Lithium Sulfide/Metal Nanocomposite as a High-Capacity Cathode Prelithiation Material
ADVANCED ENERGY MATERIALS(2016)
Abstract
DOI: 10.1002/aenm.201600154 due to their limited specific capacity.[18,19] Very recently, we have proposed that conversion reaction materials can be attractive high capacity prelithiation materials for cathodes and demonstrated using Li2O/metal and LiF/metal nanocomposites.[20,21] For example, a high “donor” lithium-ion specific capacity of 609 mAh g−1 has been achieved for a stable nanoscaled mixture of Li2O and Co in the cutoff potential range of current cathodes.[20] Herein, we would like to explore the potential of Li2S and metal nanocomposites for cathode prelithiation. We believe that having a new set of lithium compounds beyond Li2O for cathode prelithiation is important. Li2S possesses a high theoretical specific capacity of 1166 mAh g−1 and can be paired with lithium free anodes (e.g., graphite, Sn, and Si). It has been widely investigated as one of the best cathode candidates for the next-generation rechargeable lithium batteries and significant achievements have been demonstrated.[22–26] Here we propose that Li2S can be used as a high-capacity cathode prelithiation material for the existing LIBs. To this end, four significant challenges need to be overcome: (1) The compatibility of electrolytes. Previous investigations on pristine Li2S and S particles for cathodes were usually carried out in ether-based electrolytes instead of the widely used carbonate-based electrolytes in commercial LIBs.[4,22,27,28] Because carbonate-based solvents chemically react with the intermediate polysulfides, such solvents are inappropriate for S and Li2S cathodes.[29] (2) The compatibility of working potential. The widely used cathode materials usually have a cutoff charge potential higher than 4.2 V versus Li+/Li (e.g., 4.3 V for LiCoO2, LiNixCoyMnzO2, and LiMnO4, and 4.2 V for LiFePO4) and a cutoff discharge potential higher than 2.5 V (e.g., 2.5–3 V) in the carbonate-based electrolytes. To maximize the “donor” lithium-ion capacity, relative low delithiation (e.g., u003c4.2 V) and lithiation potentials (e.g., u003c2.5 V) are desirable for a cathode prelithiation material, so that all the stored lithium can be irreversible extracted during the first charge/discharge cycle. (3) The diffusion of the intermediate polysulfides and low conductivity of Li2S. Due to the loss of polysulfide active material into the electrolyte, it is challenging to maximize the lithium-ion capacity. Additionally, it is hard to extract lithium from Li2S due to its insulating nature. (4) The instability under ambient and battery fabrication conditions. Li2S is highly reactive with moisture in air. Such property makes it incompatible with ambient and industrial battery fabrication conditions. Despite the great knowledge obtained from the previous work on Li2S-based cathode material, the solutions to this issue remain to be developed. Therefore, it is highly desirable to solve these inherent problems of Li2S via a rational structure and component design to pave the path for its application as Two approaches to increase specific energy and energy density of batteries include the exploration of new battery chemistries and the continuous improvement of the existing lithium-ion battery (LIB) chemistries.[1,2] The exploration of new highcapacity electrode materials, such as Si for anodes and S/Li2S for cathodes, has recently become an important focus of materials research and will potentially revolutionize energy storage technologies.[3,4] At the same time, the improvement of the existing LIB systems could also offer more practical avenues and faster performance increment in the near future. Accompanied by the formation of a solid electrolyte interphase (SEI) layer on the anode surface during the initial battery charge process, some lithium from the cathode is consumed, which reduces appreciably the specific energy and energy density of current LIBs (5%–20% of a total battery capacity).[5–8] In the past few years, increased efforts have been devoted to exploring prelithiation strategies to compensate for the initial lithium loss of LIBs. Although electrochemical prelithiation approaches have been successfully demonstrated, an electrochemical cell needs to be built and disassembled, resulting in complex operations.[9,10] Sacrificial lithium salts can be used as prelithiation additives to electrolytes or electrodes. However, as Armand and co-workers mentioned, undesired gas, such as N2, CO, or CO2, was produced upon the release of lithium.[11] Specifically designed prelithiation additives to electrodes can deliver high “donor” lithium-ion specific capacities to offset the initial lithium loss and hence improve the electrochemical performance of current LIBs. To date, anode prelithiation materials, including stabilized lithium metal powders[12–15] and lithium silicide particles,[16,17] have been investigated due to their high specific capacities. However, their high chemical reactivity and instability under ambient and battery fabrication conditions remain as challenges. Cathode prelithiation materials have relatively high open circuit voltage (OCV) and chemical stability, offering another attractive route. However, little success has been shown on the cathode prelithiation materials, probably
MoreTranslated text
Key words
lithium sulfide/metal,sulfide/metal nanocomposite,high-capacity
AI Read Science
Must-Reading Tree
Example
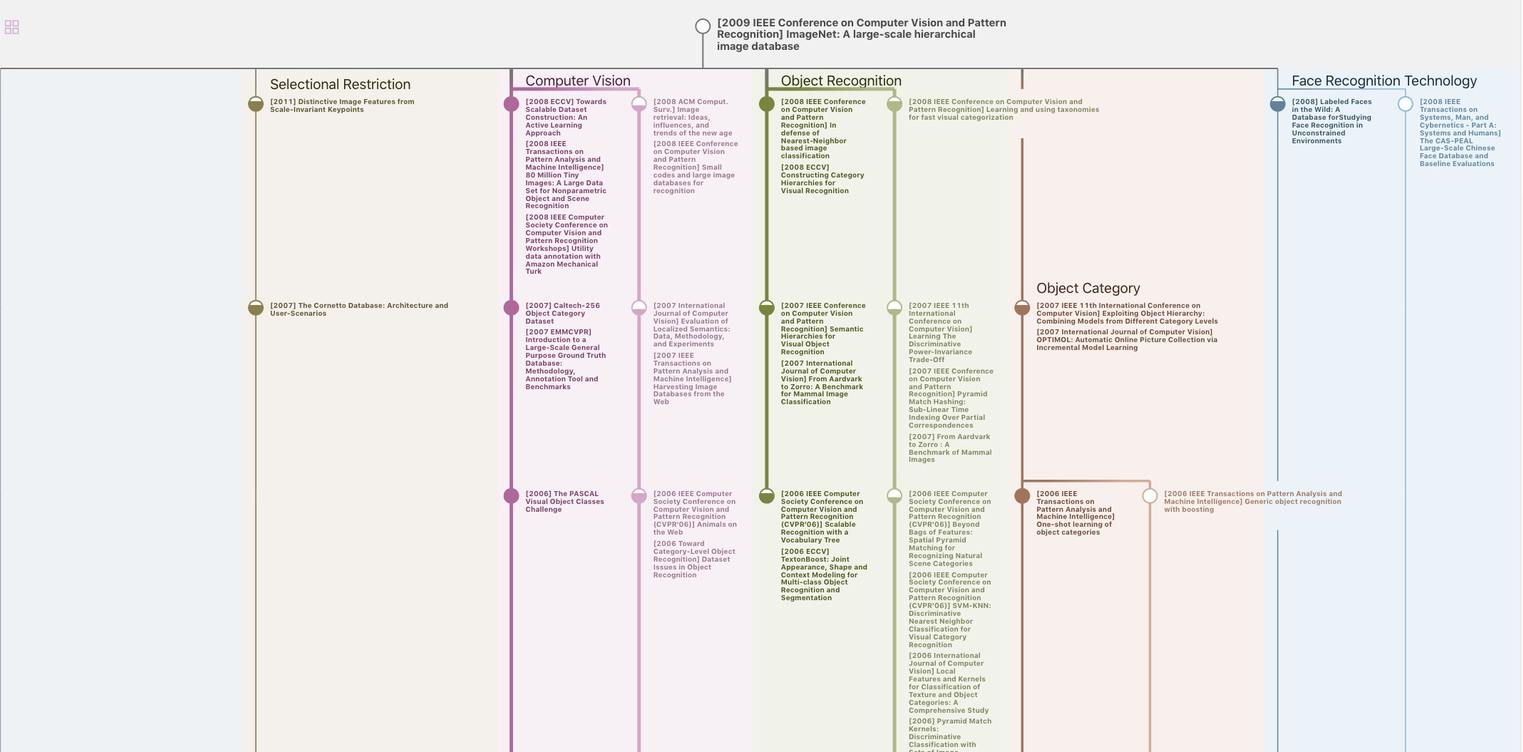
Generate MRT to find the research sequence of this paper
Chat Paper
Summary is being generated by the instructions you defined