Liu1, Jinghu Gao1, Xin Zhou1, Juan Chen1 and Anbing Shi *,1,2,3 1Department of Biochemistry and Molecular Biology, School of Basic Medicine and the Collaborative Innovation Center for Brain Science, Tongji Medical College, Huazhong University of Science and Technology, Wuhan, Hubei, China 2Institute for Brain Research, Huazhong University of Science and Technology, Wuhan, Hubei, China 3Key Laboratory of Neurological Disease of National Education Ministry, Tongji Medical College, Huazhong University of Science and Technology, Wuhan, Hubei, China ‡These authors contributed equally to this work *Corresponding author. Tel: +86 139 8605 6760; E-mail: [email protected] EMBO J (2018)37:e98556https://doi.org/10.15252/embj.201798556 PDFDownload PDF of article text and main figures. Peer ReviewDownload a summary of the editorial decision process including editorial decision letters, reviewer comments and author responses to feedback. ToolsAdd to favoritesDownload CitationsTrack CitationsPermissions ShareFacebookTwitterLinked InMendeleyWechatReddit Figures & Info Abstract Cargo sorting and membrane carrier initiation in recycling endosomes require appropriately coordinated actin dynamics. However, the mechanism underlying the regulation of actin organization during recycling transport remains elusive. Here we report that the loss of PTRN-1/CAMSAP stalled actin exchange and diminished the cytosolic actin structures. Furthermore, we found that PTRN-1 is required for the recycling of clathrin-independent cargo hTAC-GFP. The N-terminal calponin homology (CH) domain and central coiled-coils (CC) region of PTRN-1 can synergistically sustain the flow of hTAC-GFP. We identified CYK-1/formin as a binding partner of PTRN-1. The N-terminal GTPase-binding domain (GBD) of CYK-1 serves as the binding interface for the PTRN-1 CH domain. The presence of the PTRN-1 CH domain promoted CYK-1-mediated actin polymerization, which suggests that the PTRN-1-CH:CYK-1-GBD interaction efficiently relieves autoinhibitory interactions within CYK-1. As expected, the overexpression of the CYK-1 formin homology domain 2 (FH2) substantially restored actin structures and partially suppressed the hTAC-GFP overaccumulation phenotype in ptrn-1 mutants. We conclude that the PTRN-1 CH domain is required to stimulate CYK-1 to facilitate actin dynamics during endocytic recycling. Synopsis Direct interaction of the actin regulator formin/CYK-1 with the patronin/CAMSAP-family microtubule-binding protein PTRN-1 reveals an unexpected role of patronin in actin cytoskeleton remodeling, which is critical in ensuring endocytic recycling transport of clathrin-independent cargo. PTRN-1 depletion impairs actin dynamics and recycling transport of clathrin-independent hTAC-GFP cargo in worm intestinal cells. The N-terminal calponin homology (CH) domain of PTRN-1 is essential for proper actin organization. Binding of CYK-1 to the PTRN-1 CH domain triggers release of self-inhibitory intramolecular interaction with the CYK-1 FH2 domain. Abberrant actin structures and overaccumulation of hTAC-GFP cargo in ptrn-1 mutant animals can be suppressed by expression of the CYK-1 FH2 domain. Introduction After endocytic uptake, cargo-loaded vesicles fuse with early endosomes, and the internalized molecules are sorted and destined for cell surface or other organelles (Maxfield & McGraw, 2004; Grant & Donaldson, 2009). Some of the endocytosed cargos are transferred to recycling endosomes for further sorting and delivery to the plasma membrane (Brown et al, 2000; Wang et al, 2000; Folsch et al, 2009). Accumulating evidence indicates that recycling transport is coordinated by different types of actin regulators (Puthenveedu et al, 2010; Winter et al, 2012; Delevoye et al, 2016; Gleason et al, 2016; Wang et al, 2016). Specifically, actin assembly, disassembly, and bundling play significant roles in cargo sorting and tubular membrane carrier biogenesis (Llado et al, 2008; Greene & Gao, 2009; Hagedorn et al, 2014; Steenblock et al, 2014; Wang et al, 2016). For example, abnormal actin disassembly results in the retention of cargo protein Tac in Arf6-positive recycling endosomes, demonstrating the necessity of filamentous actin in Arf6-mediated recycling (Radhakrishna & Donaldson, 1997; Schafer et al, 2000). As a master regulator of endocytic recycling, Arf6 is also capable of promoting actin assembly (Schafer et al, 2000). CAMSAPs/Nezha/Patronin are known to associate with microtubule minus-end to promote non-centrosomal microtubule stability (Goodwin & Vale, 2010; Hendershott & Vale, 2014; Jiang et al, 2014). PTRN-1 is the sole CAMSAPs/Patronin homolog in Caenorhabditis elegans and is broadly expressed in multiple tissues including the intestines (Richardson et al, 2014). In the epidermis, PTRN-1 functions redundantly with NOCA-1 to assemble non-centrosomal microtubule arrays during worm morphogenesis (Wang et al, 2015a). Also, PTRN-1 is required for microtubule stability and neuronal axon regeneration in neuronal processes (Chuang et al, 2014; Marcette et al, 2014; Richardson et al, 2014). Loss of PTRN-1 leads to upregulated microtubule dynamics in the PLM touch neuron axon (Chuang et al, 2014). PTRN-1 consists of an N-terminal calponin homology (CH) domain, a middle coiled-coils (CC) segment, and a C-terminal common domain of CAMSAP1, KIAA1078, and KIAA1543 (CKK; Baines et al, 2009; Goodwin & Vale, 2010; Chuang et al, 2014). Thus far, the functional significance of the N-terminal CH domain has not been directly evaluated. Recent studies in mammal indicate that the CKK domain mediates the association of CAMSAPs with microtubule minus-end, while the CC region promotes the microtubule binding of the CKK domain and functions with the CKK domain to suppress the microtubule treadmilling phenotype (Hendershott & Vale, 2014; Jiang et al, 2014). Consistently, the CC region of CAMSAP3 is implicated in the recruitment of microtubule minus-end to the apical side of human Caco-2 cells (Toya et al, 2016). In contrast, Drosophila Patronin CKK domain is localized along the microtubules, and the central CC region is capable of localizing in the microtubule minus-end and cooperating with the CKK domain to suppress microtubule minus-end dynamics (Goodwin & Vale, 2010; Hendershott & Vale, 2014). In C. elegans neurons, the CC region displays punctate labeling along processes. However, the cytosolic diffusive CKK domain is sufficient to rescue axonal regrowth defects and microtubule dynamic abnormalities independent of the CC region (Chuang et al, 2014), consistent with a role of the CKK domain in stabilizing microtubules. It is noteworthy that the microtubule depolymerization did not perturb PTRN-1-labeled puncta along neuronal processes and the PTRN-1a(ΔCKK)-positive puncta often failed to colocalize with microtubule marker EMTB-GFP, suggestive that the PTRN-1 subcellular localization is independent of microtubules (Richardson et al, 2014). Recently, a study in C. elegans epidermis has provided more comprehensive information on the PTRN-1 domains localization and the corresponding functional implications (Chuang et al, 2016). In line with the findings in neurons, the CKK domain is essential for the function of PTRN-1 in C. elegans epidermal morphology maintenance. Further analysis showed that in the epidermis, the CKK domain is distributed along microtubules and the overexpression of the CKK domain excessively stabilized microtubules and affected epidermal development (Chuang et al, 2016). Importantly, the presence of the CC domain appears to inhibit the activity of the CKK domain, suggesting that the proper PTRN-1 localization serves to tightly administer CKK domain activity (Chuang et al, 2016). Taken together, these results indicated that the CC region and the CKK domain are functionally distinct and often act synergistically in the microtubule-targeting and/or maintenance of microtubule stability. CAMSAP3 are coupled to actin filaments in Caco2 epithelial cells (Ning et al, 2016). The spectraplakin ACF7 anchors CAMSAP3 to actin filaments, maintaining the length and orientation of non-centrosomal microtubules. We found that the depletion of PTRN-1 resulted in the impairment of actin organization in C. elegans intestinal epithelia. We also found that PTRN-1 is required in the recycling transport of clathrin-independent cargo hTAC-GFP and that the N-terminal CH domain and the central CC region can complement the recycling defect of hTAC-GFP in ptrn-1 mutants. In an effort to characterize the mechanism underlying the abnormal actin reduction in ptrn-1 mutants, we were able to identify filamentous actin nucleator CYK-1/formin as a binding partner of PTRN-1. The formin family consists of a series of highly conserved proteins that bind to the barbed ends of actin filaments, promoting actin filament nucleation and elongation (Campellone & Welch, 2010). In mouse hippocampal synapses, mDia1/formin is crucial for the formation of endosome-like vacuoles (Soykan et al, 2017). Similar to ptrn-1 mutants, loss of CYK-1 led to the overaccumulation of hTAC-GFP in the cytosol. We showed that the N-terminal CH domain of PTRN-1 provides the binding interface for the N-terminal GTPase-binding domain (GBD) of CYK-1, which presumably encloses the actin-binding FH2 domain of CYK-1 via the interaction with the C-terminal DAD (Diaphanous Autoregulatory Domain; Zigmond, 2004; Chesarone et al, 2010). As expected, we observed that the presence of PTRN-1 promoted the CYK-1-mediated actin polymerization, whereas a truncated PTRN-1 lacking the CYK-1-interacting CH domain was unable to do so. The transgenic overexpression of CYK-1(FH2) containing fragment restored decreased levels of GFP-Utrophin-CH-labeled actin structures and sufficiently suppressed hTAC-GFP accumulation phenotype in ptrn-1 mutants. From our findings, we conclude that the CYK-1-mediated coupling of PTRN-1 with actin dynamics is essential for endocytic recycling. The central CC region of PTRN-1, a fragment required for microtubule positioning, is also essential. The operative connection of PTRN-1 with recycling transport appears to be independent of the C-terminal CKK domain. Results PTRN-1 is required for proper actin organization in C. elegans intestinal epithelia The precise regulation of the endosome-associated actin cytoskeleton is a prerequisite for the proper operation of endocytic recycling (Campellone & Welch, 2010; Wang et al, 2016). Irregular F-actin depolymerization causes the retention of cargo protein Tac in Arf6-labeled recycling endosomes, which demonstrates the necessity of F-actin in Arf6-mediated recycling transport (Radhakrishna & Donaldson, 1997; Schafer et al, 2000). To better understand the regulatory mechanisms of endosomal actin dynamics during endocytic recycling, we carried out a genetic screen for novel endocytic recycling and actin organization regulators in C. elegans. Our previous study showed that rab-10 mutant animals are viable and superficially normal in growth and development (Shi et al, 2010; Wang et al, 2016). Remarkably, rab-10 mutants accumulate hTAC-GFP (clathrin-independent recycling cargo, the α-chain of the human IL-2 receptor) in enlarged structures in the intestinal cytosol (Chen et al, 2006). Thus, we performed a genome-wide RNAi genetic screen to identify potential candidate proteins whose knockdown can induce the hTAC-GFP overaccumulation phenotype. After the phenotype validation, we found that RNAi-mediated expression knockdown of 54 candidate genes resulted in intracellular hTAC-GFP aggregation, including PTRN-1, ARX-7, GGTB-1, EXOC-7, HUM-2, and SEC-10. It has been reported that mammalian homolog of HUM-2/myosin functions together with Rab10 to mediate the delivery of GLUT4 storage vesicle to the plasma membrane (Chen et al, 2012). Moreover, SEC-10 and EXOC-7 were previously found to be implicated in the regulation of hTAC recycling transport in C. elegans intestine (Chen et al, 2014). arx-7 encodes a subunit of Arp2/3 complex, which is known to be essential for endocytic trafficking (Duleh & Welch, 2010). GGTB-1 is a C. elegans homolog of human RABGGTB (Rab geranylgeranyltransferase β subunit) that presumably catalyzes the add-on of geranylgeranyl group to the C-terminal cysteines of Rab proteins (Gutkowska & Swiezewska, 2012). Utrophin calponin homology domain (Utrophin-CH) was initially used to serve as an F-actin probe in mammalian cells (Burkel et al, 2007). Recently, the GFP-Utrophin-CH transgene was expressed in C. elegans neurons and faithfully reported the presynaptic F-actin network (Chia et al, 2012; Mizumoto & Shen, 2013). We performed fluorescent phalloidin staining in intestinal cells to validate the F-actin labeling of Utrophin-CH. As expected, transgenic mCherry-Utrophin-CH (ycxIs253)-labeled structures extensively overlapped with Alexa Fluor 488-phalloidin foci in the cytosol (Fig EV1A and A′). Within these candidate recycling regulators, we conducted a small-scale screen for the potential actin regulators and observed that PTRN-1 exhibited a significant effect on GFP-Utrophin-CH-labeled actin structures. PTRN-1 encodes the sole C. elegans homolog of CAMSAPs/Nezha/Patronin and is essential for neuronal microtubule stability and axon regeneration (Chuang et al, 2014; Marcette et al, 2014; Richardson et al, 2014). PTRN-1 is broadly expressed in multiple tissues of C. elegans, including the intestine, where it locates on microtubule marker EMTB-GFP-positive (ycxIs51) structures (Fig EV1B and B′; Richardson et al, 2014). To examine the requirement for PTRN-1 in actin organization, we deployed a ptrn-1 null allele (tm5597) containing a 593-nucleotide intragenic deletion (1,716–2,308 bp), which led to an early nonsense mutation (Fig EV1C; Appendix Supplementary Material 1; Chuang et al, 2014, 2016; Marcette et al, 2014; Richardson et al, 2014). In PTRN-1-depleted intestinal cells, we observed that actin marker GFP-Utrophin-CH- or Lifeact-GFP (pwIs1257)-labeled actin structures were significantly diminished (Figs 1A and A′, and EV1D–E′). Consistent with the results of actin markers, the signal obtained with the phalloidin staining was notably reduced in ptrn-1 mutants (Fig EV1F and F′). The actin defects in ptrn-1(tm5597) animals can be fully rescued by stable expression of the PTRN-1-mCherry fusion protein (ycxEx829; Fig 4D–D”). Colocalization analysis showed that PTRN-1a-mCherry partially overlapped with the actin marker GFP-Utrophin-CH in the intestinal epithelia (Fig 1B and B′, Pearson's correlation coefficient: ~72%). The CH domain of PTRN-1 could be a potential actin-binding domain, so we tested the CH-actin interaction using a co-sedimentation assay (Wang et al, 2016). Nevertheless, there was no detectable association between the CH domain (aa 1–359) and F-actin (Fig EV1G and G′). Click here to expand this figure. Figure EV1. Actin structures decrease in PTRN-1-depleted cells A, A′. Confocal image showing colocalization between Alexa Fluor 488-phalloidin and mCherry-Utrophin-CH (ycxIs253) in the intestinal cells. Phalloidin and mCherry-Utrophin-CH overlap well on tubular structures. Pearson's correlation coefficients for Alexa Fluor 488 and mCherry signals are calculated (˜92.5%), and error bars are SEM, n = 6 animals. B, B′. Confocal image showing colocalization between EMTB-GFP (ycxIs51) and PTRN-1-mCherry (ycxEx829) in the intestinal cells. EMTB-GFP and PTRN-1-mCherry colocalize well on tubular structures. Arrowheads and inset indicate positive overlap. White asterisks in the panels indicate intestinal lumen. Pearson's correlation coefficients for GFP and mCherry signals are calculated (˜72%), and error bars are SEM, n = 6 animals. C. The ptrn-1a open reading frame. The tm5597 allele contains a 593-nucleotide intragenic deletion (1,716–2,308 bp), which led to an early nonsense mutation. D, D′. Confocal images showing GFP-Utrophin-CH-labeled actin structures in the intestinal cells. In ptrn-1(RNAi) animals, GFP-Utrophin-CH (ycxIs100)-labeled actin structures significantly decreased. Error bars are SEM [n = 18 each, 6 animals of each genotype sampled in three different unit regions of each intestine defined by a 100 × 100 (pixel2) box positioned at random]. Asterisks indicate the significant differences in the one-tailed Student's t-test (***P < 0.001). E, E′. Confocal images showing Lifeact-GFP (pwIs1257)-labeled actin structures in the intestinal cells. In ptrn-1(tm5597) mutants, Lifeact-GFP-positive puncta diminished in intestinal epithelia. Error bars are SEM [n = 18 each, six animals of each genotype sampled in three different unit regions of each intestine defined by a 100 × 100 (pixel2) box positioned at random]. Asterisks indicate the significant differences in the one-tailed Student's t-test (**P < 0.01). F, F′. Confocal images showing Alexa Fluor 488-phalloidin labeling in the fixed intestinal cells. In ptrn-1(tm5597) mutants, the phalloidin staining significantly decreased. Error bars are SEM [n = 18 each, six animals of each genotype sampled in three different unit regions of each intestine defined by a 100 × 100 (pixel2) box positioned at random]. Asterisks indicate the significant differences in the one-tailed Student's t-test (***P < 0.001). G, G′. SDS–PAGE gel stained with Coomassie Blue showing the F-actin co-sedimentation of the GST-tagged PTRN-1 CH domain. GST-PTRN-1-CH (aa 1–359) did not co-sediments with actin filaments. P/S ratio (pellet/supernatant) was quantified in (G′), and error bars are SEM (n = 3). Data information: Scale bars represent 10 μm. Source data are available online for this figure. Download figure Download PowerPoint Figure 1. PTRN-1 is required for proper actin dynamics in intestinal epithelia A, A′. Confocal images showing GFP-Utrophin-CH-labeled actin structures in the intestinal cells. In ptrn-1(tm5597) mutants, GFP-Utrophin-CH (ycxIs100)-labeled actin structures significantly diminished in intestinal epithelia. White asterisks in the panels indicate intestinal lumen. Error bars are SEM (n = 18 each, six animals of each genotype sampled in three different unit regions of each intestine defined by a 100 × 100 (pixel2) box positioned at random). Asterisks indicate the significant differences in the one-tailed Student's t-test (***P < 0.001). B, B′. Confocal image showing colocalization between GFP-Utrophin-CH and PTRN-1-mCherry in the intestinal cells. PTRN-1-mCherry (ycxEx829) partially colocalizes with the actin marker GFP-Utrophin-CH (ycxIs100) in puncta. Arrowheads indicate structures labeled by both PTRN-1-mCherry and GFP-Utrophin-CH. Pearson's correlation coefficients for GFP and mCherry signals are calculated, and error bar is SEM (n = 6 animals). C, C′. Confocal images showing ACT-5/actin dynamics in the intestinal cells. Representative images of ACT-5-GFP (pwIs314) in wild-type and ptrn-1(tm5597) mutant animals were collected before bleaching (Pre-Bleach), immediately after bleaching (Bleach) and after bleaching (0.5, 1, 2, 2.5, 3, 3.5, 4, 4.5, 5 min). Black circles indicate bleached areas. (C′) Mean time courses of FRAP (±SEM) from eight animals of wild-type and ptrn-1(tm5597). Error bars represent SEMs from the mean (n = 8 each time point, eight animals of each genotype were sampled in the intestinal cells). Data information: Scale bars, 10 μm. Download figure Download PowerPoint ACT-5/actin is expressed in the intestine and executes a unique functional role in intestinal microvilli formation (Macqueen et al, 2005). To better characterize the defects of actin organization, we measured actin dynamics using the Fluorescence Recovery After Photobleaching (FRAP) assay (Greener et al, 2001; Wu et al, 2001; Shi et al, 2009). Compared with wild-type animals (~95.6% recovery), ACT-5-GFP (pwIs314) fluorescence recovery in puncta was significantly impaired in animals deficient in PTRN-1 (~21.8% recovery; Fig 1C and C′). The data suggest that PTRN-1 functions as a positive regulator of actin dynamics in worm intestinal epithelia. PTRN-1 partially colocalizes with RAB-5 and RAB-10 on sorting endosomes Proper actin organization is of great importance to the recycling process (Llado et al, 2008; Hagedorn et al, 2014; Wang et al, 2016). Abnormal distribution and dynamics of actin in ptrn-1 mutants prompted us to determine whether PTRN-1 resides on sorting endosomes. We compared GFP-tagged PTRN-1a with a set of well-established organelle markers in living animals. In C. elegans intestines, PTRN-1a-GFP (ycxIs58) labels sparse punctate structures, presumably the microtubule minus-end (Chuang et al, 2014; Marcette et al, 2014; Richardson et al, 2014). Live-cell imaging revealed the partial colocalization of PTRN-1a with RAB-10 (ycxEx684), a master regulator of clathrin-independent cargo recycling (Shi et al, 2010; Fig 2A and A′, Pearson's correlation coefficient: ~75.8%). RAB-10 partially colocalizes with canonical early endosomal marker RAB-5 on endosomes and regulates the endosome-to-plasma membrane recycling process (Chen et al, 2006; Shi et al, 2010). Further assays also demonstrated the partial overlap of PTRN-1a with mCherry-RAB-5 (pwIs846; Fig 2A and A′, Pearson's correlation coefficient: ~70.1%). However, we did not observe significant colocalization between PTRN-1a and late endosome marker RAB-7 (pwIs849), TGN marker MANS (ycxEx379), and ER marker TRAM (qxIs162; Fig 2A and A′). We conclude that PTRN-1a is likely to reside on endosomes, where it could potentially link endosomes to microtubules during endocytic transport. Figure 2. PTRN-1 localizes on sorting endosomes A, A′. Confocal image showing colocalization between PTRN-1-GFP and organelle markers in the intestinal cells. PTRN-1-GFP (ycxIs58) partially colocalizes with early endosomal marker mCherry-RAB-5 (pwIs846). Arrowheads and the inset indicate endosomes labeled by both PTRN-1-GFP and mCherry-RAB-5. Also, PTRN-1-GFP partially colocalizes with mCherry-RAB-10 (ycxEx684). In addition, PTRN-1-GFP occasionally overlaps with TGN marker MANS-mCherry (ycxEx379) and ER marker mCherry-TRAM (qxIs162). However, PTRN-1-GFP displayed little colocalization with late endosome marker mCherry-RAB-7 (pwIs849). Arrowheads indicate positive overlap. Pearson's correlation coefficients for GFP and mCherry signals are calculated, and error bar is SEM (n = 6 animals). B, B′. Confocal images showing PTRN-1-GFP-labeled structures in the intestinal cells. In rab-10(q373) mutants, PTRN-1-GFP-labeled structures accumulated on enlarged structures, and PTRN-1-GFP (ycxIs58) decorates the vacuoles edges in a dotted-line manner. In rme-1(b1045) mutants, PTRN-1-GFP accumulated on enlarged puncta. Nevertheless, PTRN-1-GFP did not label the recycling endosome vacuoles. Red asterisks in the panels indicate endosomal vacuoles. Black asterisks in the panels indicate intestinal lumen. Error bars are SEM (n = 18 each). Asterisks indicate the significant differences in the one-tailed Student's t-test (***P < 0.001, **P < 0.01). Data information: Scale bars, 10 μm. Download figure Download PowerPoint The previous study in C. elegans showed that rab-10 mutants accumulate RAB-5-labeled enlarged early endosome vacuoles near the basolateral membranes of intestinal cells (Chen et al, 2006). Using this prominent vacuoles phenotype, we confirmed the endosomal presence of PTRN-1. In the rab-10(q373) mutant background, we found that PTRN-1a-GFP-labeled structures accumulated on enlarged puncta (average intensity increased by ~3.73-fold), and the vacuole edge seems to be decorated by PTRN-1a-GFP in a dotted-line manner (Fig 2B and B′, the average edge intensity: ~56.5). To determine whether PTRN-1 also localizes on recycling endosomes, we examined the PTRN-1a-GFP localization in rme-1(b1045) mutants. Notably, PTRN-1a-GFP accumulated on enlarged puncta (Fig 2B and B′, the average intensity increased by ~4.4-fold), which is also the case in arf-6(tm1447) mutants (Appen
MoreTranslated text
Key words
polymerization,actin
AI Read Science
Must-Reading Tree
Example
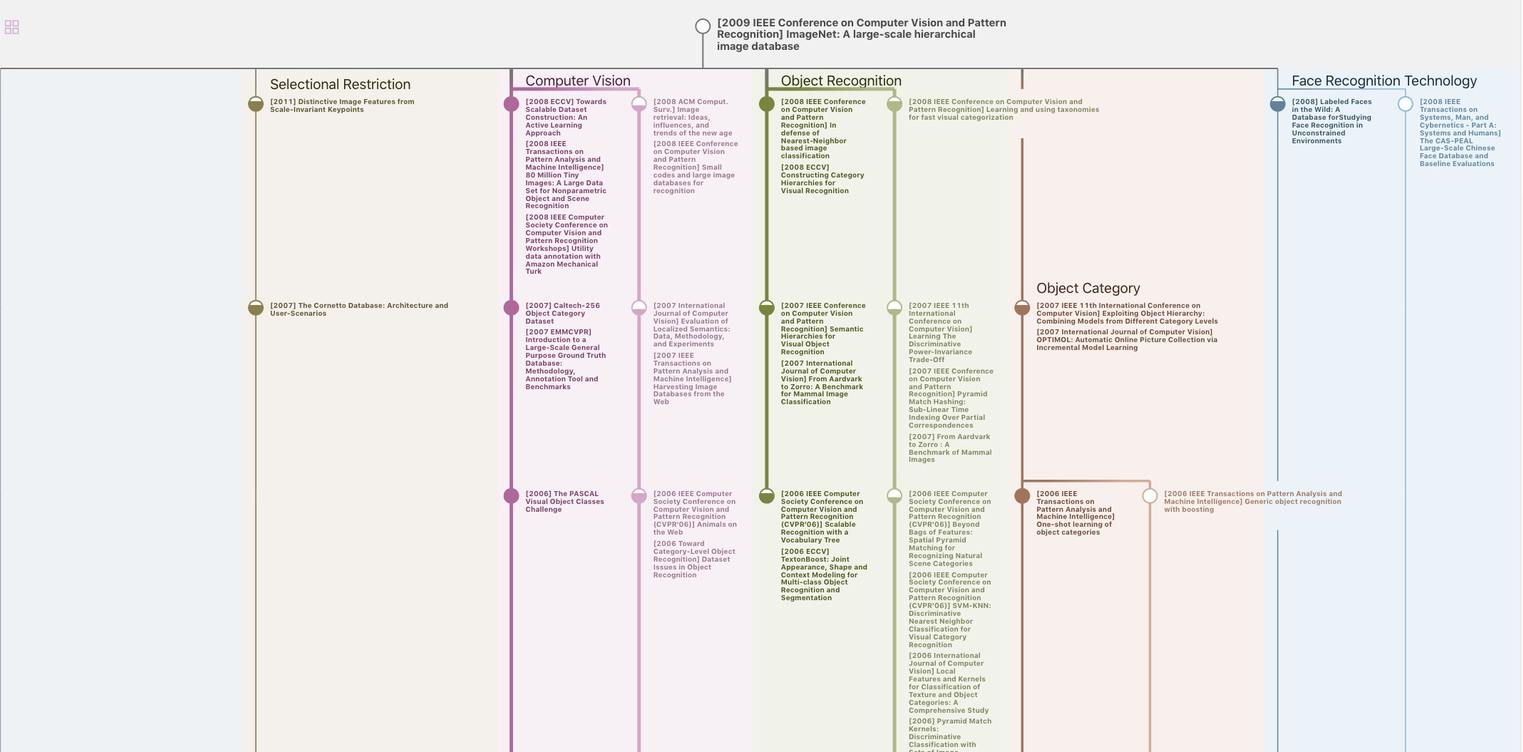
Generate MRT to find the research sequence of this paper
Chat Paper
Summary is being generated by the instructions you defined