Title: Molecular characterization of latent GDF8 reveals mechanisms of activation Running title: Activation of latent GDF8
semanticscholar(2017)
摘要
Growth/differentiation factor 8 (GDF8) or myostatin negatively regulates muscle mass. GDF8 is held in a latent state through interactions with its N-terminal prodomain, much like TGF-β. Using a combination of small angle X-ray scattering and mutagenesis, we characterized the interactions of GDF8 with its prodomain. Our results show that the prodomain:GDF8 complex can exist in a fully latent state and an activated or ‘triggered’ state where the prodomain remains in complex with the mature domain. However, these states are not reversible, indicating the latent GDF8 is ‘spring-loaded’. Structural analysis shows that the prodomain:GDF8 complex adopts an ‘open’ configuration, distinct from the latency state of TGF-β and more similar to the ‘open’ state of Activin A and BMP9 (non-latent complexes). We determined that GDF8 maintains similar features for latency, including the alpha-1 helix and fastener elements, and identified a series of mutations in the prodomain of GDF8 that alleviate latency, including I56E, which does not require activation by the protease Tolloid. In vivo, active GDF8 variants were potent negative regulators of muscle mass, compared to wild-type GDF8. Collectively, these results help characterize the latency and activation mechanisms of GDF8. Introduction One of the most thoroughly described negative regulators of skeletal muscle mass is the TGF-β superfamily ligand growth/differentiation factor 8 (GDF8), also known as myostatin (McPherron & Lee, 1997a; 1997b). Genetic disruption of GDF8 results in substantial skeletal muscle growth (Grobet et al, 1997; Kambadur et al, 1997; McPherron & Lee, 1997a; 1997b; Schuelke et al, 2004; Clop et al, 2006; Mosher et al, 2007). Further, a significant increase in muscle fiber size is also observed when adult animals are treated with agents that bioneutralize GDF8 (reviewed in (Lee, 2010)). As such, targeted inhibition of GDF8 is currently being pursued for the treatment of skeletal muscle-related disorders and associated symptoms (Suragani et al, 2014; Lach-Trifilieff et al, 2014). GDF8, like numerous TGF-β family members, is a disulfide-linked dimer that is synthesized as a precursor protein which requires cleavage by a furin-like protease to yield a N-terminal prodomain and a Cterminal mature, signaling domain (Lee & McPherron, 2001). Interestingly, for a number of TGF-β ligands the role of the prodomain extends beyond ligand maturation and folding support (Gray & Mason, 1990; Harrison et al, 2011), remaining non-covalently associated with the mature ligand following secretion in either a lowaffinity, non-inhibitory or high-affinity, inhibitory fashion (reviewed in (Hinck et al, 2016)). For example, the prodomains of TGF-β1, TGF-β2, TGF-β3, GDF11, and GDF8 hold the mature ligand in a latent or inactive state mediated by a non-covalent, yet high affinity, ligand-specific interaction (Lee & McPherron, 2001; Thies et al, 2001; Zimmers et al, 2002; Hill et al, 2002; 2003) whereas mature Activin A and BMP9 remain associated with, but are not inhibited by, their prodomain (Mi et al, 2015; Wang et al, 2016). Activation of TGF-β1 and TGF-β3 requires covalent interactions with the extracellular matrix and cellular contractile forces to release the mature ligand (Annes et al, 2004; Wipff & Hinz, 2008; Shi et al, 2011). In fact, resolution of the latent TGF-β1 crystal structure provided a molecular explanation for how latency is exerted by the prodomain via a coordinated interaction between the N-terminal alpha helix (alpha1), latency lasso, and fastener of the prodomain with type I and type II receptor epitopes of the mature domain (Shi et al, 2011). On the other hand, GDF8 activation requires a second cleavage event within the prodomain via proteases from the BMP1/Tolloid (TLD) family of metalloproteases (Wolfman et al, 2003). However, the molecular and structural details of the GDF8 latent state have yet to be determined. Based on sequence conservation and prior biochemical data describing the N-terminal portion of the GDF8 prodomain (Thies et al, 2001), it is plausible that the molecular interactions and overall structure of the GDF8 latent complex may be similar to that of TGF-β1. However, the prodomains of a number of TGF-β family members share similar sequence conservation, yet they do not regulate the mature ligand in the same fashion and also exhibit significant structural diversity (Mi et al, 2015; Wang et al, 2016). Therefore, while one might expect that GDF8 and TGF-β1 would share certain elements for how the prodomain binds and confers latency, it is possible that significant structural and molecular differences in these interactions occur as they exhibit profoundly different mechanisms of activation. However, this comparison is hindered by a lack of understanding of the GDF8 latent complex at the molecular level. In this study, we utilized small angle X-ray scattering (SAXS) and mutagenesis to characterize the GDF8 latent complex. Interestingly, SAXS analysis reveals that the GDF8 latent complex adopts a more ‘open’ conformation, similar to the overall structure of the BMP9 and Activin A prodomain complexes, which are not latent. The ‘open’ conformation of the GDF8 latent complex is in stark contrast to the ‘closed’ conformation adopted by the TGF-β1 latent complex. Furthermore, we identify key residues in the GDF8 prodomain that are responsible for promoting latency indicating that GDF8 and TGF-β1 share similar features for latency including a latency lasso. We further show that certain mutations in the prodomain of GDF8 can reduce latency, producing a more active ligand both in vitro and in vivo. Overall, our data provide insight toward the molecular mechanisms of GDF8 latency and activation. Results Prodomain-GDF8 can exist in a latent and active complex. Initial characterization in adult mice showed that GDF8 is secreted into the systemic circulation as a latent protein complex that requires activation to trigger GDF8 signaling (Zimmers et al, 2002). While the biological mechanism for activation remained unknown, it was shown that a GDF8-specific signal derived from the serum of a wild-type mouse, but not a gdf8 mouse, could be detected following exposure to acidic conditions, referred to here as “acid-activation” (Zimmers et al, 2002). The premise for acid-activation stemmed from a similar observation that was made during the characterization of TGF-β, which is similarly regulated by its prodomain, (Lawrence et al, 1985; Schultz-Cherry & Murphy-Ullrich, 1993) and provided the initial basis that latent GDF8 and latent TGF-β are likely to be very similar in terms of activation and prodomain release. While a molecular basis to describe how acid-activation alleviates ligand latency remains unknown, it is thought that the acidic-conditions simply dissociate the prodomain from the mature domain, thereby freeing the ligand from inhibition (Zimmers et al, 2002). However, our initial attempts to purify the mature domain from the prodomain after acid-activation using an affinity column to the high-affinity antagonist, follistatin, failed, even though the complex exhibited significant activity. This observation suggested that perhaps the prodomain remained bound to the mature domain, but was not in a fully inhibitory state. To extend these initial observations, we isolated the mammalian-derived latent proGDF8 complex (GDF8) and compared its signaling activity to both the acid-activated state (GDF8) and to the mature, unbound GDF8 (GDF8) using a SMAD3-responsive (CAGA)12 luciferase reporter HEK293 cell line (Cash et al, 2012a; 2012b; 2013; Walker et al, 2015; 2017). As expected and consistent with our previous report, GDF8 readily signaled with a calculated half-maximal effective concentration (EC50) of 0.72 nM (Walker et al, 2017), whereas media containing GDF8 did not readily signal and required nearly 10,000-fold more protein to achieve a similar response as compared to GDF8 (Figure 1a). In contrast, acid-activation of media containing GDF8 at pH 2 to generate GDF8 resulted in a significant gain in activity compared to non-acid activated latent GDF8 (Figure 1a). Interestingly, the calculated EC50 for GDF8 (5.7 nM) still did not reach the EC50 of GDF8, suggesting that under these conditions we were unable to observe the full signaling potential of mature GDF8. Since GDF8 is stable and stored in 10 mM HCl, we do not expect this difference in activity to be caused by subjecting GDF8 to extreme conditions. We next evaluated the activation of proGDF8 as a function of pH by subjecting GDF8 to various pH ranges (pH 2-10) for 1 hour, followed by neutralization and (CAGA)12 activation (Figure 1b). We determined that at the concentration tested (40 nM), the level of activation increases with a decrease in pH (Figure 1b), however substantial activation was observed throughout the pH range examined. The shape of the titration experiment indicated that multiple ionizable groups could be involved in the latency mechanism or that shifts in the pH cause disruption in the structure of the prodomain that effects its ability to inhibit GDF8. Given that titration of the GDF8 prodomain against mature GDF8 results in potent ligand inhibition (Thies et al, 2001), we hypothesized that we were unable to recover the full signal from acid activated latent GDF8 due to the possibility that the prodomain may still be able to provide some level of antagonism, through a non-covalent interaction, despite being acid activated. To test this hypothesis, we subjected GDF8 and GDF8 to size exclusion chromatography (SEC) followed by SDS-PAGE/Coomassie staining. In addition, we combined isolated GDF8 prodomain with GDF8 and applied the mixture to SEC. We determined that all three variations of GDF8 complexes had similar retention volumes with both components (co-eluting the prodomain and GDF8), indicative of complex formation (Figure 1c). This result supports the idea that during the acid activa
更多查看译文
AI 理解论文
溯源树
样例
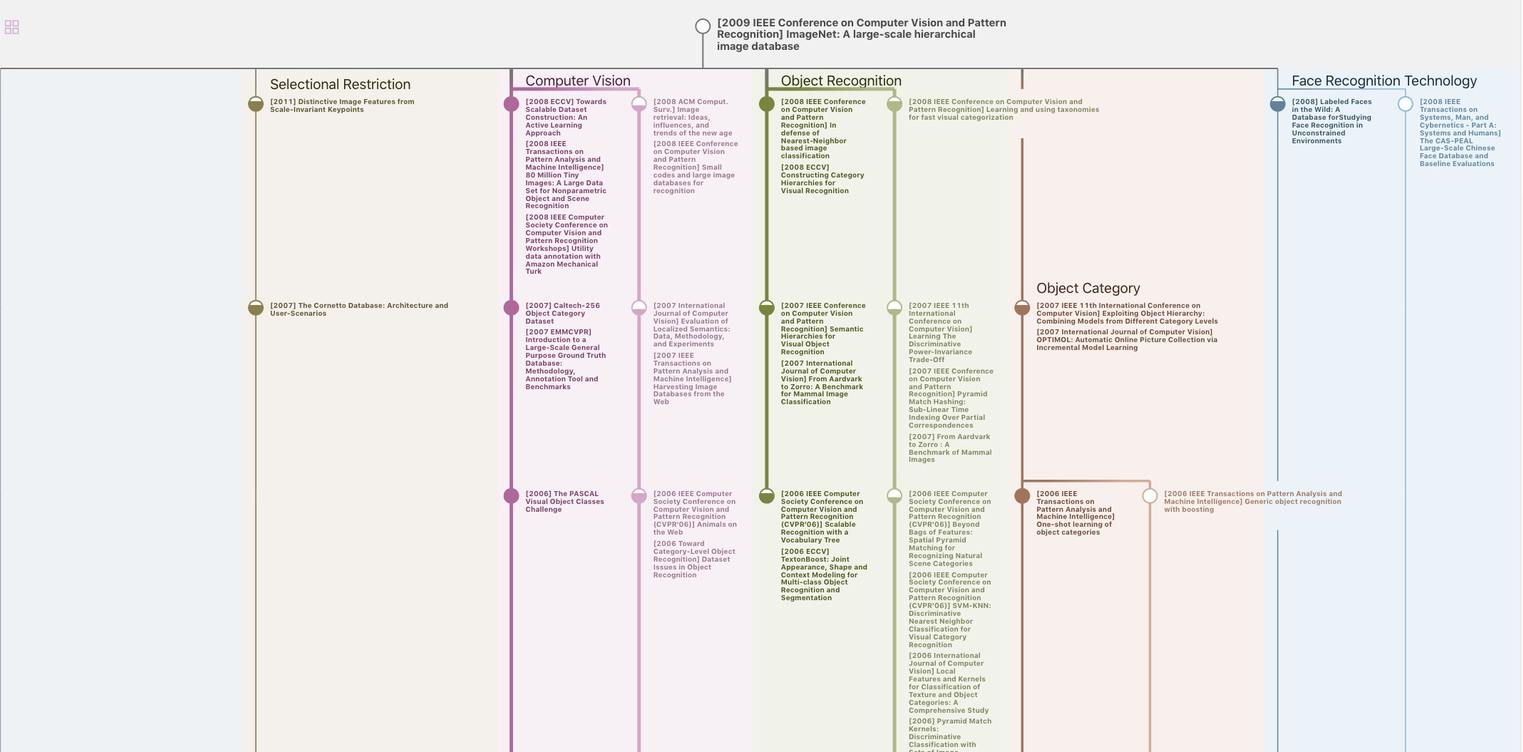
生成溯源树,研究论文发展脉络
Chat Paper
正在生成论文摘要