Field studies demonstrate bivoltinism in the mountain pine beetle.
AMERICAN NATURALIST(2014)
摘要
Previous articleNext article FreeReplyField Studies Demonstrate Bivoltinism in the Mountain Pine Beetle (A Reply to Bentz and Powell)Jeffry B. Mitton and Scott FerrenbergJeffry B. MittonDepartment of Ecology and Evolutionary Biology, University of Colorado, Boulder, Colorado 80309*Corresponding author; e-mail: [email protected].†The authors contributed equally to this reply. Search for more articles by this author and Scott FerrenbergDepartment of Ecology and Evolutionary Biology, University of Colorado, Boulder, Colorado 80309†The authors contributed equally to this reply. Search for more articles by this author PDFPDF PLUSFull Text Add to favoritesDownload CitationTrack CitationsPermissionsReprints Share onFacebookTwitterLinkedInRedditEmailPrint SectionsMoreDryad data: http://dx.doi.org/10.5061/dryad.4df03.We appreciate Bentz and Powell’s (2014; hereafter interchangeable with “the authors”) interest in our article (Mitton and Ferrenberg 2012). We are pleased the authors have confirmed the summer generation of mountain pine beetles (MPBs; Dendroctonus ponderosae), which we reported is now a widespread phenomenon arising in response to greater than average annual temperatures. A recent article by Bentz et al. (2014) also reported a switch from one to two generations of MPBs in roughly one year at a California site, indicating that bivoltinism, or two MPB generations in a year, was not isolated to our Colorado sites. While Bentz et al. (2014) documented two generations within 13 months and state that technically the two generations must occur within 12 months to meet the definition of bivoltine, this switch to multiple generations of MPBs within the same time span historically necessary for one generation has large demographic implications. This decrease in generation times is possible because, as reported by Bentz et al. (2014), “substantial genetic variation in development time is known to exist among MPB (Dendroctonus ponderosae) populations across the western United States” (p. 434).Despite their confirmation of summer generations of MPBs and the additional verification that a summer generation can produce offspring that emerge and mate during the same season, Bentz and Powell argue against our report of bivoltinism. The authors’ arguments fall into three categories: (1) critiques of our methodology, which the authors consider inadequate for monitoring MPB development—that is, while they support our report of a summer generation, they argue that we did not demonstrate bivoltinism because of flaws in our experimental design; (2) arguments that beetles at our study sites cannot exhibit bivoltinism because this would counter the authors’ assertion that generation times of MPBs are relatively similar across their expansive range; and (3) conflicts between the authors’ computer simulation model of MPB phenology, which suggests that MPBs require at least one year per generation, and our direct field observation of two generations of MPBs in one year.We reject these criticisms because (1) Bentz and Powell misrepresent our study’s methodology and subsequently take issue with their version of our methods, not those we employed; (2) MPB development rates and generation times are documented to be highly variable, even under equivalent thermal regimes, such that beetles living in shared environments and from the same broods can have generation times differing by more than twofold (Reid 1962; Bracewell et al. 2013; Bentz et al. 2014); and (3) given that the thermal energy needed by MPBs to develop from egg to adult within and among broods is highly variable (Bentz et al. 2001, 2014; Carrol et al. 2006; Bracewell et al. 2013), the application of one phenology model to all MPB populations regardless of location or environment is questionable. In fact, the derivation of one phenology model that would be accurate for all beetles within a population, let alone across all populations of MPBs, is highly improbable given the evidence of genetic differentiation among MPB populations (Stock and Amman 1980; Kelley et al. 2000; Bracewell et al. 2011; Gayathri Samarasekera et al. 2012), the importance of local adaptation by MPB populations to thermal regimes across latitude and elevation (Bentz et al. 2001; Bracewell et al. 2013), and the documented effects of local ecological influence, such as host tree species and quality, on beetle growth and development (Sturgeon and Mitton 1986; Langor 1989; Langor et al. 1990; Cerezke 1995). While we will expand in detail below, we assert that the model used by Bentz and Powell—which was parameterized using data on MPB development in petri dishes at constant temperatures in a laboratory—is not likely to capture the full range of variation in phenotypes and responses to the range of conditions found in natural field settings (for model development, see Logan and Amman 1986; Bentz et al. 1991; Gilbert et al. 2004). Thus, using this model to reinterpret our findings and dismiss our firsthand observations is unfounded.Comments on Our Methods and Description of MPB Life HistoryBentz and Powell strongly critique of our methods for assigning MPBs to cohorts and for following MPB development. In particular, the authors argue that flaws in our procedure led us to monitor broods with unknown dates of inception and that by using random trees we lacked sufficient knowledge of MPB attack history, leading to a scenario where we misidentified adult beetles from prior attacks as recently eclosed (emerged from pupation) beetles—or as stated by Bentz and Powell,[Mitton and Ferrenberg] sampled life stages under the bark of random trees … , although the timing of cohort initiation in these trees was not known. They presumed that these trees were attacked the previous fall (i.e., September 2009). … A key piece of missing evidence in the Mitton and Ferrenberg (2012) study is knowledge of when these trees were actually attacked. (p. 790)Given this presumed flaw, the authors argue for the dismissal of our observations of beetle development and instead resort to the application of their phenology simulation model to determine whether beetles at our field sites could produce multiple generations in a year.We reject this criticism and encourage the authors to read paragraphs three and four of “Material and Methods,” where we explain our procedures for selecting trees with no prior MPB attacks and, hence, no existing broods under the bark. Using trees with no preestablished broods, we then used pheromone lures to attract MPBs to predetermined target trees and subsequently examined each tree for attacks at a regular time interval to establish reasonable estimates of attack dates and locations within a tree.Trees attacked in June 2009 released adult beetles in August 2009, and we noted that previously unattacked trees came under attack at the same time. In June 2010, exit holes appeared on trees first attacked the previous summer in August. We peeled the bark and found that the brood inside was in various stages of development, including larvae, empty pupal chambers, teneral adults, and numerous fully pigmented adults boring exit holes (fig. 1). These observations revealed the existence of a summer generation and also indicated that some beetles completed development between August 2009 and June 2010, demonstrating the existence of a winter generation.Figure 1. Characteristic mountain pine beetle (Dendroctonus ponderosae) gallery established in the month of August, releasing mature beetles the following June. After approximately 360 growing degree days, the brood consisted of larvae, teneral adults, and mature adults chewing exit holes. Empty pupal chambers and exit holes indicate that some beetles have already emerged.View Large ImageDownload PowerPointBecause Bentz and Powell support our report of a summer generation at our Colorado field sites in 2009 but subsequently argue the impossibility of a winter generation, we calculated the number of growing degree days (GDDs) available for development of the summer MPB generation in 2009 and compared that with the number of GDDs available for the winter MPB generation, which released adults in June of 2010. We calculated GDDs by subtracting the previously reported MPB developmental threshold of 5.5°C from daily mean temperatures (long-term data are available from the Niwot Ridge Long-Term Ecological Research C-1 climate station, http://niwot.colorado.edu; data and calculations analyzed here are available in the Dryad Digital Repository: http://dx.doi.org/10.5061/dryad.4df03 [Mitton and Ferrenberg 2014]) and then transforming negative values to 0 before summation as in Mitton and Ferrenberg (2012). From June 8 (the date we reported seeing the first MPB attacks) to August 15, 2009 (the date we first noted the emergence of a summer generation), the summer generation had 391 GDDs to complete development. The following winter generation, which we consider as beetles developing from August 16, 2009, to June 27, 2010 (the date we noted the first MPB attacks on trees in 2010), had 379 GDDs. Thus, the number of GDDs available for the summer and winter generations differed by only 12, which is equivalent to one calendar day with a mean temperature of 17.5°C.We also reject Bentz and Powell’s use of data recorded >550 km away from our study site for characterizing the historical MPB life history of our study’s location (Bentz et al. 2014). As reported in our original note, a report from a US Forest Service experimental forest site at a similar elevation and only 30 km west of our study site verifies that MPBs attacking a shared tree species had univoltine generations in years prior to our study (Tishmack et al. 2005). Thus, we reiterate that broods observed for our study had a mixture of univoltine (which we consider historically common) and bivoltine (which we observed arising in response to warming) generations.Documented Variation in MPB Responses to TemperatureA decrease in MPB generation times in response to warming is possible because of genetic variation for development rates within and among populations (Reid 1962; Reid and Gates 1970; Bentz et al. 2001, 2014; Bracewell et al. 2013). In fact, Bentz and Powell note in their comment that sufficient variation exists in the development rates of MPB populations to allow some high-elevation populations to exhibit a mix of univoltinism (one generation per year) and semivoltinism (one generation every two years) within the same thermal regime. Furthermore, median generation times across broods monitored by Bentz et al. (2014) varied by more than sixfold (mean = 373 days; range = 585 days) while nearly 39% of broods exhibited a mixture of voltinism strategies, indicating that even sibling beetles in a shared environment can vastly differ in the time and associated thermal energy necessary for completing development. Importantly, patterns of development time exhibit adaptive variation; the thermal energy required for one generation is less at sites at higher elevation and latitude (cooler climate) than at sites at lower elevation and latitude (warmer climate; Bracewell et al. 2013; Bentz et al. 2014).It is paradoxical that Bentz and Powell assert that a minimum of 883 degree days (GDDs >5.5°C) is needed to complete development and then use this assertion to reject our report of bivoltinism in an MPB population. The authors (and colleagues) have previously reported a nearly threefold variation in the number of degree days (GDDs >10.0°C) required for MPB development across studies (Carroll et al. 2006) while also reporting that total degree days (GDDs >15°C) necessary for emergence across field populations varied by nearly sevenfold (Bentz et al. 2014). This evidence of heritable genetic variation for development and growth rates indicates that the threshold temperature for development or the rates of development and growth (or any combination) are variables subject to selection to adapt populations to their local environments and changing climate. Evidence for climate-driven changes in life history, particularly generation times, have been reported in other MPB populations and other Dendroctonus beetles. For example, MPBs in the Greater Yellowstone Ecosystem are shifting from a semivoltine (one generation every two or more years) to a univoltine life cycle (Logan et al. 2010), while some populations of spruce beetles (Dendroctonus rufipennis) have decreased generation times from two years to one (Berg et al. 2006). The reproductive advantage of shorter generation times would create strong selection favoring phenotypes capable of rapid growth and development.ConclusionsBentz and Powell confirmed our observation of a summer generation of MPBs in 2009 but argued that eggs deposited in August 2009 could not complete development by June 2010. However, broods we observed as being established in August 2009, at the same time the summer generation was emerging, released adults the following summer in June 2010 (see fig. 1 in Mitton and Ferrenberg 2012). The authors’ criticisms of our methods for following beetle development are unfounded, as they ignored our reported procedures and later argued for a future study using methods nearly equivalent to those we employed. Although Bentz and Powell’s simulation model denies the possibility of bivoltinism, we show that the GDDs available for the summer generation of MPBs at our field site (which is accepted by the authors as a valid generation) is nearly equivalent to the GDDs available for the winter generation we observed. Thus, reinterpreting our findings via application of the authors’ phenology model, which is based on MPB development in petri dishes under constant temperatures in a laboratory, is neither necessary nor founded given that several studies confirm the existence of substantial heritable variation in MPB development rates, even for siblings developing in equivalent environments and thermal regimes.AcknowledgmentsWe thank J. Langenhan and two anonymous reviewers for comments on the manuscript. Additional support was provided by a Department of Energy grant (DE-FG02-07ER64457).Literature CitedBentz, B. J., J. A. Logan, and G. D. Amman. 1991. Temperature-dependent development of the mountain pine beetle (Coleoptera: Scolytidae) and simulation of its phenology. Canadian Entomologist 123:1083–1094.First citation in articleCrossrefGoogle ScholarBentz, B. J., J. A. Logan, and J. C. Vandygriff. 2001. Latitudinal variation in Dendroctonus ponderosae (Coleoptera: Scolytidae) development time and adult size. Canadian Entomologist 133:375–387.First citation in articleCrossrefGoogle ScholarBentz, B. J., and J. A. Powell. 2014. Mountain pine beetle seasonal timing and constraints to bivoltinism. American Naturalist 184:787–796.First citation in articleLinkGoogle ScholarBentz, B., J. Vandygriff, C. Jensen, T. Coleman, P. Maloney, S. Smith, A. Grady, and G. Schen-Langenheim. 2014. Mountain pine beetle voltinism and life history characteristics across latitudinal and elevational gradients in the western United States. Forest Science 60:434–449.First citation in articleCrossrefGoogle ScholarBerg, E. E., J. D. Henry, C. L. Fastie, A. D. De Volder, and S. M. Matsuoka. 2006. Spruce beetle outbreaks on the Kenai Peninsula, Alaska, and Kluane National Park and Reserve, Yukon Territory: relationship to summer temperatures and regional differences in disturbance regimes. Forest Ecology and Management 227:219–232.First citation in articleCrossrefGoogle ScholarBracewell, R. R., M. E. Pfrender, K. E. Mock, and B. J. Bentz. 2011. Cryptic postzygotic isolation in an eruptive species of bark beetle (Dendroctonus ponderosae). Evolution 65:961–975.First citation in articleCrossref MedlineGoogle Scholar———. 2013. Contrasting geographic patterns of genetic differentiation in body size and development time with reproductive isolation in Dendroctonus ponderosae (Coleoptera: Curculionidae, Scolytinae). Annals of the Entomological Society of America 106:385–391.First citation in articleCrossrefGoogle ScholarCarroll, A. L., J. Régnière, J. A. Logan, S. W. Taylor, B. J. Bentz, and J. A. Powell. 2006. Impacts of climate change on range expansion by the mountain pine beetle. Mountain Pine Beetle Initiative Working Paper 2006-14. Canadian Forest Service, Pacific Forestry Centre, Victoria.First citation in articleGoogle ScholarCerezke, H. F. 1995. Egg gallery, brood production, and adult characteristics of mountain pine beetle, Dendroctonus ponderosae Hopkins (Coleoptera: Scolytidae), in three pine hosts. Canadian Entomologist 127:955–965.First citation in articleCrossrefGoogle ScholarGayathri Samarasekera, G. D. N., N. V. Bartell, B. S. Lindgren, J. E. Cooke, C. S. Davis, P. M. A. James, D. W. Coltman, K. E. Mock, and B. W. Murray. 2012. Spatial genetic structure of the mountain pine beetle (Dendroctonus ponderosae) outbreak in western Canada: historical patterns and contemporary dispersal. Molecular Ecology 21:2931–2948.First citation in articleCrossref MedlineGoogle ScholarGilbert, E., J. A. Powell, J. A. Logan, and B. J. Bentz. 2004. Comparison of three models predicting developmental milestones given environmental and individual variation. Bulletin of Mathematical Biology 66:1821–1850.First citation in articleCrossref MedlineGoogle ScholarKelley S. T., B. D. Farrell, and J. B. Mitton. 2000. Effects of specialization on genetic differentiation in sister species of bark beetles. Heredity 84:218–227.First citation in articleCrossref MedlineGoogle ScholarLangor, D. W. 1989. Host effects on the phenology, development, and mortality of field populations of the mountain pine beetle, Dendroctonus ponderosae Hopkins (Coleoptera: Scolytidae). Canadian Entomologist 121:149–157.First citation in articleCrossrefGoogle ScholarLangor, D. W., J. R. Spence, and G. R. Pohl. 1990. Host effects on fertility and reproductive success of Dendroctonus ponderosae Hopkins (Coleoptera: Scolytidae). Evolution 44:609–618.First citation in articleCrossref MedlineGoogle ScholarLogan, J. A., and G. D. Amman. 1986. A distribution model for egg development in mountain pine beetle. Canadian Entomologist 118:361–372.First citation in articleCrossrefGoogle ScholarLogan, J. A., W. W. Macfarlane, and L. Willcox. 2010. Whitebark pine vulnerability to climate-driven mountain pine beetle disturbance in the Greater Yellowstone Ecosystem. Ecological Applications 20:895–902.First citation in articleCrossref MedlineGoogle ScholarMitton, J. B., and S. M. Ferrenberg. 2012. Mountain pine beetle develops an unprecedented summer generation in response to climate warming. American Naturalist 179:E163–E171.First citation in articleLinkGoogle Scholar———. 2014. Data from: Field studies demonstrate bivoltinism in the mountain pine beetle. American Naturalist, Dryad Digital Repository, http://dx.doi.org/10.5061/dryad.4df03.First citation in articleGoogle ScholarReid, R. W. 1962. Biology of the mountain pine beetle, Dendroctonus monticolae Hopkins, in the East Kootenay region of British Columbia. I. Life cycle, brood development, and flight periods. Canadian Entomologist 94:531–538.First citation in articleCrossrefGoogle ScholarReid, R. W., and H. Gates. 1970. Effect of temperature and resin on hatch of eggs of the mountain pine beetle (Dendroctonus ponderosae). Canadian Entomologist 102:617–622.First citation in articleCrossrefGoogle ScholarStock, M. W., and G. D. Amman. 1980. Genetic differentiation among mountain pine beetle populations from lodgepole pine and ponderosa pine in northeast Utah. Annals of the Entomological Society of America 73:472–478.First citation in articleCrossrefGoogle ScholarSturgeon, K. B., and J. B. Mitton. 1986. Biochemical diversity of Pinus ponderosa Laws. and predation by bark beetles, Dendroctonus spp. (Coleoptera: Scolytidae). Journal of Economic Entomology 79:1064–1068.First citation in articleCrossrefGoogle ScholarTishmack, J., S. A. Mata, and J. M. Schmid. 2005. Mountain pine beetle emergence from lodgepole pine at different elevations near Fraser, CO. Research Paper RMRS-RN-27. USDA Forest Service, Fort Collins, CO.First citation in articleGoogle ScholarNatural History Editor: Mark A. McPeek Previous articleNext article DetailsFiguresReferencesCited by The American Naturalist Volume 184, Number 6December 2014 Published for The American Society of Naturalists Article DOIhttps://doi.org/10.1086/678404 Views: 648Total views on this site Citations: 4Citations are reported from Crossref HistorySubmitted April 04, 2014Accepted July 19, 2014Published online November 12, 2014 Keywordsbark beetlesclimate changedegree daysDendroctonusgeneration timePinusvoltinism© 2014 by The University of Chicago. All rights reserved.PDF download Crossref reports the following articles citing this article:Mélodie Kunegel-Lion, Rory L. McIntosh, Mark A. Lewis Management assessment of mountain pine beetle infestation in Cypress Hills, SK, Canadian Journal of Forest Research 49, no.22 (Feb 2019): 154–163.https://doi.org/10.1139/cjfr-2018-0301Jessica RK Forrest Complex responses of insect phenology to climate change, Current Opinion in Insect Science 17 (Oct 2016): 49–54.https://doi.org/10.1016/j.cois.2016.07.002Scott Ferrenberg, Alexander S. Martinez, Akasha M. Faist Aboveground and belowground arthropods experience different relative influences of stochastic versus deterministic community assembly processes following disturbance, PeerJ 4 (Oct 2016): e2545.https://doi.org/10.7717/peerj.2545Lulu Dai, Mingyuan Ma, Chunyan Wang, Qi Shi, Ranran Zhang, Hui Chen Cytochrome P450s from the Chinese white pine beetle, Dendroctonus armandi (Curculionidae: Scolytinae): Expression profiles of different stages and responses to host allelochemicals, Insect Biochemistry and Molecular Biology 65 (Oct 2015): 35–46.https://doi.org/10.1016/j.ibmb.2015.08.004
更多查看译文
关键词
bark beetles,climate change,degree days,Dendroctonus,generation time,Pinus,voltinism
AI 理解论文
溯源树
样例
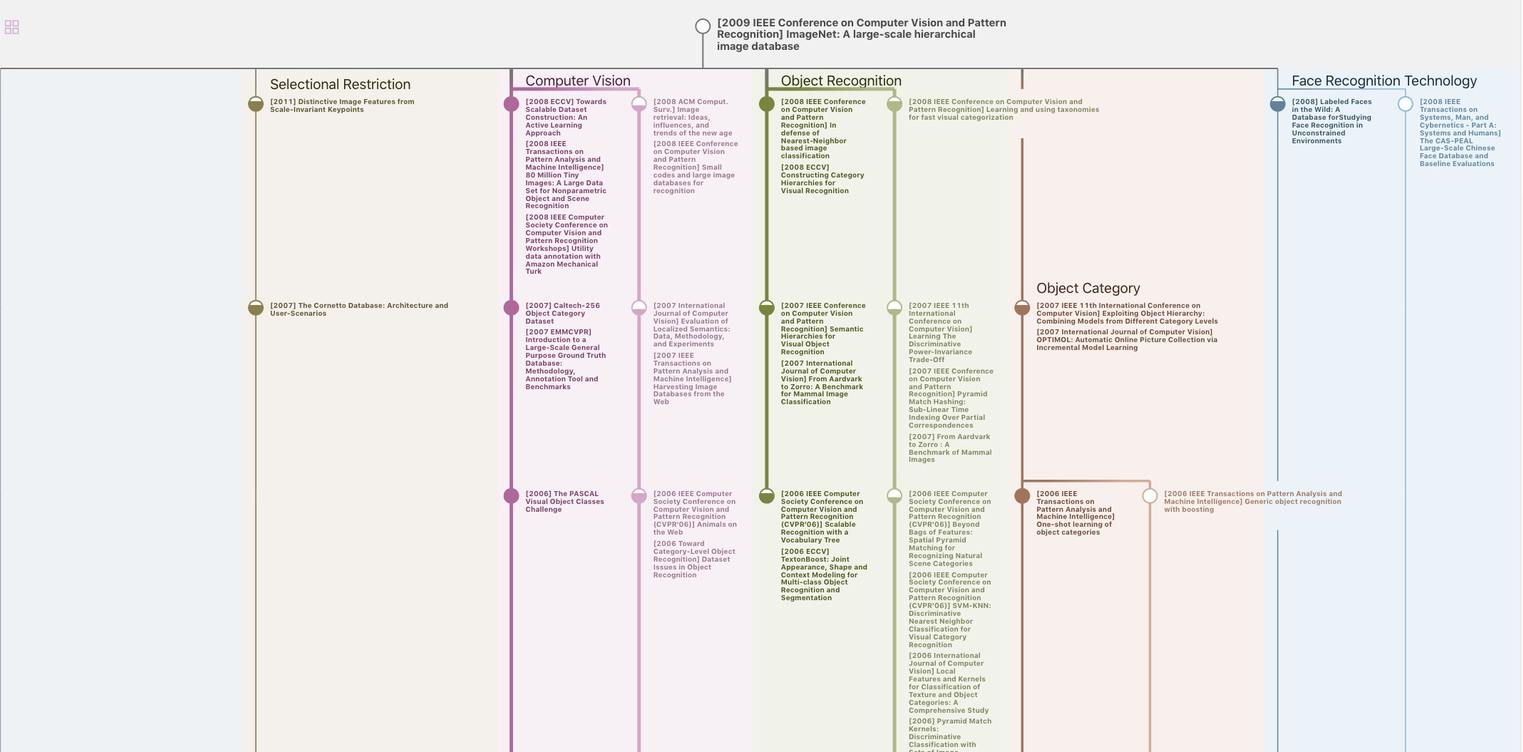
生成溯源树,研究论文发展脉络
Chat Paper
正在生成论文摘要