Magnetic properties of NdMn 1-xFexO 3 + system
semanticscholar(2012)
摘要
Magnetization, AC susceptibility and specific heat measurements were performed on polycrystalline NdMn1-xFexO3+ compounds (x = 0.0, 0.1 and 0.2). Substitution of Fe for Mn reduces the Néel temperature TN, which is associated with magnetic ordering of Mn sublattice, from 85.5 K to 57.0 K. The temperature dependence of magnetization for x = 0.2 exhibits the compensation temperature and the pole inversion below Tcomp = 26.7(5) K. Initial magnetization curves present a remnant magnetization in the ordered region with the sign of remnant magnetization depending on temperature. The substitution of Fe for Mn changes hysteresis loop of NdMnO3+ from the ferromagnetic-like one (0Hc = 0.09 T) by expanding the width of the loop (0Hc = 0.55 T) for x = 0.1 and then shrinking the central part of the loop to “butterfly” type (0Hc = 0.35 T) for x = 0.2. RETO3 compounds (RE = rare earth, T = 3d-metal) attract large attention for their very interesting physical properties e. g. multiferroism [1], ferroelectricity [2, 3], strange “butterfly-type” hysteresis loops [4], compensation temperatures [5, 6] and other effects. Substitution on the rare earth site or 3d metal site is the basic approach of tuning physical properties of these compounds. Up to now, the substitutions were done mainly on the RE-site by Na, K, Ca, Sr and Ba [7]. On the other hand, there is only scarce information about the doping on the 3d-metal site. In our work we study effect of Fe for Mn substitution on NdMn1-xFexO3+ polycrystalline compounds. Both parent compounds NdMnO3 and NdFeO3 adopt the orthorhombic crystal structure (space group Pnma). Nd atoms occupy the 4c position, Mn/Fe atoms occupy 4b and oxygen atoms occupy 4c and 8d crystallographic positions in the unit cell. In both compounds the Nd and Mn/Fe sublattices order magnetically [8, 9]. In the case of NdMnO3, the Mn sublattice orders at TN = 82 K with magnetic moments parallel to b-axis and Nd sublattice orders ferromagnetically (moments parallel to c-axis) at T1 20 K [8, 10]. On the other hand Fe sublattice of NdFeO3 orders above room temperature and undergoes spin reorientation transition in temperature range 70 – 160 K [9], but Nd sublattice orders only at very low temperatures (T1 1.5 K) [11]. Considering known magnetic properties of parent compounds one could suggest that Fe substitution for Mn in NdMnO3 will raise the TN to higher temperatures, but T1 will decrease. Up to now, the only studied Fe-Mn substitution compound was NdMn0.9Fe0.1O3 [6]. For this compound T1 14 K, which is lower than T1 for the NdMnO3 compound (agreement with expectations), but TN also decreases [6], which is in contradiction with expectations. These evidences imply that the concentration dependence of TN is not as straightforward as one can expect, and the more detailed study of Mn-Fe substitution has to be undertaken. Since this study has not been done before, we have prepared several compounds of the general formula NdMn1xFexO3+ and studied their magnetic and thermal properties. Our textured polycrystalline samples were prepared by floating zone method in a mirror furnace. As a starting materials we used high purity oxides of MnO2 (purity 3N, supplier: Alpha Aesar), Nd2O3 (purity 3N, supplier: Sigma Aldrich) and Fe2O3 (purity 2N, supplier: Sigma Aldrich). The starting materials were mixed, cold pressed into the rods and sintered at 1200 °C for 24 hours in air. The float zone experiment was performed using the Table 1. Lattice parameters of studied NdMn1-xFexO3+ compounds. x a (nm) b (nm) c (nm) V (nm3) 0 0.5843(2) 0.7546(3) 0.5415(2) 0.2387(7) 0.1 0.5807(2) 0.7572(3) 0.5415(2) 0.2381(7) 0.2 0.5774(2) 0.7602(3) 0.5421(2) 0.2380(7) EPJ Web of Conferences 40, 15007 (2013) DOI: 10.1051/epjconf/20134015007 © Owned by the authors, published by EDP Sciences, 2013 This is an Open Access article distributed under the terms of the Creative Commons Attribution License 2.0, which permits unrestricted use, distribution, and reproduction in any medium, provided the original work is properly cited. Article available at http://www.epj-conferences.org or http://dx.doi.org/10.1051/epjconf/20134015007 EPJ Web of Conferences flowing air (1 l/min) atmosphere. All samples which were used for the experiments were checked by X-ray powder diffraction and were proved to be single-phased. The obtained lattice parameters are summarized in Table 1. The oxygen content of all samples was checked by iodometric titration and was found small excess of oxygen, = 0.035, 0.095, 0.065 for x = 0, 0.1, 0.2, respectively. We have also tried to prepare samples in Ar atmosphere (ambient pressure and pressure of 8 bar), but we have observed the reduction of Mn ion into the Mn ion and thence the MnO impurities in the grown ingots. Magnetization (M) and susceptibility () data were measured using the SQUID magnetometer from Quantum Design with RSO option. The samples were prepared by powdering the grown ingot. The sample’s typical mass was around 6 mg. The heat capacity (HC) data were measured on bulk, highly textured samples directly cut from the grown ingot. The sample’s mass was between 8 and 14 mg. For HC measurements we have used Physical Property Measurement System (Quantum Design). Temperature dependence of HC (Fig. 1) clearly exhibits lambda-like anomaly at 85.1(2) K for NdMnO3+ and at 74.8(2) K for NdMn0.9Fe0.1O3+. Taking into account data from neutron diffraction [8, 10] these anomalies indicate the ordering of Mn sublattice into type-A antiferromagnetic structure. HC shows additional large anomaly below 25 K which was attributed by other authors to Schottky-type contributions [12, 15]. Contrary to this, magnetization measurement revealed a larger ferromagnetic component at low temperature which cannot be explained reasonably by the canting of the AAF structure of Mn spins [13], and neutron diffraction experiments [8, 10] suggest ferromagnetic ordering of Nd sublattice at this temperature. Substitution of Fe for Mn leads to shift of the anomaly at T1 to higher temperature. In order to estimate magnetic part of the specific heat (Cmag) we have subtracted phonon contribution using heat capacity data of NdGaO3 nonmagnetic analogue. The specific heat data for this compound were presented by Schnelle et al. [14]. The resulting Cmag vs. T curve (Fig. 2) clearly shows that the low temperature bump has the maximum at 10.5(5) K for NdMnO3+ and at 12.5(5) K for NdMn0.9Fe0.1O3+ (Fig. 2). This bump is consistent with the specific heat data published previously [12, 15]. The calculated magnetic entropy saturates for both compounds reaching the value Rln7 and Rln6 (R = gas constant) for NdMnO3+ and NdMn0.9Fe0.1O3+, respectively (insert of Fig. 2). AC susceptibility measurements for both in phase part (’) and out phase part (’’) of susceptibility are displayed in Fig. 3. These measurements revealed that antiferromagnetic transition decreases with Fe doping, shifting TN from 85.5(2) K to 74.9(2) K and to 57.0(3) K for x = 0.0, 0.1 and 0.2, respectively. The anomaly of ’(T) at T1, which is associated with Nd sublattice, becomes more pronounced and for smaller doping (x = 0.1) shifts to higher temperature from 10 K to 16 K and then decreases to 13 K for x = 0.2. These results are again 0 50 100 150 200 0 20 40 60 80 100 NdMnO3+δ NdMn0.9Fe0.1O3+δ C (J m ol -1 K 1 )
更多查看译文
AI 理解论文
溯源树
样例
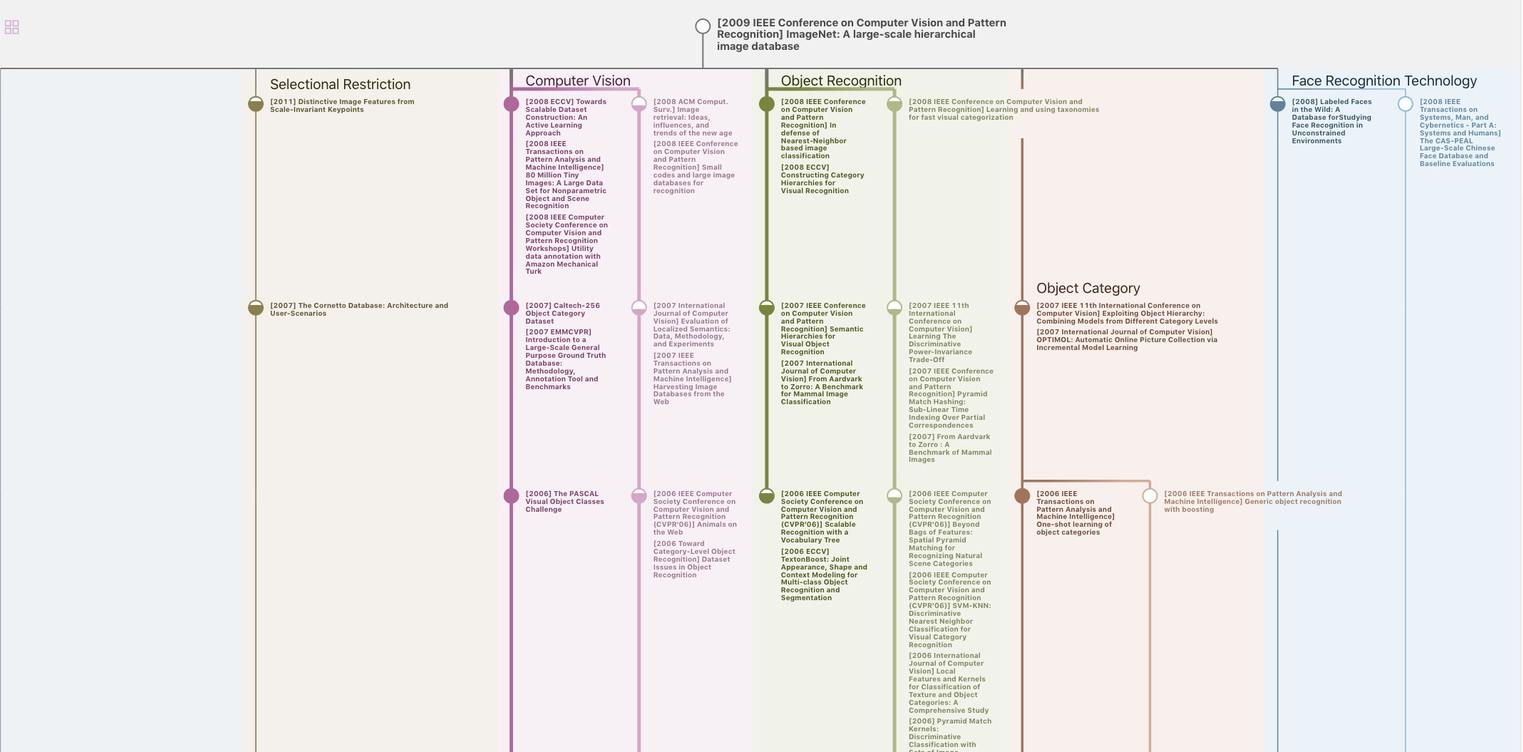
生成溯源树,研究论文发展脉络
Chat Paper
正在生成论文摘要