The effect of deposition conditions on atmospheric pitting corrosion location under Evans droplets on 304 L stainless steel Street
semanticscholar(2018)
Abstract
Pit location during atmospheric corrosion of 304L stainless steel under MgCl2 droplets depends on initial droplet concentration. Pits formed predominantly in the center of the droplet for concentrations ≥ 4 M, closer to the perimeter for 1.5-3 M, and were randomly distributed for concentrations ≤ 1 M. Pits initiated only after the droplets had evaporated to a critical concentration > 3 M, where droplets deposited with lower initial concentrations were thinner. The results can be explained in terms of “differential aeration” and IR drop effects, showing that corrosion in “splash zones” may differ from that under aerosol salt deposit layers that deliquesce forming initially saturated solutions. INTRODUCTION Atmospheric corrosion of metals occurs when small amounts of salt are deposited onto exposed surfaces. In “splash zones”, dilute droplets will land on metal surfaces, and then concentrate as they equilibrate with the ambient relative humidity [1-4]. However, in other conditions, solid aerosol salt particles can accumulate on surfaces, and then deliquesce when the ambient relative humidity is sufficiently high, forming concentrated salt droplets when it initially wets [5, 6]. At equilibrium the water activity of saline solutions will equal that of the atmosphere, which is determined by the relative humidity (RH) [7]. It is therefore possible to get highly concentrated saline solutions that allow the corrosion of metals usually considered resistant to attack when immersed in dilute saline solutions. The restricted solution volume also shortens the pathway of oxygen from the air-solution interface to the metal-solution interface [8] allowing oxygen reduction reactions to continue and drive further anodic dissolution. As such, the dominant limiting factor to atmospheric corrosion propagation is generally considered to be the cathodic current [9, 10]. Dilute droplets of saline solution have long been used to simulate the effects of atmospheric corrosion. The first major discussion of corrosion under droplets was by U.R. Evans [11-13] who used NaCl with pH indicator—phenol-phthalein/potassium ferricyanide—on freshly ground mild steel to show a strong trend for anodic dissolution at the center of droplets and cathodic reactions occurring around the droplet edge. This was explained by “differential aeration”, with oxygen supply to the surface of the metal being significantly easier at the edge of the droplet where it is thinner. Evans also observed that where corrosion initiated at the edge of the droplet, it slowly migrated towards the center as the passive layer there was less stable. The Evans droplet experiment is noted to be of particular use with alloys that have less stable passive oxide layers [14, 15]. Chen and Mansfeld [16] used scanning Kelvin probe on steel to show a strong cathodic region dominates at, and beyond, the edge of a droplet. Risteen et al. [17] noted corrosion behavior became progressively more filiform and less Evans-like in droplets under 500 μm diameter on 1010 steel. Li and Hihara [18] observed corrosion occurring in the center of NaCl droplets on ultrapure iron for droplets with diameters greater than 200 μm. Wire beam electrode (WBE) arrays of Zn alloy [19] also indicate corrosion towards the center of droplets of sufficient size, with modelling supporting the influence of oxygen diffusion resulting in differential aeration [20]. However, the Evans droplet experiment is less useful in interpreting corrosion of alloys with stable passive oxide layers. Using large datasets, a wide range of pitting initiation sites has been observed on 316L [21] and 304L stainless steels [3], with pit location under the droplet often influencing the pit morphology. Some examples of wet/dry cycling of MgCl2 droplets on 304 stainless steels have shown no strong trend in pitting location when cycled up to 6 times in both as-received [22] and sensitized [23] conditions. Longterm corrosion of 2205 duplex stainless steel has shown corrosion distributed around the droplet with significant selective dissolution of the austenite phase at the edges [24]. Aluminum alloys have generally shown pitting location to be determined by microstructure and not differential aeration [25]. Knight et al.[26] confirmed this when they observed that droplets of 0.6 M NaCl on AA2025 and AA7075 aluminum alloys had no preferential pitting location over a range of droplet diameters up to 10 mm, though this was not quantified. Interestingly, Oltra et al. [27] did see an influence of differential aeration on AA2011 aluminum alloy, observing attack around Al2Cu intermetallic particle towards the edge of droplets of very pure water. This was supported by the observation of increased pH in the edge regions of the droplet as compared to the center. It has been suggested [28] that the lack of trend in position of pitting site on highly passive alloys may be due to a reduced rate of oxygen consumption on the altered passive layer, preventing differential aeration from developing in the droplet. However, WBE arrays constructed with 304 stainless steel [29] show that large droplets cause a trend in corrosion towards the droplet edge rather than the center, suggesting oxygen access does play a role. A threephase boundary region, where oxygen is readily accessible, has been suggested to account for this [30]. Dilute droplets of saline solution have been used to simulate both splash zone and aerosol deposition scenarios despite the difference in the early stages of experimental conditions. This raises questions about the universality of the droplet deposition technique, particularly in light of recent work that has found differences when directly comparing droplets and dry salt deposition [31-35]. Highly concentrated solutions show reduced diffusivity, which hinders mass transport during pitting, and reduced ionic conductivity, which contributes to an increased IR-drop between anodic and cathodic regions [3]. Pitting initiation on stainless steel is stochastic [36-38] and conclusions from small datasets are unreliable. High-throughput methods [3, 39], where large numbers of droplets are deposited at once, allow statistical analysis to be conducted on pitting corrosion to see the influence of experimental variables. Several statistical tools exist that allow trends in categorical data to be examined and quantified [40]. The Chi-squared (χ2) test is a method of hypothesis testing commonly used in categorical datasets where sampling method is considered random. It is particularly useful in testing the “null hypothesis”, i.e. that the results of a dataset are independent of a particular variable. Chi-squared tests rely on a standard distribution determined by the degrees of freedom in the dataset, and produce a probability that the null hypothesis is true. The binomial standard deviation is also used on categorical data where just two outcomes are possible and where the results are independent from each other. This technique gives a range of the variability of a dataset from the mean. This study investigates the influence of deposition conditions on pitting location under droplets of MgCl2 on 304L stainless steel. High-throughput methods are used to collect large volumes of data so that statistical techniques can be applied to determine trends in pitting location.
MoreTranslated text
AI Read Science
Must-Reading Tree
Example
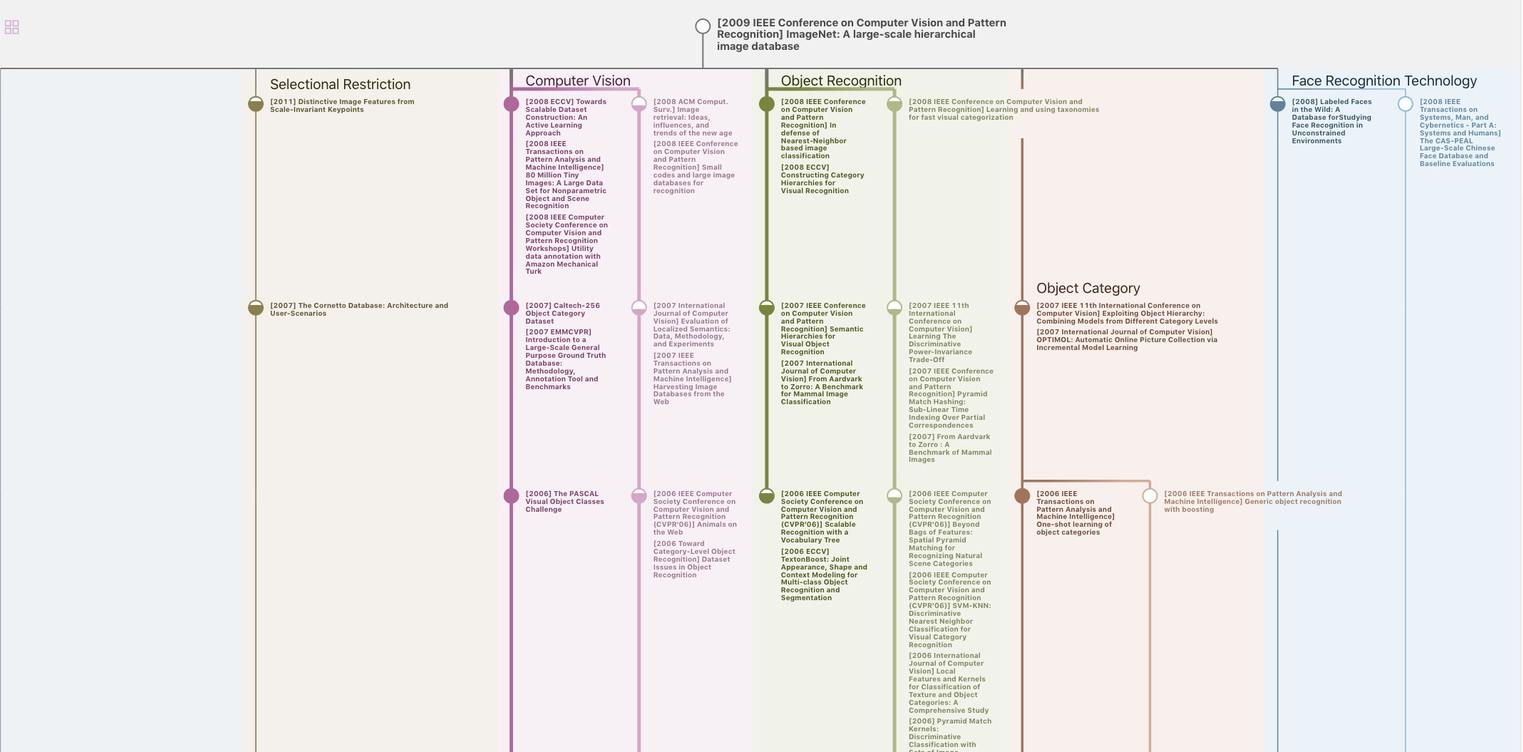
Generate MRT to find the research sequence of this paper
Chat Paper
Summary is being generated by the instructions you defined