Role Of Dehydroepiandrosterone Sulfate As A Scavenging Antioxidant: The Evidence From Caenorhabditis Elegans' Nervous System Under Hypoxia
NEURAL REGENERATION RESEARCH(2021)
摘要
In nature, changes in O2 levels occur frequently. An inadequate supply of oxygen often happens in living systems, which induces an imbalance between oxygen entering cells and their oxygen demand to produce energy. The condition is known as hypoxia. Although hypoxia takes place in multiple physiological processes, it can be a primary cause of cellular injury and death (Nystul and Roth, 2004). Consequently, cells and tissues are habitually at risk, and they have developed many strategies to cope with low oxygen levels leading to pathological conditions (Fawcett et al., 2015). These adaptative responses to hypoxia though not entirely understood, seem to be evolutively conserved. Notwithstanding these responses, hypoxia can still have harmful effects. It should consider that the sensitivity of cells and organisms to hypoxic damage varies widely, and injuries in the central nervous system (CNS) are likely severe. In the case of the GABAergic system, hypoxia induces changes in mammal models. Neuroactive steroids have shown protective effects against hypoxic processes in mammals, but their exact mechanism of action at the cellular level is unclear. Neurosteroid dehydroepiandrosterone (DHEA) and its sulfate ester (DHEAS) have putative indirect and direct mechanisms underlying their protective effects. The indirect mechanism implies the conversion of DHEA into androgens or estrogen. Direct mechanisms include interacting with neurotransmitter receptors, prompting neurogenesis via non-classic steroid receptors, or having antioxidant properties and anti-inflammatory activities (Allolio and Arlt, 2002). In any case, it is complicated to assure these underlying mechanisms of action, particularly in mammal studies, because of their wide variety of targets in signal transduction pathways, in addition to transport and bioconversion processes. Recently, employing a model of chemical hypoxia in the nematode Caenorhabditis (C.) elegans, we demonstrated a neuroprotective activity of DHEAS probably associated with its chemical structure, functioning as a chain-breaking antioxidant (Gallegos-Saucedo et al., 2020). DHEAS and C. elegans: C. elegans possess steroid hormones like the pregnenolone (PREG) and other human-observed derivatives of pregnane and androstane, however, DHEA, DHEAS, estrogens, or androgens are not found naturally (Broue et al., 2007), suggesting that nematode lacks biosynthetic machinery involved in either DHEA formation from pregnenolone or DHEA conversion into estrogens and androgens downstream, and probably any related receptor or transporter would not be present. Admittedly, C. elegans has a nuclear receptor, DAF-12, however, given its hydrophilic nature, DHEAS requires active transport to get into the cell and produces genomic effects, but as noted earlier, so far, no steroid transporter protein has been found in the nematode. There is no evidence that DHEAS could be converted to lipophilic DHEA because, as far as we know, there is no steroid sulfatase that drives this reaction. Consequently, the model organism C. elegans makes it possible to rule out indirect and direct DHEAS mechanisms of action associated with any intracellular or extracellular receptor. Hypoxia and C. elegans: Hypoxia induces a wide diversity of cellular responses, and studies in living mammals are complicated because of the difficulty of measuring or controlling cellular O2 concentrations (Fawcett et al., 2015). In this context, hypoxia models in C. elegans provide information that would otherwise have been difficult to discern. The response of C. elegans to hypoxia includes regulatory systems and cellular processes evolutionarily conserved in mammals. Moreover, in C. elegans, it is possible to accurately control cellular O2 available and dismiss confusing compensatory responses that increase blood flow to hypoxic tissues appearing in higher animals. Notably, the model of chemical hypoxia with sodium sulfite in C. elegans emulates the physical hypoxia process that generates a hypoxic environment that causes typical hypoxia-related injuries in cells and tissues (Jiang et al., 2011). To lengthen survival with low oxygen, transcriptional changes are induced under hypoxic conditions after the activation of hypoxia-inducible factor (HIF-1). In C. elegans, a HIF-1 homolog induces expression of 67 from 110 genes altered by hypoxia during its development and adult life (Shen and Powell-Coffman, 2003). Gene expression under hypoxia condition is complex in embryonic and larval stages and deserves a thorough analysis not considered in this perspective. In post-mitotic tissues of C. elegans, hypoxia triggers a transcriptional program that, among other actions, reduces oxidative phosphorylation, increases glycolytic flux rates, reduces metabolic rate, or improves the efficiency of environmental oxygen removal (Van Voorhies and Ward, 2000), ensuring an optimal production of ATP and cellular integrity but raise the production of reactive oxygen species. Also, there are pathways activated in stressful and hypoxic conditions without requiring HIF-1 function. Insulin-like signaling pathway involves the transcription factor DAF-16/FOXO that induces the expression of antioxidant proteins like heat shock proteins, particularly the small heat shock protein HSP16 (Camargo et al., 2016). Hypoxia-related oxidative stress: Hypoxia induces enhanced formation of ROS, mostly mitochondrial hydrogen peroxide (H2O2), a product of an inefficient transfer of electrons to molecular oxygen in the final step of the respiratory chain (Nystul and Roth, 2004) occurring during oxidative phosphorylation disruption and increasing anaerobic glycolysis. H2O2 is relatively low reactive and acts as a second messenger, but what is most relevant, passes across the cell membranes and accumulate to excess levels due to its longer half-life concerning other ROS. The highly accumulated H2O2, combined with the presence of free metals, produces the hydroxyl radical (•OH), one of the most potent oxidants in nature, which is responsible for extracellular and intracellular oxidative stress. Thus, the supposition is that H2O2 comes from the more susceptible cells, namely neurons and muscle cells, damaging them and those in the surrounding. Jiang et al. (2011) reported in C. elegans, this kind of hypoxic damage in pharyngeal muscle and remarkably in nerve cells, mostly in nerve cords, where are visible cholinergic and GABAergic neurons. As noted above, the GABAergic system is prone to alterations by hypoxia, modifying either GABA levels or the availability and function of its receptors. Effects of DHEAS on GABAergic neuron injuries related oxidative stress by hypoxia in C. elegans: Given the preceding, we conducted a study in C. elegans to examine the effects of DHEAS on damage to the GABAergic system associated with hypoxia (Gallegos-Saucedo et al., 2020). With this purpose, we employed a model of chemical hypoxia similar to models of physical hypoxia to the effect that the stabilization of HIF-1 results from lowering the available oxygen (Jiang et al., 2011), and that also present gene expression (dependent or independent of HIF-1) typical of hypoxic cell response. We found that in EG1285 strain, worms expressing GFP in all GABAergic neurons, chemical hypoxia caused severe structural damage to the GABAergic neurons, including beading, tortuosity, and gaps. Additionally, the GABAergic system was functionally impaired, as evidenced by the shrinking response after nose touch stimulus that appeared more frequently. Considering that a greater frequency of this body shrinking response is symptomatic of a failure in the GABA system because, after the nose touch stimulus, a backward movement in the worm is normal and depends on good state GABAergic neurons in the ventral cord. DHEAS administration, on the other hand, reduced the severity of axonal damage induced on GABAergic neurons by chemical hypoxia and consequently lowered the ratio of shrinking response. The latter indicates that DHEAS conferred protection against damage by hypoxia in the nervous system. As in other cases of oxidative stress, we observed that the chemical hypoxia raised the DAF-16 translocation and HSP16.2 expression. Because HSP16.2 expression correlates with the appearance of ROS (Soti and Csermely, 2007), this confirms that hypoxia increases the level of ROS. Conversely, DHEAS did not increase the translocation of DAF-16 or the HSP16.2 expression. This finding suggests that DHEAS diminished the ROS accumulation, arguably H2O2, but it was unable to stimulate the production of antioxidant proteins through the DAF-16 transcriptional activities. As mentioned before, DHEAS is unable to get into cells, and consequently it cannot directly affect DAF-16. So, DHEAS surely neutralizes only the extracellular H2O2 (Figure 1).Figure 1: Proposed scheme for oxidative damage induced by chemical hypoxia, cell response, and effect of dehydroepiandrosterone sulfate on D-type GABAergic motor neurons in Caenorhabditis elegans.Due to low levels of oxygen, during chemical hypoxia, mitochondrial superoxide anion (O2 –) is raised on account of an inefficient transfer of electrons to molecular oxygen in the final step of the electron transport chain. O2 – is catalyzed to hydrogen peroxide (H2O2) by enzymes manganese or copper/zinc SOD. H2O2 molecules diffuse throughout mitochondrial and cell membranes, causing oxidative injury. However, through the oxidation of Fe2+, H2O2 favors HIF-1 stabilization and so, promoting cell integrity and viability. Furthermore, H2O2 could allow the DAF-16 translocation, activating the transcription of antioxidant proteins (SOD, CAT, HSP, etc.) and consequently improve oxidative stress resistance and survival by inhibiting the DAF-2 receptor extracellularly and insulin-like signaling pathway inside of the cell. Still, in H2O2accumulation, the noxious effects will prevail over good ones. In such a situation, DHEAS exerts antioxidant effects, mostly as a scavenger of external H2O2, reducing the oxidative injuries and response induced by DAF-16. Colored arrows depict inward and outward cell motion of transcription factors (blue) and H2O2 (red). Arrows indicate activations, blunt-ended lines, inhibitions, or in case of hypoxia, a decline in O2availability. CAT: Catalase; DHEAS: dehydroepiandrosterone sulfate; HSP: heat shock proteins; SOD: superoxide dismutase.Furthermore, the ILS pathway seems suppressed under hypoxia and activated following the DHEAS administration, which is relevant because the ILS pathway suppression allows the DAF-16 translocation and its transcriptional program, which brings us to the topic of how the ILS pathway is on/off to regulate DAF-16. Indeed, several studies have suggested the action of intracellular proteins (e.g. DAF-18/PTEN), but in C. elegans, DHEAS acts extracellularly and lacks specific receptors and transporters in the cell membrane. Estevez et al. (2014) proposed that H2O2 extracellularly induces phosphorylation of the substrate of DAF-2/IGFR, deactivating the ILS pathway. This observation accounts for the reversal of the DAF translocation in hypoxia when DHEAS neutralizes extracellular H2O2. Thus, ruling out other mechanisms, in our study, DHEAS only showed antioxidant activity, apparently as a scavenger of ROS. It is not well documented, for neither DHEAS nor DHEA, but that antioxidant activity could be associated with their steroidal multiple-ring structure able to accept electrons or the electrophilic sulfate groups, because of their strong capacity of hydrogen donation. This assumption is in line with in-vitro studies reporting DHEAS acted as a free radical scavenger and inhibitor lipid peroxidation at a lower or similar level than DHEA (van Rensburg et al., 2000). In conclusion, the neuroprotective activity of DHEAS under the hypoxia depends on its capacity as a ROS (H2O2) scavenger. DHEAS has an effect related to its chemical structure, probably acting as a chain-breaking antioxidant. In this connection, DHEAS may be related to the group of endogenous scavenging antioxidants that constitute the second line of defense against oxidative stress, which restrain chain initiation and break chain propagation reactions. Scavenging antioxidants are just after antioxidant enzymes and metal ion binding proteins, which compose the first line of defense. Therefore, the antioxidant action of DHEAS should occur in higher organisms. Besides, DHEAS is the most prevalent form of DHEA in circulation in human beings; consequently, this finding has relevant implications in neuroprotection investigations. That is to say, ROS scavenging certainly would not be the only antioxidant mechanism of DHEAS, especially in mammals. Based on the research findings, we stated that ROS scavenging could have a significant role in hypoxic processes not envisioned before, without precluding other possible neuroprotective mechanisms of DHEAS. In this connection, it is also pertinent to mention that we investigate the effect of DHEAS on the structure and function of the GABAergic neurons in untreated and DHEAS-treated hypoxic animals 24 hours after the hypoxic insult, but not at the time when it happens. Consequently, we cannot rule out that the action of DHEAS as an allosteric regulator of GABAA or NMDA receptors could be related to protection against hypoxic processes, as suggested studies in mammals. After all, the redundancy of the homeostatic functions is common in the complex nervous systems of higher organisms, and we believe that studies in mammalian models are needed to elucidate this point. Finally, DHEAS as a scavenger of ROS against hypoxia has a profound impact on how the animals and humans respond to decreased O2availability, considering that this situation is present during development, homeostasis, and disease.
更多查看译文
关键词
dehydroepiandrosterone sulfate,scavenging antioxidant,caenorhabditis elegans
AI 理解论文
溯源树
样例
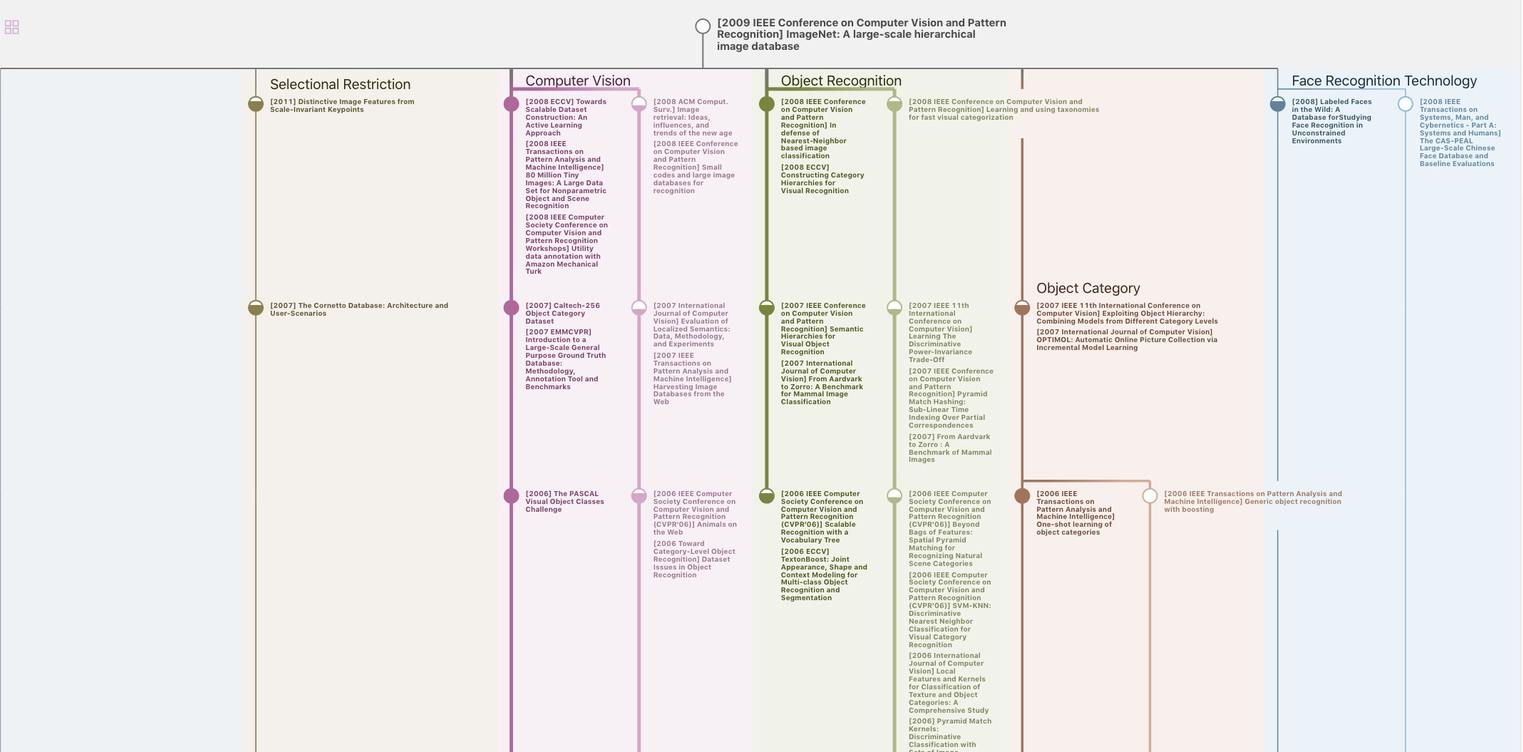
生成溯源树,研究论文发展脉络
Chat Paper
正在生成论文摘要