Ventilator management in the surgical intensive care unit.
TEXAS HEART INSTITUTE JOURNAL(2010)
Abstract
Invasive mechanical ventilation at its most basic level provides support for intubated patients during critical illness. Along with this goes the ability to affect pulmonary gas exchange, to relieve respiratory distress, and to improve lung expansion. Adult Respiratory Distress Syndrome (ARDS) was first described in the late 1960s as a constellation of respiratory failure, cyanosis refractory to supplemental oxygen, decreased lung compliance, noncardiogenic pulmonary edema, and bilateral pulmonary infiltrates.1 Each year, approximately 150,000 new cases of ARDS arise in both medical and surgical patients, and, in some series, the mortality rate continues to be as high as 30%.2 Since its initial description by Ashbaugh and colleagues,1 ARDS is now recognized not just as an isolated pulmonary process, but as the result of a systemic inflammatory response to sepsis that leads to the development of pulmonary edema. The American-European Consensus Conference on ARDS met in 1994 and defined ARDS as a constellation involving the following hallmarks3: Acute onset of symptoms; Ratio of arterial oxygen to fraction of inspired oxygen (PaO2/FIO2) <200 mmHg; Bilateral infiltrates on frontal chest radiograph; and Pulmonary artery wedge pressure ≤18 mmHg (or no clinical evidence of left atrial hypertension). It has further been recognized that the disease process can be broken down into multiple components that ultimately end in tissue damage at the alveolar level. As a result of inflammatory mediators, leukocytes adhere to the basement membrane, move across it, and then degranulate, triggering microvascular thrombosis and ultimately increasing pulmonary vascular resistance, increasing shunt, decreasing compliance, and worsening V/Q mismatch. In the late 1990s, the ARDSnet study4 showed an 8.8% absolute mortality decrease when a lower (6 mL/kg body weight) tidal volume and plateau pressure (Pplat ≤30 cmH2O) was used for mechanical ventilation—compared with a traditional (12 mL/kg body weight) tidal volume and plateau pressure (Pplat ≤50 cmH2O). In addition, study investigators found deceased ventilator length of stay, lower interleukin-6 (IL-6) levels in the blood, and less multisystem organ failure.4 Current goals seen in the literature focus on limiting lung damage (by preventing overdistention of stiff lung), limiting cyclical collapse, reopening alveolar units, and maximizing oxygen delivery. Two modes of mechanical ventilation are very useful for these goals: bilevel ventilation and airway pressure release ventilation (APRV). These fall under the “open lung” concept of ventilation, which focuses on the following5: Pressure control to limit airway pressures and prevent overdistention, as well as to prevent the cyclical opening and closing of alveolar units; Manipulation of the inspiratory:expiratory ratio with use of inverse-ratio ventilation, which enables higher mean airway pressure and the recruitment of collapsed alveoli; and The ability of the patient to breathe spontaneously, which results in increased patient comfort and synchrony with the ventilator. Bilevel ventilation sets a range for positive end-expiratory pressure (from PEEPHigh to PEEPLow). Inspiratory and expiratory times can be manipulated as well, enabling inverse-ratio ventilation in which short expiratory times enable ventilation and longer inspiratory times encourage recruitment of alveoli, thus facilitating oxygenation. Bilevel ventilation and APRV are essentially 2 levels of continuous positive airway pressure that allow a mixture of spontaneous and ventilator-mandated breaths. These 2 pressure levels are the PEEPHigh and PEEPLow settings. The timing of the cycle is referred to as time high (TH) and time low (TL). The difference between PEEPHigh and PEEPLow serves as the driving force for ventilation and can be adjusted to deliver a tidal volume of 6 to 8 cc/kg in accordance with the ARDSnet guidelines (Fig. 1). As alveoli are recruited and the lungs become more compliant, this number might need to be adjusted so that excessive tidal volumes are avoided. A tidal volume that maintains a pH of greater than 7.25 is sufficient in most patients. The PEEPLow setting is determined, ideally, by identifying the inflection point on a pressure–volume curve, so that alveolar collapse is prevented (Fig. 2). Fig. 2 The upper (gray arrow) and lower (red arrow) inflection points on a pressure–volume curve are shown. Above the upper inflection point, where the curve flattens out, there is a risk of alveolar overdistention. At pressures less than the ... Fig. 1 The PEEPHigh and PEEPLow settings are shown. The difference between the 2 settings creates the driving pressure for ventilation. Inspiration occurs during PEEPHigh time, and expiration occurs when the lungs deflate during PEEPLow time. Throughout ... Beyond lung-protective ventilator measures, there are techniques that involve positioning the patient in a manner that reduces V/Q mismatch. These are rotational (or kinetic) therapy and prone positioning. Rotational therapy involves turning the patient at least 42° to each side for variable periods of time, which can help to open atelectatic lung segments. Some of the beneficial effects of rotational or prone positioning may be consequent to relieving compression from the heart. Albert and Hubmayr6 found that up to 40% of the left lung is under the heart when the patient was supine, versus less than 1% when prone. Relieving the compression of the viscera on the lungs may decrease the inspiratory pressure needed to open collapsed alveoli, decrease the end-expiratory pressure needed to keep alveoli open, and reduce the cyclical opening and closing of alveoli.
MoreTranslated text
Key words
respiration
AI Read Science
Must-Reading Tree
Example
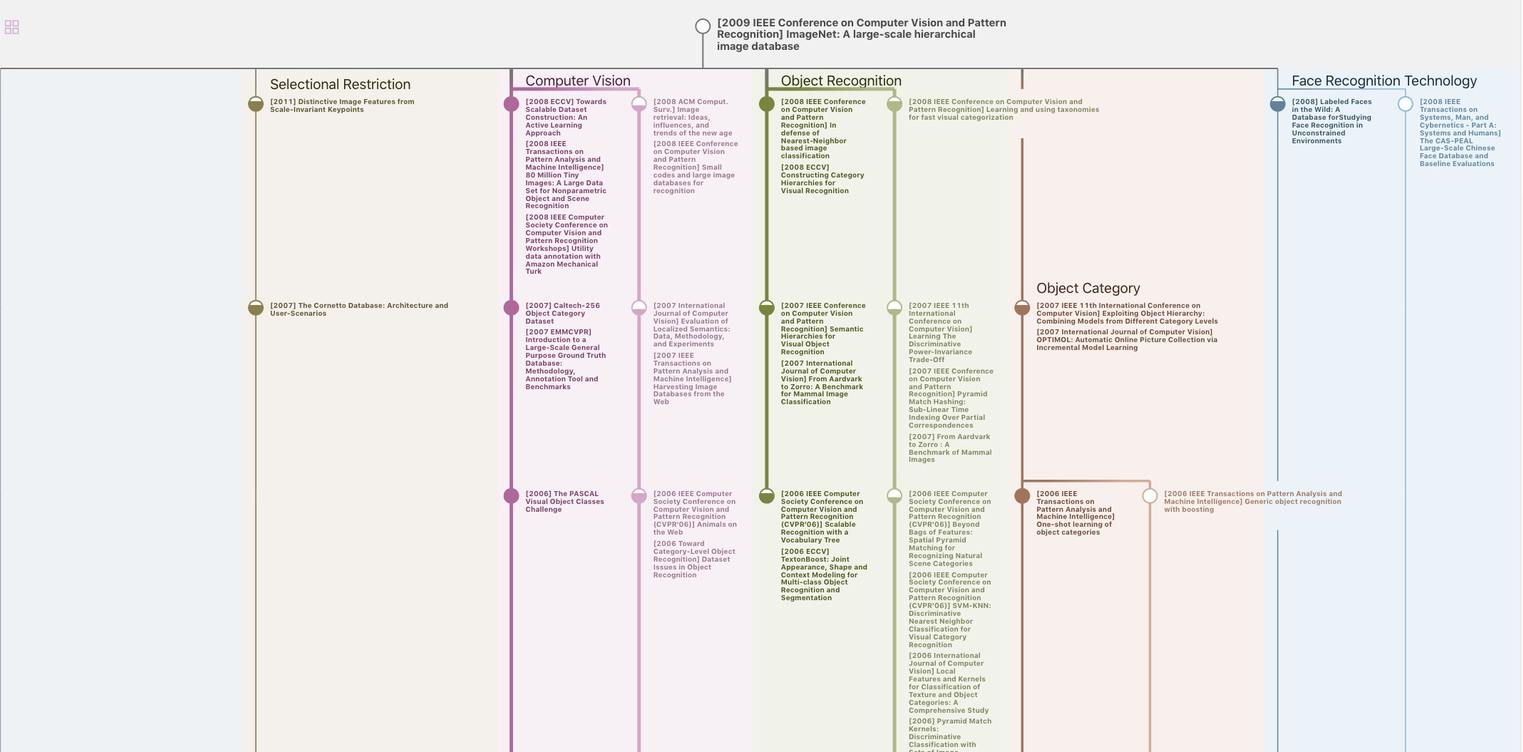
Generate MRT to find the research sequence of this paper
Chat Paper
Summary is being generated by the instructions you defined