The Effect of Nitrogen , Sulfur , and Phosphorus Compounds on Bioremediation of Oil Spills by Pseudomonas fluorescens and Bacillus subtilis
semanticscholar(2013)
摘要
Bioremediation involves microbial metabolism of harmful substances. This study was undertaken to enhance oilspill bioremediation through the addition of sodium sulfate, sodium nitrate, and sodium phosphate to samples containing Pseudomonas fluorescens and Bacillus subtilis. Since these bacteria often utilize nitrates during cellular respiration and nitrate ammonification, respectively, it was hypothesized that sodium nitrate would increase the amount of hydrocarbon metabolites present in samples more than sodium sulfate or sodium phosphate. Samples containing different combinations of bacteria, .1 and .01 molar solutions of the above nutrients, salt water, and crude-oil-substitute hexadecane (utilized to replicate the long hydrocarbon chains found in petroleum) were shaken at 27°C for seven or 14 days. Bacterial concentration was estimated by turbidity measurements. Each organic layer was analyzed with gas chromatography to identify any smaller hydrocarbons. The data from two trials partially disprove the hypothesis. While the treatment of .1 M sodium nitrate caused one of the greatest increases in bacterial concentration with P. fluorescens and B. subtilis, the chromatographic data from these treatments differed only marginally from the positive controls and other treatments. Additionally, the data suggested that substantial metabolite formation occurred only in P. fluorescens samples with .1 M and .01 M treatments of sodium phosphate. However, the .1 M nutrient combination and .01 M sodium sulfate treatments caused the greatest hexadecane disappearance. These results are relevant to the enhancement of bioremediation techniques; a need exemplified by the Deepwater Horizon catastrophe. Introduction Marine oil spills represent one of the most devastating accidents occurring worldwide, as demonstrated by the Deepwater Horizon explosion on April 20, 2010. Preventing these spills entirely would be practically impossible; however, preparing for them can limit the damage they cause. Increasing the rate at which oil spills in the ocean can be cleaned would save millions of organisms and acres from lasting harm. This project was completed to increase the effectiveness of bioremediation techniques by the addition of inorganic nutrients to Pseudomonas fluorescens and Bacillus subtilis for use during oil spills. According to the EPA, almost 14,000 oil spills are reported each year1. Many different techniques are used to attempt to clean oil spills. Booms–barriers that extend about three feet below the surface of the water can be used to contain the oil, while The Effect of Nitrogen, Sulfur, and Phosphorus Compounds on Bioremediation of Oil Spills by Pseudomonas fluorescens and Bacillus subtilis Meghan Shea1*, Sandra Litvin2, and Anastasia Chirnside3 Student1, Teacher2: Unionville High School, Kennett Square, PA 19348 Mentor3: University of Delaware, Newark, DE 19716 *Corresponding author: meghan.shea28@gmail.com INTERNSHIP ARTICLE skimmers act as vacuums to remove this contained oil1. Sorbents, or materials that absorb or adsorb oil, are also commonly used to remove the final traces of oil from an oil spill1. However, the technique that requires the least human effort and is often the most successful in the long run is bioremediation1. According to the Civil Engineering Department of Virginia Tech, bioremediation is “the application of biological treatment to the cleanup of hazardous chemicals in the soil and surface or subsurface waters”2. Certain bacteria are able to feed on the contamination, which in this case is long hydrocarbon chains, and use the energy for growth and reproduction2. Through this process, the oil is metabolized into water and carbon dioxide. While this process usually occurs naturally, it can be stimulated through the addition of nutrients and microbes, which is known as bioaugmentation. Common organisms used in the bioaugmentation of petroleum are Pseudomonas, Proteus, Bacillus, Penicillum, and Cunninghamella. For this experiment, two rod-shaped, aerobic, heterotrophic microbes, Pseudomonas fluorescens and Bacillus subtilis, were used to degrade oil. P. fluorescens colonizes soil, water, and plant surface environments and is motile due to multiple polar flagella3. This Gram-negative bacterium can utilize nitrate as an electron accepter in place of oxygen during cellular respiration3. B. subtilis, a Gram-positive bacterium with peritrichous flagella, also inhabits soil and water4. In place of crude or motor oil, both of which contain additives that react with different aspects of this experiment making data collection difficult, HD (see figure 1 for abbreviations and acronyms) was utilized to replicate the long hydrocarbon chains found in petroleum. HD is an alkane hydrocarbon with a 16-carbon chain. Often, nitrogen, phosphorus, and sulfur are the limiting factors of bioremediation in aquatic environments. Therefore, sodium nitrate (NaNO3), sodium phosphate (Na3PO4), and sodium sulfate (Na2SO4) were used as sources of the above nutrients in order to determine which has the greatest effect on the bioremediation of hydrocarbons by P. fluorescens and B. subtilis. All three inorganic nutrients are soluble in water, making them effective for aquatic oil spills. In P. fluorescens, 30 Figure 1. Abbreviations and acronyms. Meghan Shea, Sandra Litvin, and Anastasia Chirnside Page 2 of 8 31 Materials and Methods Inoculating liquid cultures of P. fluorescens and B. subtilis: One liter of nutrient broth was prepared using the standard procedure found on the container and sterilized using an autoclave. 150 ml of nutrient broth were added to each of three clean Erlenmeyer flasks labeled B. subtilis, P. fluorescens, and Spectronic 20 control, respectively. The three flasks were autoclaved. The flask labeled P. fluorescens was inoculated with bacteria from a pure colony from Carolina Biological, using a metal inoculating loop and standard flaming techniques to avoid contamination. The inoculation was repeated twice more with P. fluorescens, re-inoculating the same flask to ensure adequate bacterial growth. The above procedures were repeated with the B. subtilis pure colony and the flask labeled B. subtilis. All three flasks – two inoculated and one control – were incubated at 27oC. Creating a standard spec-20 absorbance curve for each bacterium: A Spec-20 was set to a wavelength of 686 nm and left to warm up for a minimum of 15 minutes. Eleven clean culture tubes were obtained and labeled 1-11. Using different pipettes, turbid P. fluorescens liquid culture and nutrient broth were added to each tube to a total volume of 10.0 ml. The volume of liquid culture was reduced by 1.0 ml in each tube from 10.0 ml to 0.0 ml. Using a vortex mixer, each tube was mixed thoroughly for 1 – 2 seconds. After mixing, 3-4 ml of broth from tube 11 were transferred to a clean Spec-20 tube and the outside of the tube was wiped with a Kimwipe. The tube was placed in the Spec-20 and used as a blank, following the instructions for the specific machine. Starting with tube 10 and going backwards to tube 1, 3-4 ml of each dilution were transferred to the Spec-20 tube and the absorbance was measured. Using the absorbance data, a X-Y scatter plot of dilution factor vs. absorbance was created and a second order polynomial trend line was added to the plot. From the plot, the range over which absorbance was proportional to bacterial concentration was identified, and a second plot was created using only this range. A linear trend line was added to this nitrate is often incorporated in metabolism by serving as an electron acceptor during cellular respiration3. B. subtilis uses nitrate ammonification in anaerobic conditions4. However, the specific use of these nutrients during the hydrocarbon metabolism of P. fluorescens and B. subtilis has not yet been studied. Since the two bacteria utilize nitrate during cellular respiration in anaerobic conditions and nitrate ammonification, respectively, it was hypothesized that sodium nitrate, an inorganic nutrient that provides nitrogen to the microbes, would cause the greatest HD degradation and production of smaller hydrocarbons. To test this hypothesis, samples were prepared containing different combinations of bacteria, .1 and .01 molar solutions of the above nutrients, salt water, and crude-oil-substitute hexadecane. The turbidity of each sample was measured daily using a Spec-20. An absorbance standard curve for each bacterium coupled with serial-dilution plating facilitated the conversion of absorbance to CFU/ml. After shaking at 27°C for 7 or fourteen days, the organic layer was removed from each sample using an Acetonitrile-based Solid Phase Extraction. Each organic layer was analyzed with gas chromatography (FID) to identify any smaller hydrocarbons, the presence of which would indicate successful bioremediation. new plot. Using the equations of the trend lines from the first and second plots, a correction formula was derived to correct for linearity at high absorbance readings. The entire procedure above was repeated with a turbid B. subtilis culture to obtain a second corrected absorbance curve. Calibrating absorbance measurements with viable cell counts: One liter of nutrient agar was prepared using the standard procedure found on the container, sterilized using an autoclave, and poured into sterile Petri dishes. 16 sterile culture tubes were obtained and labeled 10-1 – 10 -16. Using a 10-ml pipette, 9 ml of sterile nutrient broth were added to each culture tube. 32 nutrient agar Petri dishes were obtained and two were labeled 10-1, two 10-2, etc., through 10-16. Using a Spec-20 and the procedure detailed above, the absorbance of a turbid P. fluorescens culture was recorded. Using a 1-ml micropipette, 1 ml of turbid P. fluorescens culture was transferred to the 10-1 tube. The dilution was mixed thoroughly by vortexing for 1 – 2 seconds. Using a new pipette tip, 1 ml of the 10-1 dilution was withd
更多查看译文
AI 理解论文
溯源树
样例
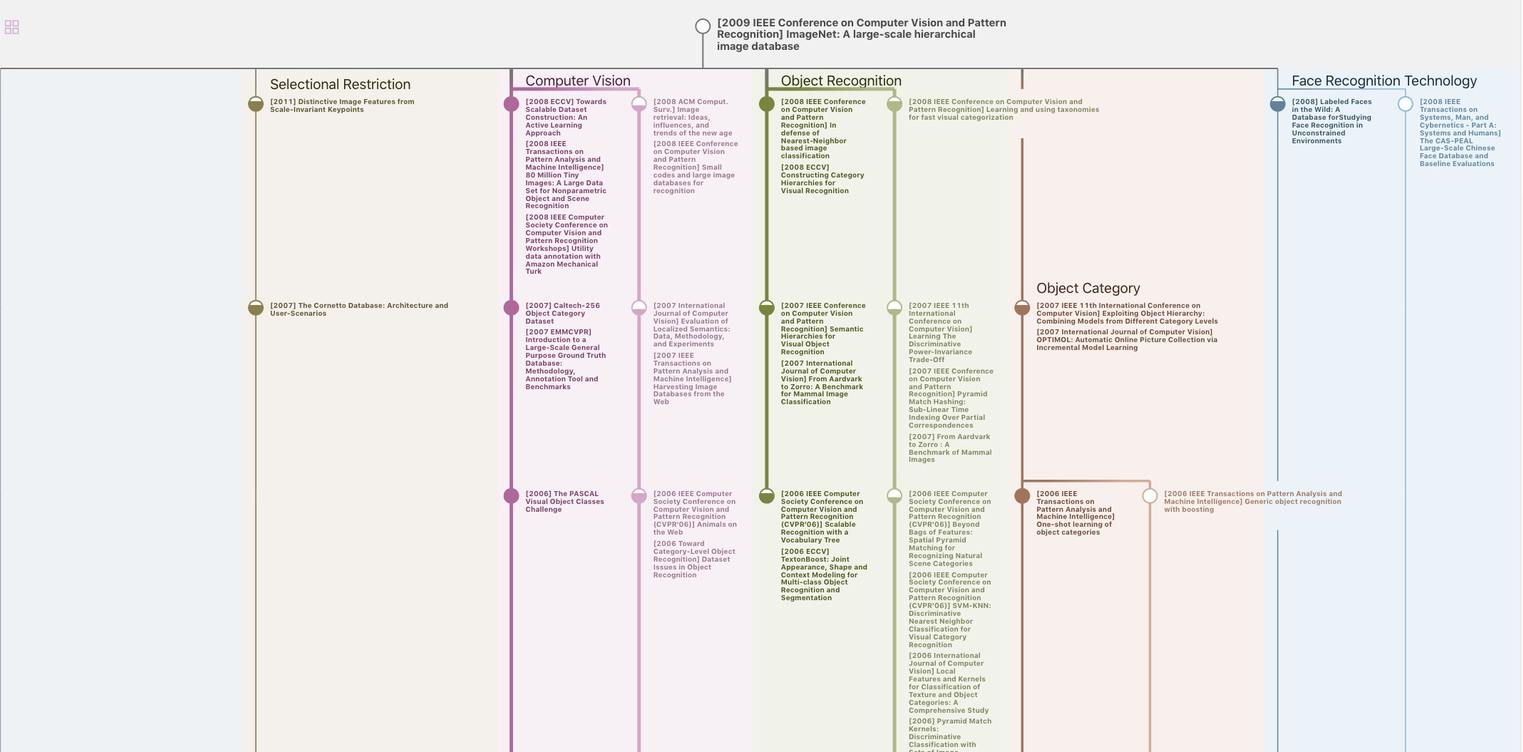
生成溯源树,研究论文发展脉络
Chat Paper
正在生成论文摘要