Identification of a valuable kinetic process in copper-catalyzed asymmetric allylic alkylation.
ANGEWANDTE CHEMIE-INTERNATIONAL EDITION(2011)
Abstract
Neuer Blick auf Bekanntes: Ein bereits beschriebener Prozess zur dynamischen kinetischen asymmetrischen Umsetzung ermöglichte bei acyclischen Substraten die Identifizierung eines wichtigen kinetischen Prozesses in der Titelreaktion (siehe Schema; CuTC=Kupfer(I)-thiophencarboxylat, Naphth=Naphthyl). Beschrieben werden die Optimierung der Reaktionsbedingungen, die Allgemeingültigkeit der Methode und mechanistische Überlegungen. Since the past decade, copper-catalyzed asymmetric allylic alkylation has attracted the interest of many research groups, and such groups have succeeded in considerably extending the range of applications as well as the efficiency of this reaction.1 Prochiral substrates are generally used, thus providing versatile chiral synthons stemming from a selective SN2′ displacement of the leaving group.2 Because of this particular feature, chemists rarely studied racemic substrates as an anti SN2′ process would lead to racemic products. However, the few investigations on the reactivity of this class of substrates gave rise to the development of outstanding processes that significantly contributed to the understanding of the reaction.3 In 1998, Pineschi, Feringa, and co-workers achieved pioneering work in this field with the kinetic resolution of cycloalkadiene monoepoxide in the presence of chiral phosphoramidite ligands.4 A few years later, the same groups established that the enantiomers of the racemic allylic epoxide might have different reactivity under the reaction conditions and that they could be selectively transformed into two distinct regioisomers (regiodivergent kinetic resolution).5 Recently, our group reported on the development of a dynamic kinetic asymmetric transformation (DYKAT).6 Such a process used the difference in reactivity of the enantiomers of a racemic substrate to quantitatively form a unique enantioenriched product (up to 99 % ee; Scheme 1 a). From a synthetic point of view, this reaction constitutes a significant achievement considering the complete conversion of the starting material as well as the formation of a unique isomer of the alkylation product. Notwithstanding, the reaction proved to be effective only on 3-bromocyclohexene derivatives and this lack of generality limited its application in organic synthesis. To address this problem, the reactivity of acyclic racemic substrates should be studied (Scheme 1 b). Herein, we present our findings on the difficulty of performing a DYKAT process on acyclic substrates and how the conformational flexibility of these substrates led us to unveil a new and valuable process for copper-catalyzed asymmetric allylic alkylation. Evaluation of acyclic allylic halides as candidates for a dynamic kinetic asymmetric transformation. Our investigations started with the reaction of (E)-4-bromopent-2-ene (1)7 with phenethylmagnesium bromide in dichloromethane at −78 °C. In using the reaction conditions previously described for the DYKAT,6a,6b a combination of CuTC (copper (I) thiophenecarboxylate) and the phosphoramidite ligand L1 was chosen as the catalyst. This attempt quantitatively afforded the alkylation product 3 albeit as an inseparable mixture of E and Z stereoisomers (Table 1, entry 1). Interestingly, we noticed that both isomers of 3 were isolated in promising enantiomeric excesses of 72 and 66 %, respectively. The use of ligands L2 and L3 failed to improve this result even though a slight preference for the Z isomer was observed (entries 2 and 3). Better enantioselectivities and an E/Z ratio of 45:55 were obtained when the sterically hindered ligand L4 was used (entry 4). Performing the reaction with (E)-4-chloropent-2-ene (2) led to a significant increase of the ee values to 84 and 81 % (entry 5).8 Furthermore, improvement of the enantioselectivity was achieved when the Grignard reagent was added over a period of 30 minutes (entry 6).9 Thus, the alkylation product 3 was obtained as an equimolar mixture of the E and Z isomers in 90 and 88 % ee, respectively. Entry Substrate Ligand Conv. [%][b] E/Z[c] ee [%][d] (E)-3 (Z)-3 1 1 L1 >99 44:56 72 66 2 1 L2 >99 37:63 72 54 3 1 L3 >99 35:65 66 42 4 1 L4 >99 45:55 76 74 5 2 L4 >99 47:53 84 81 6[e] 2 L4 >99 48:52 90 88 Goering and co-workers have demonstrated that a significant amount of the Z adduct could be formed in the alkylation of various E-allylic substrates by using an organocopper reagent, but never as an equimolar mixture with the E adduct.10 This specific feature strongly compromised the development of a DYKAT, wherein the alkylation product should be recovered as a single isomer.6c,6d However, the high level of enantioinduction observed for each isomer prompted us to get more insight into the reaction mechanism. Identification of the process. Plausible reaction mechanism. Some prerequisites are needed to better understand the reaction pathway: 1) the reactivity of each enantiomer of the allylic chloride 2 has to be distinguished, 2) as mentioned, the reactivity of the organocopper species implies an anti-SN2′ displacement of the leaving group,2 and 3) the chiral catalyst induces a selective attack on one enantiotopic face of the disubstituted olefin, that is, the Si face given the observed absolute configuration of the alkylation products. The facial selectivity might be ascribed to steric interactions between the chiral ligand and the vinylic methyl group but the difficulty in obtaining a crystal structure of the catalyst prevented us from asserting exactly which interactions governed the facial selectivity. Thus, (S)-2 is perfectly disposed to undergo an oxidative addition from its pro-E conformer, leading to the (E)-σ-allyl intermediate Aσ. Subsequent reductive elimination, either directly or through the π-allyl complex Aπ, will selectively afford the E isomer of the alkylation product 5. In sharp contrast, an anti attack on the pro-E conformer of (R)-2 would involve the approach of the chiral catalyst on the Re face of the olefin. This possibility would be considerably disfavored but the conformational flexibility offered by an acyclic system allowed a rotation around the C3–C4 bond, leading to the pro-Z conformer of the substrate. The latter could be attacked on the Si enantiotopic face providing (Z)-σ-allyl intermediate Bσ, then a possible π-allyl complex Bπ, and finally (Z)-5 after reductive elimination. Interestingly, when the reaction was stopped at 72 % conversion, a 43:57 mixture of the E/Z isomers of 3 was recovered, indicating a slight kinetic preference for the formation of the Z isomer (Scheme 4). This observation means that the rotation required for the formation of this stereoisomer did not slow down the reactivity of (R)-2 and that the system could easily make up for this expense of energy if the approach of the chiral catalyst is facilitated. This mechanism represents the first example of stereodivergent kinetic resolution (SKR) in copper-catalyzed asymmetric allylic alkylation.15 Control experiment. Having established a plausible reaction pathway, a specific aspect remains intriguing: the formation of a π-allyl intermediate is neither stereo- nor enantiodetermining in the proposed mechanism.16 In contrast to cyclic systems, it could be assumed that the flexibility of acyclic substrates may allow preorganization of the system before the key oxidative addition step. Thus, in the present case and in opposition to the DYKAT process, the enantio-, stereo- and regioselections are determined before the possible formation of a π-allyl moiety, thereby rendering its involvement only formal. This particular feature might be exemplified by the application of the process to unsymmetrical substrates. Unfortunately, the preparation of the regioisomerically pure unsymmetrical secondary allylic chlorides remains a difficult challenge in organic synthesis.17 (E)-(3-chlorobut-1-en-1-yl)benzene (6) was a rare exception and has been synthesized as a unique isomer.18 To our delight, the alkylation reaction gave the SN2′ adduct 7 as the major product (7/8 92:8) and as a mixture of E and Z stereoisomers in 84 and 92 % ee, respectively (Scheme 5 a). Importantly, a different chiral ligand, L5, was needed owing to the significant structural modification of the substrate.19 Interestingly, when substrate 9 was submitted to the reaction conditions, (E)-10 was obtained as the sole SN2′ stereoisomer albeit in a racemic form (Scheme 5 b). This result could be explained by a strong 1,3-allylic strain destabilizing the pro-Z conformer of 9 (Me–Me interaction, ΔE9=5.10 kcal mol−1) and leading exclusively to the formation of the racemic E-adduct 10.20 The larger proportion of the SN2 adduct 11 formed in this reaction could be ascribed to the propensity of the system to release the strain induced by the formation of a quaternary center. Use of unsymmetrical substrates. n.d.=not determined The generality of the reaction was finally explored using the readily accessible model substrate 2 and various Grignard reagents (Table 2). The introduction of homoprenyl group was achieved with rigorously the same efficiency than the model phenethyl group, affording the product 13 in 92 % yield (isolated) and ee values of 90 and 88 % (entries 1 and 2, Table 2). The addition of dodecylmagnesium bromide provided 14 in high yield and in about 90 % ee (entry 3). (R,E)- and (R,Z)-8-(tert-butoxy)-4-methyloct-2-ene (15) were obtained in good yield and as an equimolar mixture. Surprisingly, the E isomer was isolated with lower asymmetric induction than the Z isomer (74 and 88 % ee, respectively; entry 4). Cyclohexylmagnesium bromide proved to react in the same manner as the primary alkyl Grignard reagents albeit in lower enantioselectivity (entry 5). In stark contrast, phenylmagnesium bromide displayed a totally different reactivity, leading to racemic (E)-pent-3-en-2-ylbenzene (17) in good stereoselectivity (entry 6).21 Finally, (E)-7-chloroundec-5-ene (12) showed a similar reactivity and adducts 18 were recovered in 95 % yield and 88 % ee (entry 7).22 Entry Substr. R2 Prod. Yield E/Z[c] ee [%][d] [%][b] eeE eeZ 1 2 PhCH2CH2 3 85 48:52 90 88 2 2 (CH3)2CCHCH2CH2 13 92 48:52 90 88 3 2 C12H25 14 97 44:56 89 91 4 2 tBuO(CH2)4 15 91 49:51 74 88 5 2 Cy 16 87 48:52 80 76 6 2 Ph 17 85 93:7 0 0 7 12 PhCH2CH2 18 95 48:52 88[f] – In addition to mechanistic interest, the process reported herein could be a valuable tool for organic synthesis. Simple transformations such as ozonolysis, hydrogenation, or cross-metathesis might erase the dichotomy between the stereoisomers of the alkylation product and quantitatively afford a single enantioenriched compound. For instance, (E)- and (Z)-3 were prepared on a synthetically useful scale (2 mmol) using the SKR process and subsequently reacted with methyl acrylate in the presence of the second-generation Hoveyda–Grubbs catalyst (Scheme 6).23 (E)-α,β-unsaturated ester (19) was isolated as the sole stereoisomer in 82 % yield and 88 % ee.24 Scale-up preparation and derivatization of adducts 3. HG II=Hoveyda–Grubbs second-generation catalyst. In conclusion, acyclic allylic systems have shown a fundamentally different reactivity profile compared to cyclic substrates, which precluded the development of a DYKAT. However, the conformational flexibility of this class of substrates allowed us to identify a highly enantioselective stereodivergent kinetic resolution (up to 91 % ee). Such a process is rare in transition-metal catalysis and unprecedented in copper chemistry. Studies are currently ongoing in our laboratory to extend our comprehension of the system and particularly to understand the striking difference between DYKAT and SKR processes towards the influence of a π-allyl intermediate on the reaction outcome. In a flame-dried Schlenk tube an under nitrogen atmosphere, CuTC (4.8 mg, 0.025 mmol, 0.1 equiv) and L4 (17.6 mg, 0.028 mmol, 0.11 equiv) were dissolved in dry CH2Cl2 (2 mL) and the solution was stirred for 10 min at room temperature. The reaction mixture was then cooled to −78 °C and the freshly prepared substrate (0.25 mmol) was added. After 10 min at this temperature, the Grignard reagent (1 M in Et2O, 0.3 mL, 0.3 mmol, 1.2 equiv) was added over 30 min and the reaction mixture was stirred for 1 h. The reaction was quenched with HCl 1 M (5 mL) and extracted with diethyl ether (3×5 mL). The organic layer was dried over Na2SO4, filtered, and concentrated under vacuum. The crude reaction mixture was purified by chromatography on silica gel (n-pentane) and the desired product (up to 97 %) was recovered as a colorless liquid. For additional details, see the Supporting Information. Detailed facts of importance to specialist readers are published as ”Supporting Information”. Such documents are peer-reviewed, but not copy-edited or typeset. They are made available as submitted by the authors. Please note: The publisher is not responsible for the content or functionality of any supporting information supplied by the authors. Any queries (other than missing content) should be directed to the corresponding author for the article.
MoreTranslated text
Key words
alkylation,allylic compounds,asymmetric catalysis,copper,Grignard reagents
AI Read Science
Must-Reading Tree
Example
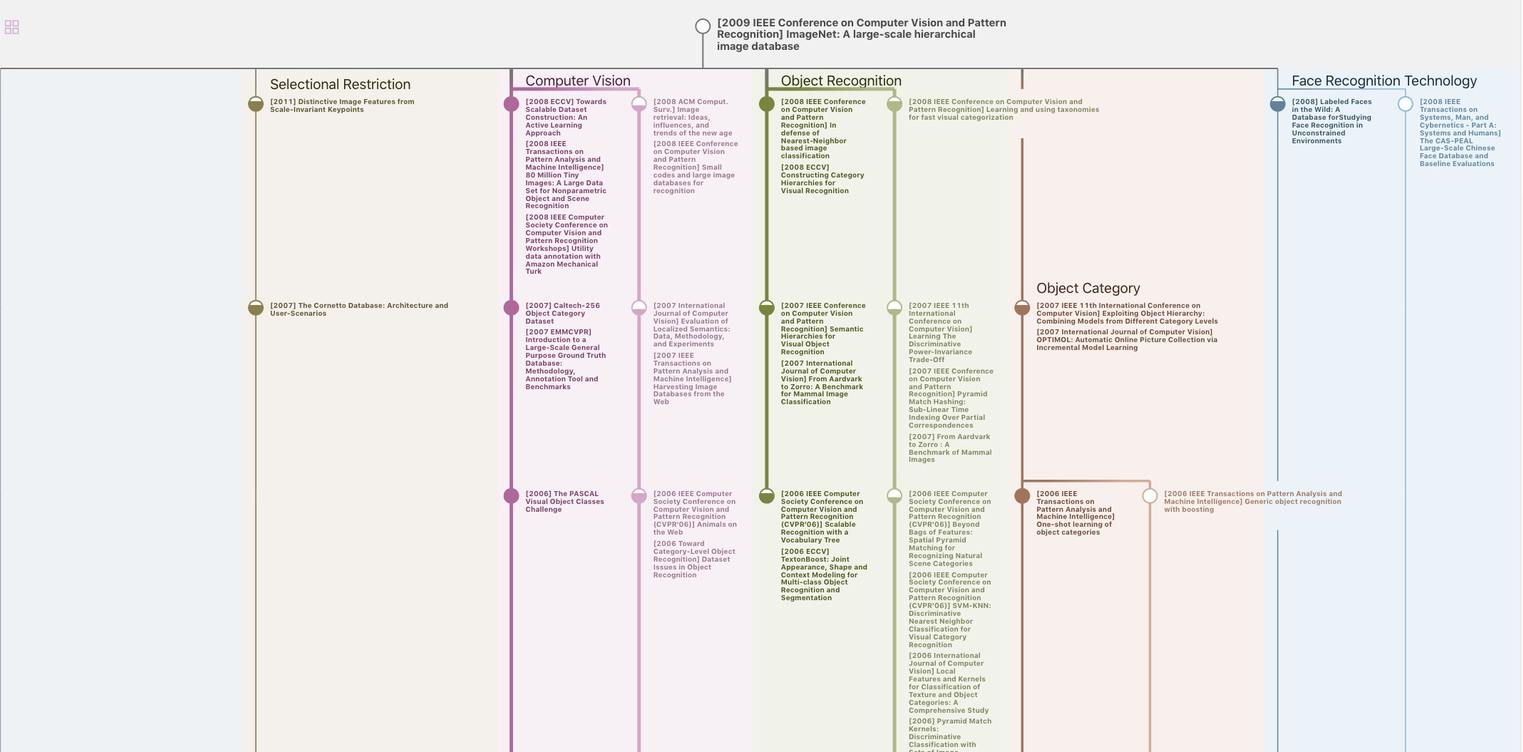
Generate MRT to find the research sequence of this paper
Chat Paper
Summary is being generated by the instructions you defined