Establishing the Feasibility of a Sensor-Based Sock Management System
JOURNAL OF MEDICAL DEVICES-TRANSACTIONS OF THE ASME(2016)
Abstract
One of the leading problems that amputees have with their prosthetic limbs is improper socket fit [1]. An improper fit can be caused by the physiology and dynamic nature of the residual limb, which causes its volume to fluctuate over time. This volume fluctuation can occur over the course of several months due to muscle atrophy or over the course of several hours due to fluid changes with increased or decreased activity. One common cost-efficient way to accommodate for the change in residual limb volume is the use of residual limb socks. Amputees restore socket fit by adding or removing socks throughout the day, depending on how their residual limb changes. A challenge arises, however, when determining the correct number of socks to wear. Many amputees who have neuropathy or who are inexperienced with sock management have difficulty determining when to add or remove socks based on the volume of their limb. Having too many socks or too few socks creates an improper socket fit and can lead to instability, discomfort, skin breakdown, and pain for the amputee [1].The aim of this paper is to explore different sensor locations within a socket that can be used to effectively classify a good socket fit. Finding a sensor location that can be used to discriminate between different socket fits will determine the feasibility of a sensor-based system that would help amputees know when to add or remove residual limb socks. Sock management would help amputees maintain proper socket fit and prevent skin breakdown of the residual limb.The first step of this study was to create a residual limb and socket model to use for testing. A prosthetic socket was provided by the Minneapolis Veterans Affairs Health Care System's (MVAHCS) Prosthetics Department. Silicone rubber (Oomoo 25, Smooth-On, Macungie, PA) was poured into the socket and allowed to set to create the residual limb model. The prosthetists then digitized the residual limb model, increased the model by 5-ply, and made a new socket with the 5-ply fit. The fit was verified by the prosthetists as the “proper” fit for the model. The classification of a proper fit provided the baseline to which we would compare force changes in the socket when the number of socks on the residual limb deviated from the ideal 5-ply.The next step was to identify key locations within the socket that would be ideal for placing the sensors. A single sensor (ELF FlexiForce, Tekscan, Boston, MA) was used in this study. The force sensor is 0.203 mm thick and 228.8 mm long with an active sensing area of 9.53 mm. Its size and flexibility were ideal for placing it in a socket to measure force. The sensor could measure forces between 0 and 111 N. Several trials were done to determine the best locations for placement of the sensor. These trials involved placing the residual limb model in the socket and recording force measurements with different sock fittings. We tested six locations within the socket and chose four as the best for use in this project (Fig. 1). These locations were selected based on accessibility, repeatability, and sensitivity to changes in sock ply. Location #1 was marked on the anterior distal part of the socket, near the tibial end. Location #4 was marked at the patellar tendon bar with location #2 proximal to it. Location #5 was placed at the distal end of the socket. The two locations that were excluded from this study were location #3 at the fibular head and location #6 at the popliteal depression.The next step was to measure the forces at the different locations under a static condition. We placed the sensor in the socket at location #1 and secured it using tape. We then attached the residual limb model to a steel plate to provide an area where weight can be applied to simulate standing. The residual limb model was placed in the socket. We then pressed on to the steel plate and applied 444.8 N (100 lbs) of force. We monitored the force being applied by placing a scale under the socket. We waited for the force readings to stabilize and then removed the load. Force readings from the sensor were recorded at a sampling rate of 8 Hz. We recorded the average force across each trial. This process was used to record three trials at each identified sensor location, under four different sock conditions: no socks, 3-ply, 5-ply, and 7-ply. The 7-ply sock condition was a combination of a 5-ply sock and two 1-ply socks. The 3- and 5-ply socks were made out of acrylic (Royal Knit, Lee's Summit, MO), and the 1-ply socks were made out of cotton (Knit Rite, Kansas City, KS). These socks were chosen based on the brands that the prosthetists' patients commonly used.The average force recorded by the sensors under each condition at the four locations is represented in Fig. 2.At location #1, the highest forces were recorded at 3-ply and 5-ply. The two extreme conditions, 0-ply and 7-ply, had average forces that were similar and lower than the other two conditions. At location #2, the force decreased with increasing ply. The no-ply condition recorded the highest force, while the 7-ply recorded zero force across all the trials at that location. Location #4 had the opposite trend of location #2. Force increased as we dressed the limb model with more sock ply. When there were no socks on the limb model, it was loose in the socket and did not make full contact with the sensor. However, increasing the sock ply tightened the fit and explains why there were higher forces measured. The 7-ply average force at this location was significantly higher than any other force we recorded.Location #5 had a similar trend to location #2 with force decreasing as more sock ply was added to the limb model. When the limb model had no socks or too few sock plies, it would slide into the socket more easily and push up against the distal end. As more ply was added, it was harder to get the limb into the socket and ensure that it was making contact with the distal end. The 3-ply and 5-ply average forces were more distinct, and the 0-ply had a force reading slightly above 0 N.Based on the trends we observed from Fig. 2, we see that the best location to place a sensor within a socket is at location #4. There was a strong correlation between increasing sock ply and average force readings recorded. Additionally, the average force readings for the different ply conditions were the most distinct at this location. The trends we observed are consistent with the literature. Location #4, the patellar tendon bar, is a feature in sockets used as a load-bearing mechanism. The added ply increased the contact between the residual limb model and the patellar tendon bar (PTB). When the PTB bears load, it relieves other areas of the socket from pressure [2]. As the force at location #4 increased, it decreased at location #2. Location #2 was good for distinguishing between the highest and lowest ply conditions. However, the difference in 3-ply and 5-ply average forces was very subtle at this location. It would be even harder to discriminate between those two fits at location #1. The average forces were within standard deviations of each other at both of these locations. In addition to lack of differentiation between fits, location #1 would not be suitable for sock management because it did not display a consistent trend between adding more ply and average force recorded.Location #5 would be another suitable location to place a sensor to help with sock management. Similar to location #4, there was discriminability between the different fits. There was also a strong correlation with adding more ply and average force.Figure 2 shows that the error was small across most trials. Large errors were mostly present in the trials that had no ply. We can credit this to the fact that with fewer socks, the residual limb sits more loosely in the socket.The biggest source of inconsistency was when we were applying weight on the limb model. The weight applied, as indicated by the scale, fluctuated around the target value. For further testing, we would use a force-machine to ensure that the force we apply is consistent.The ultimate goal of this research was to establish the feasibility of a sensor-based sock management system. Given promising results, a system could then be developed to help amputees know when they need to add or remove socks from their residual limb. In order for this system to be effective, it would need to recognize a deviation from the set baseline of fit and force, as established by a prosthetist. Additionally, the device would have to be easy to use by the amputee and convenient to place in a socket.We believe that the results of this study provide insight into possible sensor locations that can be adopted in such a system. Further work in this area will explore dynamic conditions and how forces at these locations change during simulated walking. We would also like to look at the possibility of using information from multiple sensors simultaneously to distinguish between socket fits.This project was supported by the University of Minnesota's Undergraduate Research Opportunities Program.We would like to thank MVAHCS prosthetists Kyle Barrons and Spencer Mion for their input and suggestions throughout this study and resident prosthetist Kathryn Blaharski for helping with the digitization of the limb model.
MoreTranslated text
Key words
sock management system1,sensor-based
AI Read Science
Must-Reading Tree
Example
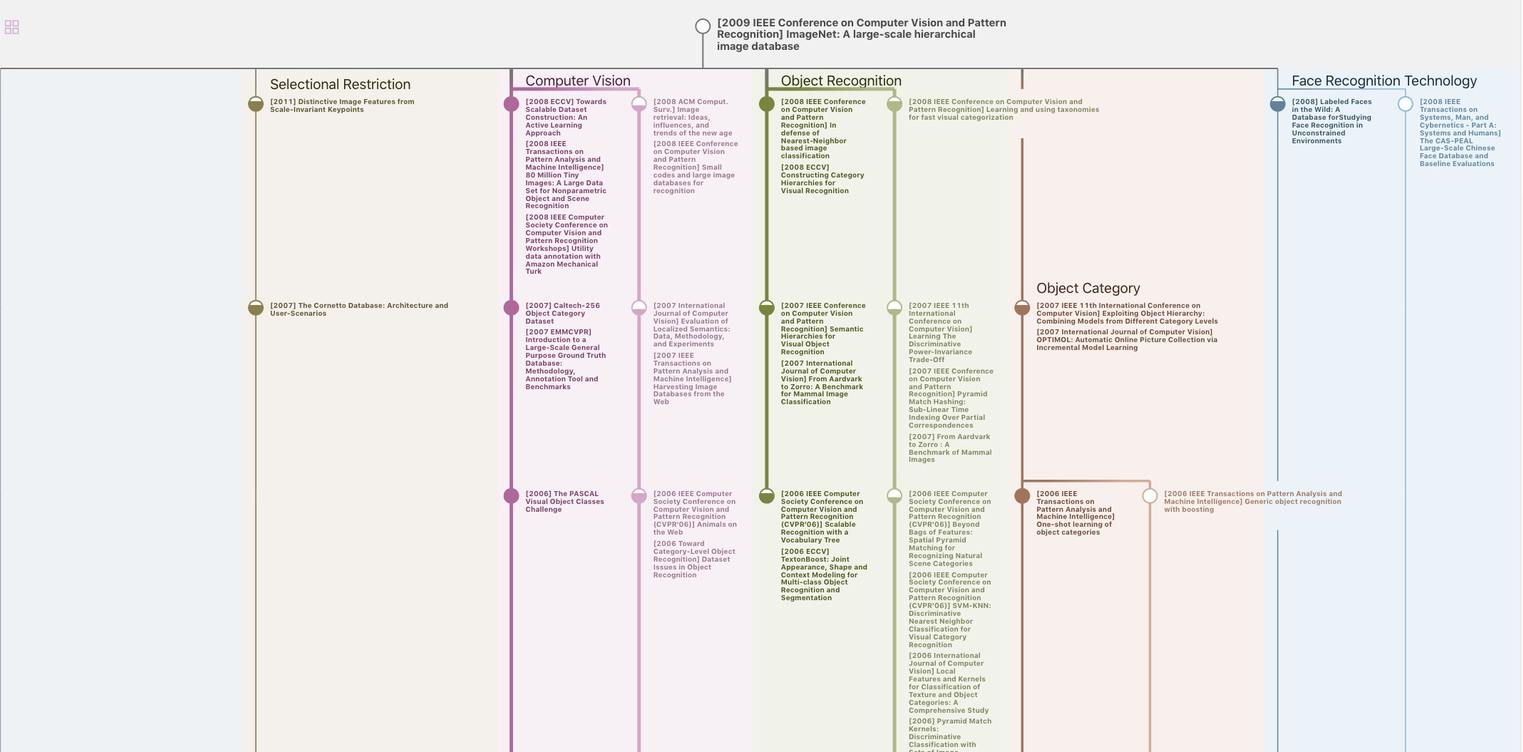
Generate MRT to find the research sequence of this paper
Chat Paper
Summary is being generated by the instructions you defined