Clouds in the atmosphere of the super-Earth exoplanet GJ[thinsp]1214b
Nature(2014)
摘要
We observed 15 transits of the planet GJ 1214b with the Wide Field Camera 3 (WFC3) instrument on the Hubble Space Telescope (HST) between 27 September 2012 and 22 August 2013 Universal Time (ut). Each transit observation (visit) consisted of four orbits of the telescope, with 45-min gaps in phase coverage between target visibility periods due to Earth occultation. We obtained time-series spectroscopy from 1.1 µm to 1.7 µm during each observation. The data were taken in spatial scan mode, which slews the telescope during the exposure and moves the spectrum perpendicularly to the dispersion direction on the detector. This mode reduces the instrumental overhead time by a factor of five compared to staring mode observations. We achieved an integration efficiency of 60%–70%. We extracted the spectra and divided each exposure into five-pixel-wide bins, obtaining spectrophotometric time series in 22 channels (resolution R ≡ λ/Δλ ≈ 70). The typical signal-to-noise ratio per 88.4-s exposure per channel was 1,400. We also created a ‘white’ light curve summed over the entire wavelength range. Our analysis incorporates data from 12 of the 15 transits observed, because one observation was compromised by a telescope guiding error and two showed evidence of a starspot crossing. The raw transit light curves for GJ 1214b exhibit ramp-like systematics comparable to those seen in previous WFC3 data10, 18, 19. The ramp in the first orbit of each visit consistently has the largest amplitude and a different shape from ramps in the subsequent orbits. Following standard procedure for HST transit light curves, we did not include data from the first orbit in our analysis, leaving 654 exposures. We corrected for systematics in the remaining three orbits using two techniques that have been successfully applied in prior analyses10, 18, 20. The first approach models the systematics as an analytic function of time. The function includes an exponential ramp term fitted to each orbit, a linear trend with time for each visit, and a normalization factor. The second approach assumes that the morphology of the systematics is independent of wavelength, and models each channel with a scalar multiple of the time series of systematics from the white-light curve fit. We obtained consistent results from both methods (see Extended Data Table 1), and report here results from the second. See the Supplementary Information and Extended Data Figs 1, 2, 3, 4, 5, 6 for more detail on the observations, data reduction and systematics correction. We fitted the light curves in each spectroscopic channel with a transit model21 to measure the transit depth as a function of wavelength; this constitutes the transmission spectrum. See Fig. 1 for the fitted transit light curves. We used the second systematics correction technique described above and fitted a unique planet-to-star radius ratio Rp/Rs and normalization C to each channel and each visit, and a unique linear limb-darkening parameter u to each channel. We assumed a circular orbit22 and fixed the inclination i to be 89.1°, the ratio of the semi-major axis to the stellar radius a/Rs to be 15.23, the orbital period P to be 1.58040464894 days, and the time of central transit Tc to be 2454966.52488 BJDTDB. These are the best-fit values to the white-light curve. The measured transit depths in each channel are consistent over all transit epochs (see Extended Data Fig. 5), and we report the weighted average depth per channel. The resulting transmission spectrum is shown in Fig. 2. Our results are not significantly affected by stellar activity, as we discuss further in the Supplementary Information. Careful treatment of the limb darkening is critical to the results, but our limb-darkening measurements are not degenerate with the transit depth (see Extended Data Fig. 4) and agree with the predictions from theoretical models (see Extended Data Fig. 6). Our conclusions are unchanged if we fix the limb darkening on theoretical values. We find that a linear limb-darkening law is sufficient to model the data. For further description of the limb-darkening treatment, see the Supplementary Information. The transmission spectrum we report here has the precision necessary to detect the spectral features of a high-mean-molecular-mass atmosphere for the first time. However, the observed spectrum is featureless. The data are best-fitted with a flat line, which has a reduced χ2 of 1.0. We compare several models to the data that represent limiting-case scenarios in the range of expected atmospheric compositions17, 23. Depending on the formation history and evolution of the planet, a high-mean-molecular-mass atmosphere could be dominated by water (H2O), methane (CH4), carbon monoxide (CO), carbon dioxide (CO2) or nitrogen (N2). Water is expected to be the dominant absorber in the wavelength range of our observations, so a wide range of high-mean-molecular-mass atmospheres with trace amounts of water can be approximated by a pure H2O model. The data show no evidence for water absorption. A cloud-free pure H2O composition is ruled out at 16.1σ confidence. In the case of a dry atmosphere, features from other absorbers such as CH4, CO or CO2 could be visible in the transmission spectrum. Cloud-free atmospheres composed of these absorbers are also excluded by the data, at 31.1σ, 7.5σ and 5.5σ confidence, respectively. Nitrogen has no spectral features in the observed wavelength range, but our measurements are sensitive to a nitrogen-rich atmosphere with trace amounts of spectrally active molecules. For example, we can rule out a 99.9% N2, 0.1% H2O atmosphere at 5.6σ confidence. Of the scenarios considered here, a 100% CO2 atmosphere is the most challenging to detect because CO2 has the highest molecular mass and a relatively small opacity in the observed wavelength range. Given that the data are precise enough to rule out even a CO2 composition at high confidence, the most likely explanation for the absence of spectral features is a grey opacity source, suggesting that clouds are present in the atmosphere. Clouds can block transmission of stellar flux through the atmosphere, which truncates spectral features arising from below the cloud altitude24. To illustrate the properties of potential clouds, we perform a Bayesian analysis on the transmission spectrum with a code designed for spectral retrieval of super-Earth atmospheric compositions25. We assume a two-component model atmosphere of water and a solar mix of hydrogen and helium gas, motivated by the fact that water is the most abundant icy volatile for solar abundance ratios. Clouds are modelled as a grey, optically thick opacity source below a given altitude. See Fig. 3 for the retrieval results. For this model, the data constrain the cloud-top pressure to less than 10−2 mbar for a mixing ratio with mean molecular mass equal to solar and less than 10−1 mbar for a water-dominated composition (both at 3σ confidence). At the temperatures and pressures expected in the atmosphere of GJ 1214b, equilibrium condensates of ZnS and KCl could form in the observable part of the atmosphere. Although these species could provide the necessary opacity, they are predicted to form at much higher pressures (deeper than 10 mbar for a 50 × solar metallicity model)16, requiring that clouds be lofted high from their base altitude to explain the spectrum we measured. Alternatively, photochemistry could produce a layer of hydrocarbons in the upper atmosphere, analogous to the haze on Saturn’s moon Titan14, 16. The result presented here demonstrates the capability of current facilities to measure very precise spectra of exoplanets by combining many transit observations. This observational strategy has the potential to yield the atmospheric characterization of an Earth-size planet orbiting in the habitable zone of a small, nearby star. Transmission spectrum features probing five scale heights of a nitrogen-rich atmosphere on such a planet would have an amplitude of 30 parts per million (p.p.m.), which is comparable to the photon-limited measurement precision we obtained with the Hubble Space Telescope. However, our findings for the super-Earth archetype GJ 1214b, as well as emerging results for other exoplanets18, 26, 27, suggest that clouds may exist across a wide range of planetary atmosphere compositions, temperatures and pressures. Clouds do not generally have constant opacity at all wavelengths, so further progress in this area can be made by obtaining high-precision data with broad spectral coverage. Another way forward is to focus on measuring exoplanet emission and reflection spectra during secondary eclipse, because the optical depth of clouds viewed at near-normal incidence is lower than that for the slant geometry observed during transit24. Fortunately, the next generation of large ground-based telescopes and the James Webb Space Telescope will have the capability to make such measurements, bringing us within reach of characterizing potentially habitable worlds beyond our Solar System. Online Content Any additional Methods, Extended Data display items and Source Data are available in the online version of the paper; references unique to these sections appear only in the online paper. Download references This work is based on observations made with the NASA/ESA Hubble Space Telescope that were obtained at the Space Telescope Science Institute, which is operated by the Association of Universities for Research in Astronomy, Inc., under NASA contract number NAS 5-26555. These observations are associated with program GO-13021. Support for this work was provided by NASA through a grant from the Space Telescope Science Institute, the National Science Foundation through a Graduate Research Fellowship (to L.K.), the Alfred P. Sloan Foundation through a Sloan Research Fellowship (to J.L.B.), NASA through a Sagan Fellowship (to J.-M.D.), and the European Research Council (for D.H. under the European Community's Seventh Framework Programme, FP7/2007-2013 Grant Agreement number 247060). The data used in this work can be accessed at the NASA Mikulski Archive for Space Telescopes (http://archive.stsci.edu).
更多查看译文
关键词
Exoplanets
AI 理解论文
溯源树
样例
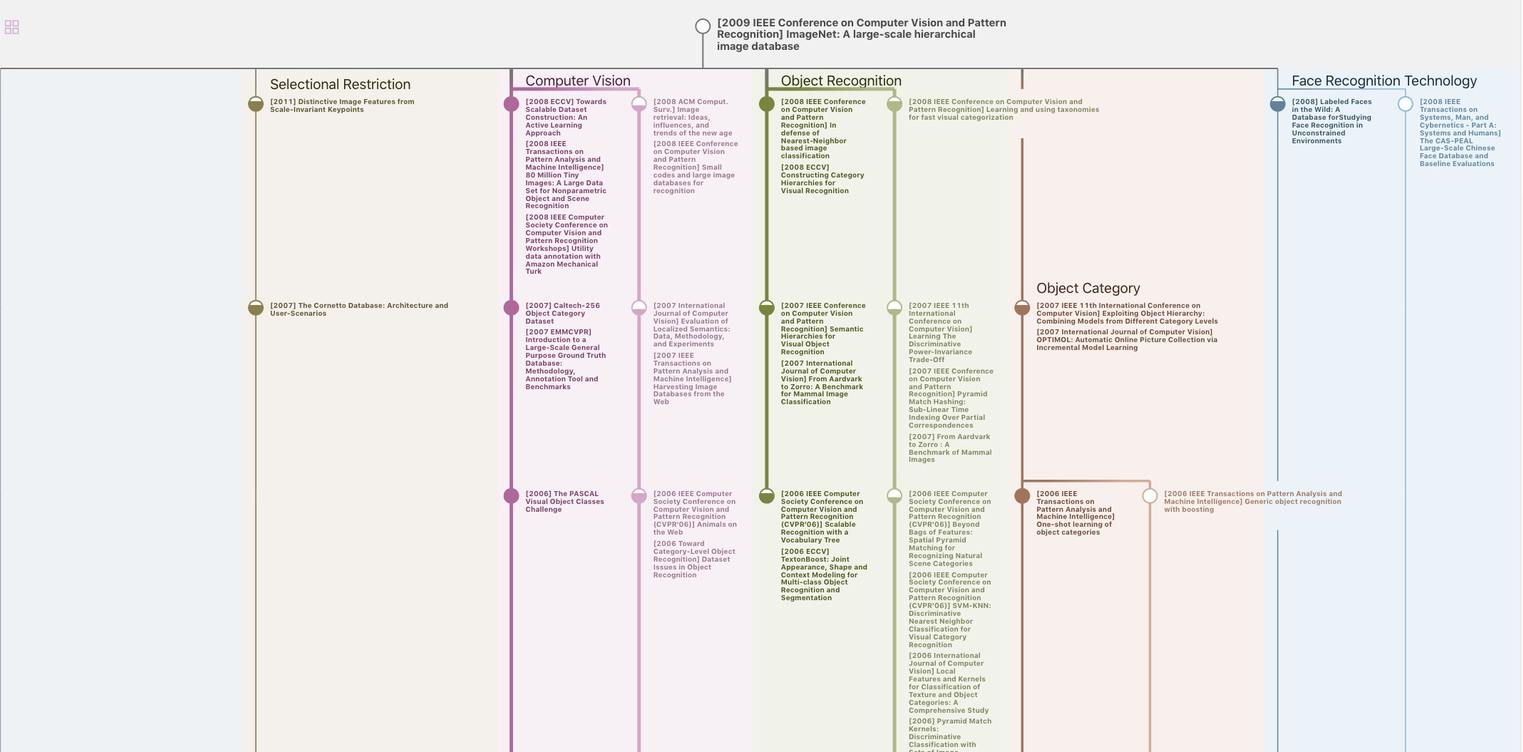
生成溯源树,研究论文发展脉络
Chat Paper
正在生成论文摘要