Flavivirus NS 1 crystal structures reveal a surface for membrane association and regions of interaction with the immune system
semanticscholar(2014)
摘要
Flaviviruses, the human pathogens responsible for dengue fever, West Nile fever, tick-borne encephalitis and yellow fever, are endemic in tropical and temperate parts of the world. The flavivirus non-structural protein 1 (NS1) functions in genome replication as an intracellular dimer and in immune system evasion as a secreted hexamer. We report crystal structures for full-length, glycosylated NS1 from West Nile and dengue viruses. The NS1 hexamer in crystal structures is similar to a solution hexamer visualized by single-particle electron microscopy. Recombinant NS1 binds to lipid bilayers and remodels large liposomes into lipoprotein nanoparticles. The NS1 structures reveal distinct domains for membrane association of the dimer and interactions with the immune system, and are a basis for elucidating the molecular mechanism of NS1 function. Flaviviruses have a positive-sense RNA genome that encodes a single viral polyprotein. The polyprotein is inserted into the ER membrane through several signal sequences and processed by viral and host proteases into three structural and seven non-structural proteins (NS1, NS2A, NS2B, NS3, NS4A, NS4B, NS5) (1). Six of the non-structural proteins (NS2A – NS5) form a replication complex on the cytoplasmic side of the ER membrane where the NS3 and NS5 enzymes function at a scaffold created by the other four trans-membrane proteins. The remaining protein, the conserved glycosylated NS1, is associated with lipids, both early in infection, where intracellular dimeric NS1 localizes on the ER membrane at the site of viral RNA replication, and late in infection, where secreted hexameric NS1 lipoprotein particles interact with components of the complement-mediated immune system (2, 3). NS1 is essential for replication of the flavivirus genome (4–8), possibly through interactions with transmembrane proteins NS4A and NS4B (5, 9). Electron microscopy Correspondence to: JanetSmith@umich.edu. †These authors contributed equally to this work. NIH Public Access Author Manuscript Science. Author manuscript; available in PMC 2015 February 21. Published in final edited form as: Science. 2014 February 21; 343(6173): 881–885. doi:10.1126/science.1247749. N IH -P A A uhor M anscript N IH -P A A uhor M anscript N IH -P A A uhor M anscript (EM) studies of secreted NS1 (sNS1) identified a symmetric barrel-shaped hexamer that carries a cargo of ~70 lipid molecules (10, 11). NS1 interacts with multiple components of both the innate and adaptive immune systems, (12–14) is involved in immune system evasion and pathogenesis, (12–15) and is the major antigenic marker of viral infection (16). While the role of NS1 in multiple stages of the virus life cycle is well established, little is known of the molecular mechanisms of its various functions. The lack of sequence identity to any protein of known structure and the difficulty of producing pure, stable protein have hindered progress in understanding the roles and mechanisms of NS1. An understanding of NS1 structure will help to sort out these contradictory results and will facilitate more efficient vaccine development. We produced recombinant, full-length, glycosylated West Nile virus (WNV) and dengue virus type 2 (DEN2) NS1 in insect cells using a baculovirus expression system. Despite the presence of a secretion signal in the expression construct, nearly all NS1 was retained by cells and partitioned with the membrane fraction. Soluble NS1 was released by mild detergent treatment of the membrane fraction and appeared as a dimer by gel filtration chromatography, in agreement with direct visualization of particles by negative-stain electron microscopy (EM) (Fig. S1A, B). Multiple chromatography steps without detergent shifted the oligomeric state to a hexamer, presumably due to removal of bound detergent (Fig. S1C, D). WNV NS1 crystallized in two forms and DEN2 NS1 in one form. The WNV NS1 dimer structure was solved from the anomalous scattering of the native sulfur atoms (12 Cys and 5 Met per subunit) using high-multiplicity (~100 fold) data acquired from 18 crystals of form 1 (Table S1, Fig. S2A, B). The twelve cysteines form six disulfide bonds within the NS1 monomer. Three asparagines are glycosylated (Asn130, Asn175 and Asn207), each with clear electron density for one to five sugar residues (Fig S2C). The structure is complete for all amino acids with the exception of one internal loop (amino acids 108 – 128). Identical dimer structures occur in WNV and DEN2 NS1 (0.58 Å root-meansquare deviation of 576 Cα atoms). The NS1 dimer is constructed around an extended central β-sheet domain (Fig. 1A, B, Fig S3). Each monomer has three domains. A small “β-roll” dimerization domain (amino acids 1-29) is a mini domain-swap structure of two β-hairpins, each stabilized by a disulfide (Cys4-Cys15). The β-hairpins extend across the dimer axis and intertwine to form a fourstranded β-sheet that curves into a roll-like structure (Fig. 1A, blue; Fig. S2D, E). The second domain (amino acids 30-180) of each monomer protrudes from the central β-domain like a wing (Fig. 1A, yellow). Each “wing” domain contains two glycosylation sites (Asn130, Asn175), an internal disulfide (Cys55-Cys143), and two discreet subdomains. An α/β subdomain (amino acids 38 – 151) comprises a four-stranded β-sheet, two α-helices and a disordered distal tip (amino acids 108 – 128; Fig. 1A, dotted line). A discontinuous connector subdomain,(amino acids 30 – 37 and 152 –180) packs against the β-roll (Fig. 1A, orange) and also links the wing to the central β-sheet domain through a disulfide (Cys179Cys223). The predominant structural feature of NS1 is the third domain, a continuous βsheet that extends along the length of the dimer with its 18 β-strands arranged like the rungs of a ladder (Fig. 1A, red). This core “β-ladder” domain is formed by the C-terminal half of NS1 (amino acids 181 – 352), in an arrangement where each monomer contributes nine rungs to the anti-parallel β-ladder. In a simple (+1) topology, the first five β-strand rungs of Akey et al. Page 2 Science. Author manuscript; available in PMC 2015 February 21. N IH -P A A uhor M anscript N IH -P A A uhor M anscript N IH -P A A uhor M anscript each monomer begin at the dimer interface and proceed sequentially towards the end of the ladder (Fig. 1B). Most of the inter-strand loops are short with the notable exception of a long “spaghetti loop” between β13 and β14, (amino acids 219 – 272) that lacks secondary structure but is ordered by 57 hydrogen bonds (Fig. 1C, pink). A conserved tip region (Fig. S3) at each end of the β-ladder domain (amino acids 278 – 352) contains four strands of the central β-ladder, a small three-stranded β-sheet and three disulfides. The overall dimensions of the NS1 dimer are 90 Å along the length of the β-ladder, 90 Å in width from wingtip to wingtip and 40 Å in the third dimension (Fig. 1A). The β-ladder defines a plane through the NS1 dimer with the β-roll domain on one side (Fig. 1C). On the other side of the plane are the spaghetti loop, the glycosylation sites, the wing domain disordered loop, and the C-terminus, which, prior to proteolytic cleavage, is fused to the >20-residue lumen-side N-terminus of viral protein NS2A. The β-roll and connector subdomain of the wing create a protrusion with a strikingly hydrophobic surface on one face of the dimer (Figs 1C, 2A). Extra electron densities at this surface were evident in the earliest maps, and were fit as parts of three detergent molecules after all regions of the polypeptide had been assigned (Fig. S2D, E). The hydrophobic character of the β-roll/connector protrusion is conserved (Fig. S3, S4). Furthermore, a dipeptide (Arg10-Gln11) implicated in interaction with the transmembrane protein NS4B (9) is located at the periphery of the hydrophobic surface in a loop of the β-roll (Fig. S2E). Thus the hydrophobic protrusion is a strong candidate for dimeric NS1 interaction with the ER membrane and with the replication complex through transmembrane proteins NS4A/B, where NS1 plays a poorly defined but essential role in viral replication. We investigated the ability of recombinant WNV NS1 to interact with membranes by incubating purified protein with liposomes and imaging the mixture by negative-stain EM. Upon exposure to NS1, the large heterogeneous liposomes were not only coated with NS1, presumably in its dimeric form, but also were converted into much smaller lipid-protein nano-particles (Fig. 2B, Fig. S5). This unexpected ability to interact with and re-model membranes may have implications for the role of NS1 in organizing replication complexes or in conversion from a membrane-associated dimer in the ER lumen to a secreted proteolipid hexamer. A “greasy finger” loop on the connector subdomain forms a prominent part of the hydrophobic protrusion and is mobile, as it is disordered in the DEN2 NS1 structure. Mutations to this conserved region (Gly159-Phe-Gly-Val162) were deleterious to virus replication as measured by plaque assay (Table S2). Substitution of charged amino acids in DEN2 NS1 (F160D and V162D) or the double substitution G159A/F160A resulted in no detectable plaques. A single substitution (F160A) impaired virus viability and RNA synthesis (Fig. S6). Purified NS1 variants with these substitutions re-modeled liposomes similarly to the wild type (Fig. S6D), suggesting that the observed phenotype is due to loss of effective interactions with transmembrane proteins of the replication complex. Intracellular NS1 is thought to be predominantly dimeric whereas secreted NS1 is a soluble, hexameric proteolipid particle (3, 10, 11). Lipid-free recombinant NS1 occurred in both Akey et al. Page 3 Science. Author manuscript; available in PMC 2015 February 21. N IH -P A A uhor M anscript N IH -P A A uhor M anscript N IH -P A A uhor M anscript dimeric and hexameric forms in solution (Fig. S1), but in all three crystal structures, NS1 exists in a hexameric arrangement of
更多查看译文
AI 理解论文
溯源树
样例
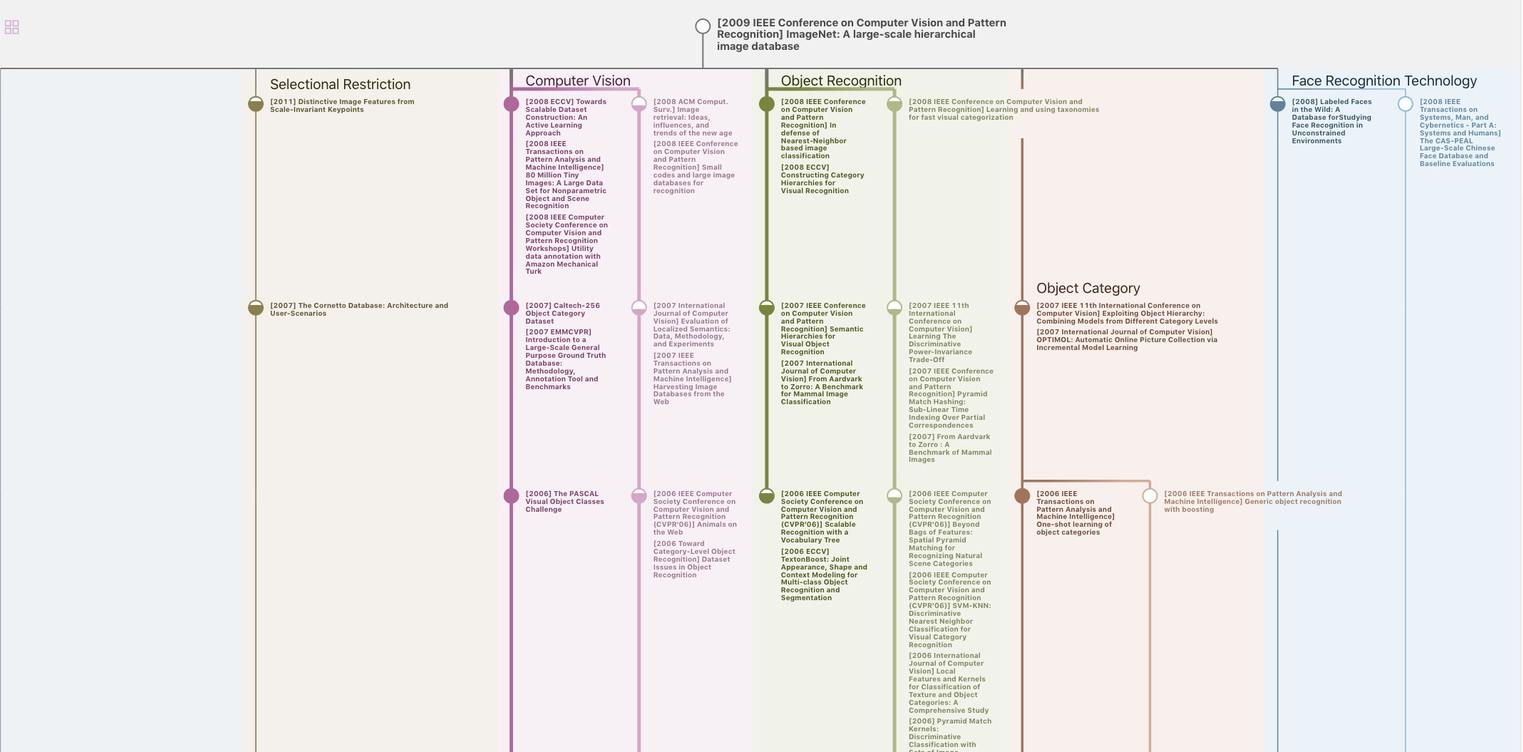
生成溯源树,研究论文发展脉络
Chat Paper
正在生成论文摘要