Intralysosomal stabilization of glucocerebrosidase by LIMP-2: potential implications for efficacy of ERT
semanticscholar(2017)
摘要
Deficiency of the lysosomal enzyme glucocerebrosidase (GBA) occurs in patients with Gaucher disease (GD) as well as individuals with Action Myoclonus Renal Failure (AMRF). GD is caused by mutations in the Gba gene encoding GBA, whilst AMRF is due to mutations in the SCARB2 gene encoding the lysosomal membrane protein LIMP-2 that governs transport of newly formed GBA to lysosomes. AMRF manifests primarily as a neurological disorder without major visceral complications except for late onset renal disease. In contrast, the common nonneuronopathic (type 1) manifestation of GD is caused by visceral complications due to accumulation of macrophages laden with glucosylceramide, the major GBA substrate. For type 1 GD macrophage-targeted enzyme replacement therapy (ERT) is a registered treatment. For AMRF no treatment is presently available. Since deficiency of GBA is the prominent biochemical abnormality in AMRF patients, we studied the feasibility to supplement LIMP-2 deficient cells with recombinant enzyme. Studies were conducted with normal and AMRF fibroblasts as well as normal and LIMP-2 deficient HEK293 cells transduced with mannose receptor to promote uptake of mannose-terminated recombinant GBA. In LIMP-2 deficient cells, the endocytosed enzyme is abnormally fast proteolytically degraded in lysosomes. The half-life of the GBA in LIMP-2 deficient lysosomes is much shorter than in corresponding normal organelles. This suggests that inside the lysosome transient interactions of GBA with LIMP-2 stabilize the conformation of the enzyme and thus slow down proteolytic breakdown. Such mechanism implies that the efficacy with which lysosomes can be supplemented with GBA is intrinsically capped by LIMP-2 presence. In conclusion, the membrane protein LIMP-2 seems to fulfil a dual purpose in the life cycle of GBA, as transporter and as intralysosomal chaperone. Introduction GD is an autosomal recessive disorder caused by impaired glucosylceramide (GlcCer) catabolism as the result of GBA deficiency. The defect leads to prominent storage of GlcCer in lysosomes of macrophages that transform to “Gaucher cells” (lipid-laden macrophages), the hallmark of the disease1. GBA is encoded by the GBA gene and more than 200 different mutations have been described in GD patients2,3. The symptomatology of GD is remarkably heterogeneous, ranging from severe neonatal forms to an almost asymptomatic course. Based on the occurrence and age of onset of the neurological involvement, GD has been classified into acute and juvenile neuropathic forms (types 2 and 3) and the non-neuronopathic forms (type 1)1,4,5. Although there are no strict genotype-phenotype correlations, some mutations are associated with type 1 GD, like the homoor heteroallelic presence of GBA with the N370S mutation. On the other hand, homozygosity for the GBA allele with the L444P mutation is associated with a neuropathic form of the disease1,6. Characteristic visceral symptoms of all types of GD are cytopenia, hepatosplenomegaly and skeletal abnormalities7,8. It was unknown for a long time how GBA reaches the lysosome. In contrast to other lysosomal enzymes, GBA does not acquire phosphomannosyl moieties in its 4 N-linked glycans9. The enzyme is targeted to lysosomes by a mechanism independent of the mannose6-phosphate receptor10,11. Only in 2007, lysosomal integral membrane protein, type 2 (LIMP2) was identified as the protein responsible for the intracellular sorting of GBA: its absence causes GBA deficiency in almost all organs12. Mutations in the SCARB2 gene, encoding the LIMP-2 protein, are the cause of a disorder called Action Myoclonus Renal Failure (AMRF). This disease is characterized by reduced levels of GBA in the majority of cells. Patients develop heterogeneous symptoms such as glomerulosclerosis, progressive myoclonus epilepsy, and ataxia13–16. In addition, accumulation of undefined storage material in the brain has been reported17. Although LIMP-2 deficient individuals share a GBA deficiency with GD patients, AMRF presents markedly different from GD. Most striking is the absence of “Gaucher cells” in AMRF patients and the corresponding lack of elevated plasma chitotriosidase and CCL1818. Additionally, AMRF patients do not show visceromegaly16. Mutations in the GBA gene have recently also been linked to Parkinson disease (PD) and Lewy-Body dementia19–21. Such increased risk has also been reported for mutations in the SCARB2 gene22–24. In mice treated with the GBA inhibitor conduritol β-epoxide (CBE), the reduction in GBA activity induces the storage of α-synuclein25,26. Consistently, adenoviral overexpression of GBA in the brain decreases α-synuclein in transgenic mouse models of GD and PD27. Recently, α-synuclein storage was also described in LIMP-2 KO mice28. Already in 1991 enzyme replacement therapy (ERT) for type 1 GD was applied using alglucerase (Ceredase®) isolated from human placenta29. This was replaced in 1994 by imiglucerase (Cerezyme®) (hrGBA), a recombinant enzyme produced in Chinese hamster ovary cells30. Alternative recombinant enzyme preparations became subsequently available: velaglucerase alfa (VPRIV®) produced in a human fibroblast cell line31 and Taliglucerase alfa (Elelyso®), produced in carrots32,33. All therapeutic GBAs expose terminal mannose residues in order to be efficiently captured by the mannose receptor expressed by macrophages, the
更多查看译文
AI 理解论文
溯源树
样例
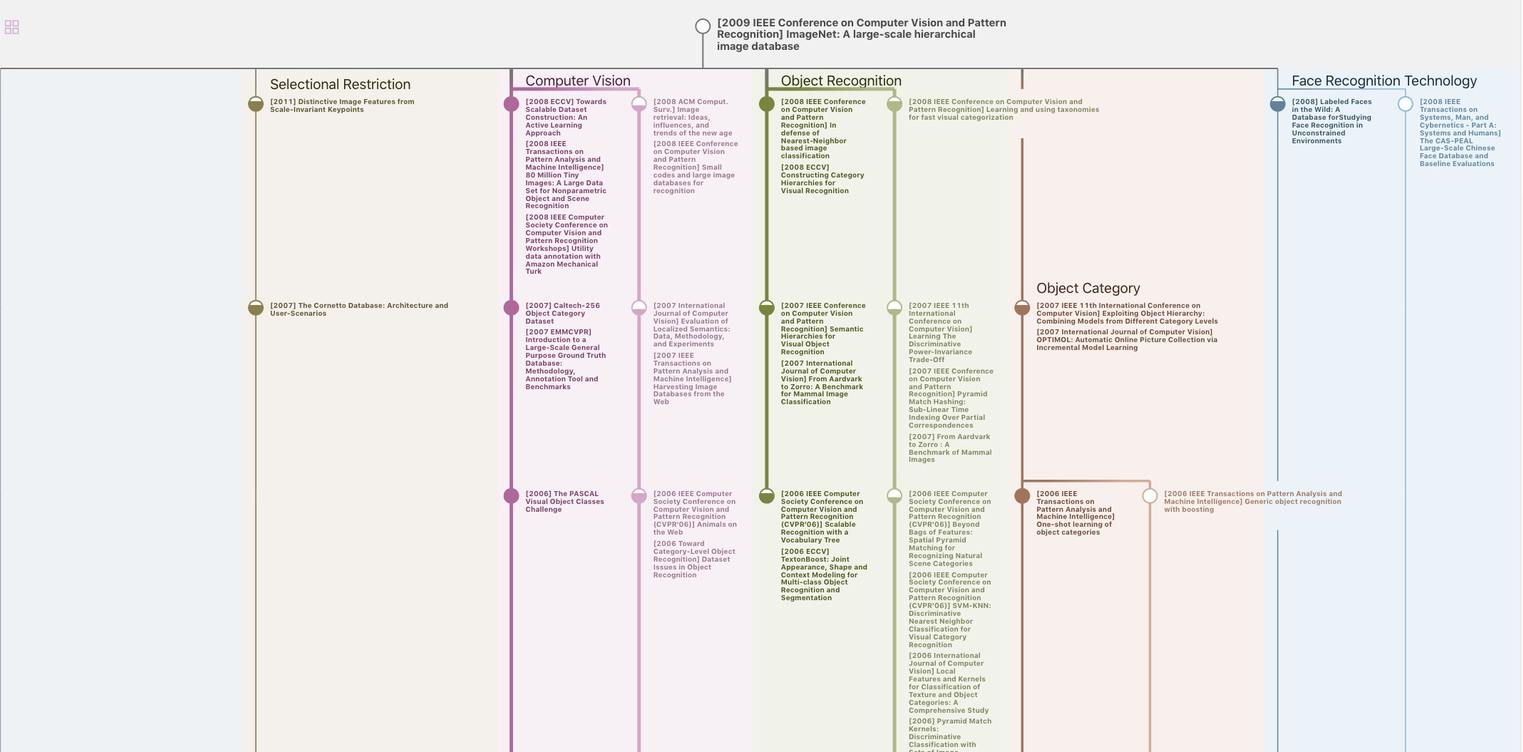
生成溯源树,研究论文发展脉络
Chat Paper
正在生成论文摘要