Cumomer Analysis of Cancer Cell Metabolism
semanticscholar(2015)
摘要
A network model for determination of tumor metabolic fluxes from C NMR kinetic isotopomer data has been developed and validated with perfused human DB-1 melanoma cells carrying the BRAF V600E mutation, which promotes oxidative metabolism. The model generated in the Bonded Cumomer formalism describes key pathways of tumor intermediary metabolism and yields dynamic curves for positional isotopic enrichment and spin-spin multiplets. Cells attached to microcarrier beads were perfused with 26 mM [1,6-C2]glucose under normoxic conditions at 37C and monitored by C NMR spectroscopy. Excellent agreement between model-predicted and experimentally measured values of the rates of oxygen and glucose consumption, lactate production and glutamate pool size validated the model. ATP productions by glycolytic and oxidative metabolism were compared under hyperglycemic normoxic conditions resulting in 51% of the energy coming from oxidative phosphorylation and 49% from glycolysis. Even though the rate of glutamine uptake was ~50% of the TCA cycle flux, the rate of ATP production from glutamine was essentially zero (no glutaminolysis). De novo fatty acid production was ~6% of the TCA cycle flux. The oxidative PPP flux was 3.6% of glycolysis, and three non-oxidative PPP exchange fluxes were calculated. Mass spectrometry was then used to compare fluxes through various pathways under hyperglycemic (26 mM) and euglycemic (5mM) conditions. Under euglycemic conditions glutamine uptake doubled, but ATP production from glutamine did not significantly change. A new parameter measuring the Warburg effect (the ratio of lactate production flux to pyruvate influx through the mitochondrial pyruvate carrier) was calculated to be 21. Cancer cells exhibit metabolic patterns that differ significantly from those of terminally differentiated adult tissues. They require rapid production of both energy and biosynthetic precursors to sustain a high rate of proliferation. http://www.jbc.org/cgi/doi/10.1074/jbc.M115.701862 The latest version is at JBC Papers in Press. Published on December 24, 2015 as Manuscript M115.701862 Copyright 2015 by The American Society for Biochemistry and Molecular Biology, Inc. by gest on July 0, 2017 hp://w w w .jb.org/ D ow nladed from Cumomer Analysis of Cancer Cell Metabolism 2 The network of biochemical pathways underlying these processes is complex. Most of the individual enzymatic conversions involved in this network have probably already been identified, but a comprehensive and quantitative description of the fluxes involved is lacking. Methods to produce such descriptions will likely be very useful in the design of new therapeutics that specifically target the metabolic abnormalities of cancer as well as in clinical implementation of noninvasive methods for detection of cancer and its therapeutic response. The kinetics of C-isotope labeling as monitored by NMR spectroscopy has been used to measure flux through various metabolic pathways of perfused isolated cells and organs and in vivo (14). Kinetic data provide estimates of absolute flux, whereas steady-state data measure relative flux. Both types of analysis require validated mathematical models, which have been formulated for the heart (5-10), liver (11-13), brain (14-16), and tumors (17). Only the heart model has been validated by comparison between model-derived and experimental parameters (6,7). Modeling of tumor metabolic fluxes has been limited to steadystate analysis with dynamic measurements of glucose uptake and lactate production subsequently added to convert to absolute fluxes; none of these models has been validated. To the best of our knowledge, this is the first validated dynamic flux model of tumor intermediary metabolism based on C NMR isotope kinetic data. Mathematical models of C MRS data are based on measurement of positional isotopic enrichment and/or on isotopomer analysis, which takes into account spin-spin coupling of labeled carbon atoms. Bonded Cumomer analysis includes both positional enrichment and isotopomer modeling and facilitates the formulation of kinetic differential equations. This method requires fewer equations than classical isotopomer analysis and is based on internal symmetry properties of metabolite isotopomers. Our laboratories have developed a Continuous Stirred Tank Bioreactor suitable for high-resolution C NMR spectroscopy of cultured cancer cells that can produce detailed spectra with good temporal resolution while simultaneously monitoring the oxygen consumption rate (18-21). Specifically, we measured the time course for labeling of key intermediates such as glutamate, aspartate, alanine, lactate and fatty acyl groups in real time during growth with C labeled glucose and unlabeled glutamine as substrates. Here we demonstrate that absolute flux measurements of perfused tumor cells can be obtained in several hours from ~5 x 10 tumor cells in this bioreactor system, and the data can be analyzed using a refined isotopomer analysis method called bonded cumomer (BC) analysis (22) that utilizes only ~210 differential equations to describe most of tumor intermediary metabolism, whereas the heart model of Chance et al. required ~325 differential equations to describe just TCA cycle activity in terms of positional enrichment. To validate the BC model, we compared model derived and experimental values of the following parameters: O2 consumption rate (MRO2), glutamate pool sizes, as well as rates of glucose consumption and lactate release. This study permits direct measurements of energy production by glycolytic and mitochondrial pathways and quantitative evaluation of the Warburg effect. It also provides a basis for future studies of the effects of various therapeutic interventions on tumor metabolism. While studies of perfused tumor cells in a bioreactor facilitate accurate and reproducible measurements, this model is also applicable to in vivo studies in animal models and humans. Recent studies of ex vivo surgically resected human brain (23) and lung (24) tumors point to the potential utility of in vivo C MRS clinical applications. EXPERIMENTAL PROCEDURES Cell Culture—DB-1 human melanoma cells were derived from a lymph node metastasis (Thomas Jefferson University Hospital, Philadelphia, PA). The cells were obtained before administration of any treatment. Cells were cultured from the tumor and cryopreserved after the 16 passage. The presence of melanoma cell surface antigens was confirmed with monoclonal antibodies (25). For routine culture, DB-1 cells were grown as monolayers at 37°C in 5% CO2 in αMEM medium supplemented with 10% fetal bovine serum, 2 mM glutamine, 26 mM glucose and 1% (v/v) non-essential amino acids and 10 mM HEPES buffer. The doubling time in tissue culture flasks was 48 h (26). Cell Perfusion and NMR Spectroscopy— For cell perfusion studies, DB-1 cells were cultured in DMEM with 1% (v/v) non-essential amino acids (Invitrogen), 10% fetal bovine serum, 10 mM by gest on July 0, 2017 hp://w w w .jb.org/ D ow nladed from Cumomer Analysis of Cancer Cell Metabolism 3 HEPES, 4 mM glutamine and 26 mM glucose (complete DMEM). During NMR experiments, ~5 x 10 cells were grown on the surface of non-porous microcarriers that had a mean diameter of 170 microns (Solohill, Ann Arbor, MI). The surface of the microcarriers was coated with either collagen or ProNectinF to enhance cell attachment and proliferation. The microcarriers were tightly packed inside a 20 mm NMR tube. They were perfused continuously with the system shown in Figure 1A. A detailed description of the perfusion system including flow rates, cell adhesion procedures, etc. has been presented previously (20). A peristaltic pump (Masterflex, Cole Parmer, Chicago, IL) was used to circulate medium through the microcarriers at a flow rate of 12 ml/min. Before entering the cell mass, the medium flowed through a gas exchange module where carbon dioxide was removed and oxygen was added across a silicone membrane (thin-wall silicone tubing). Subsequently, the medium was warmed to 40° C, and the oxygen level was measured continuously with a polarographic oxygen probe (Mettler-Toledo, Columbus, OH). The steady-state oxygen level of the medium at the inlet of the cell mass was maintained near air saturation. A second polarographic oxygen probe was used to detect the oxygen level of the medium coming out of the NMR tube so that the oxygen consumption rate of the culture could be determined continuously. A pH probe (Mettler-Toledo, Columbus, OH) was also used downstream of the outlet oxygen probe. The pH of the medium was controlled by adjusting the level of CO2 in the gas exchange module. The temperature of the medium entering the NMR tube was fine-tuned with a microstat-controller and an electrical resistance heater to 37±0.2oC. The glucose concentration in the recirculating medium was maintained at a constant level (clamped) by continuously feeding fresh medium and removing depleted medium from a recirculation bottle. The flow rate of fresh medium to the system was typically 24 ml/h, and the volume of recirculating medium was 120 mL. During C experiments, 32 mM [1,6-C2]glucose was introduced into the feed to the system, and the recirculating glucose level was clamped at 26 mM by adjusting the feed rate. C NMR spectra were acquired with standard H decoupled NOE C acquisition on a 9.4 T/89 mm vertical bore Varian spectrometer (Varian Inc. Palo Alto, CA). The acquisition parameters were: 60° pulse angle, 1.2 s repetition rate, 25,000 Hz spectral width, 16,384 points, and 750 transients per spectrum. To ensure that H decoupling did not raise the temperature of the perfusate above 37.5oC, the temperature of the medium leaving the NMR tube was continuously monitored with a thermocouple. Free-induction decays were apodized by exponential multiplication (2 Hz) for signal to noise enhancement. Nuts NMR (Acorn NMR, Fremont, CA) was used to determine resonance peak areas. The cell n
更多查看译文
AI 理解论文
溯源树
样例
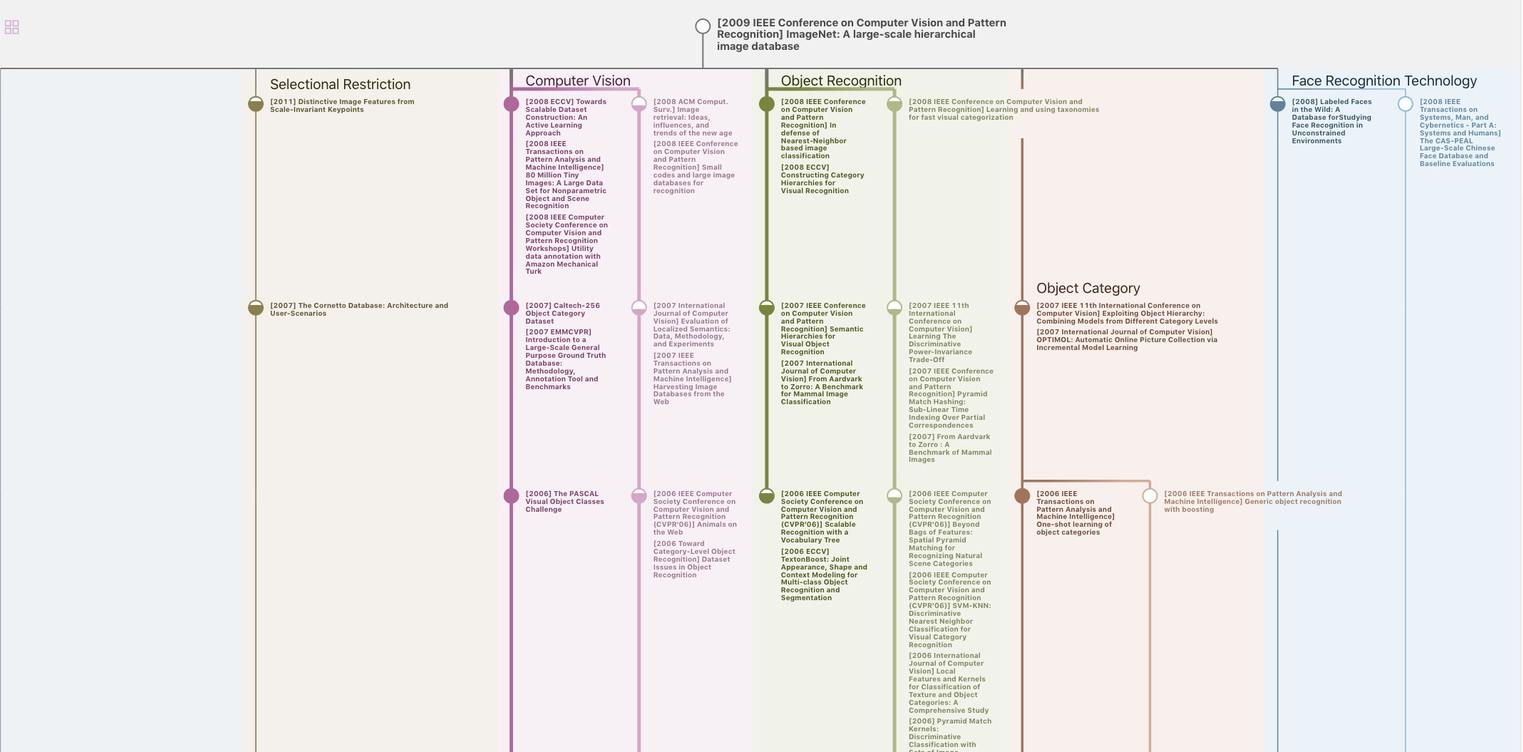
生成溯源树,研究论文发展脉络
Chat Paper
正在生成论文摘要