Different role of isoproterenol and NOS inhibitors on salivary ducts of rats
Micron(2009)
摘要
Objectives Nitric oxide (NO) is a diffusible intracellular messenger that is present in saliva. Chronic treatment with isoproterenol, a β receptor agonist, stimulates the release of NO from acinar cells and induces salivary gland hypertrophy. The aim of this study was to investigate the effect of NO synthesis inhibitors and isoproterenol on rat salivary glands. We analyzed salivary gland weight and the number of ducts per unit area (0.5 mm 2 ) by NADPH-diaphorase histochemistry (to identify the presence of the enzyme NO synthase—NOS) and haematoxylin-and-eosin (HE). Methods For 8 days male Wistar rats received daily single intraperitoneal injections of saline or a NOS inhibitor (40 mg/kg N ω -nitro- l -arginine L-NOARG or N ω -nitro- l -arginine methyl ester L-NAME). This was followed, 30 min later, by subcutaneous injection of isoproterenol (2 or 5 mg/kg) or saline. Results Isoproterenol increased parotid and submandibular gland weights. Isoproterenol (2 mg/kg) induced a decrease of ducts per unit area inversely correlated to the weight of the parotid gland. This effect was augmented by L-NAME. In the submandibular gland L-NAME attenuated isoproterenol (2 mg/kg) weight increase. In the submandibular gland isoproterenol and NOS inhibitors induced an increase in ducts per unit area (HE and NADPH-diaphorase). No effect was observed in the sublingual gland. Conclusion To our knowledge this is the first description of isoproterenol and NOS inhibitors increasing duct density in the submandibular gland. Our results corroborate the hypothesis that NO plays different roles in parotid and submandibular glands. Keywords Nitric oxide Nitric oxide synthase inhibitors NADPH-diaphorase Salivary gland hypertrophy Isoproterenol 1 Introduction Nitric oxide (NO) is a diffusible intracellular messenger that acts in the periphery as a nonadrenergic-noncholinergic neurotransmitter. It mediates nerve-evoked increases in salivary flow, regulates blood flow, induces protein secretion and controls inflammation ( Vág et al., 1998; Buckle et al., 1995; Sayardoust and Ekström, 2004; Rosignoli et al., 2004 ). NO is generated from L-arginine by NO synthase (NOS) enzymes which exists in three isoforms, namely neuronal, endothelial and inducible ( Zhang and Snyder, 1995 ). The names reflect the tissues of origin in which the protein and cDNA were originally found; however, it is now known that all three isoforms are much more widely distributed. Nicotinamide Adenine Dinucleotide Phosphate-diaphorase (NADPH-d) histochemical staining can be used to localize NOS at cellular and subcellular level ( Morris et al., 1997; Hope et al., 1991 ). In the mammalian salivary glands NADPH-d/NOS is found in nerve endings surrounding glandular tissue and in acinar and ductal cells ( Alm et al., 1997; Lohinai et al., 1995; Mitsui et al., 1997; Mitsui and Furuyama, 2000; Soinila et al., 1996; Xu et al., 1997; Tobin et al., 1997; Takai et al., 1999 ). In the rat parotid gland the neuronal isoform of the NADPH-d/NOS is predominant, corresponding to approximately 74% of the activity of NADPH-d/NOS in the cytosol ( Mitsui and Furuyama, 2000 ). NO has been implicated in mechanisms mediating nerve-evoked responses in several organs, including salivary glands ( Edwards et al., 1996 ). Several lines of evidence suggest that NO and its main intracellular target, cyclic guanosine monophosphate (cGMP), modulate salivary gland functions ( Proctor and Carpenter, 2007 ). In the submandibular gland NOS inhibitors such as N ω -nitro- l -arginine methyl ester (L-NAME) and monomethyl- l -arginine (L-NMMA) induced a decrease of salivary flow ( Takai et al., 1999 ). Additionally, in parotid acinar cells NO produced an increase in the cellular cGMP ( Tritsaris et al., 2000; Sugiya et al., 1998 ). Recent studies have suggested that adrenergic and nitrergic transmission could interact in salivary glands. The secretion of amylase from rat parotid lobules induced by isoproterenol in vitro is partially dependent on the mobilization of the NO/cGMP intracellular pathway ( Looms et al., 2002; Sayardoust and Ekström, 2003 ). Parotid acinar cells loaded with a fluorescent indicator of NO increased their fluorescence strongly upon exposure to isoproterenol ( Looms et al., 2002 ). In addition, mitotic cellular responses in salivary glands induced by sympathetic electrical stimulation depend on NO synthesis ( Ekström et al., 2004 ). Chronic treatment with isoproterenol induces enlargement of the parotid and submandibular glands ( Seyle et al., 1961 ) with morphological changes some of which resemble those found in patients with cystic fibrosis ( Peter et al., 1995 ). These changes include a predominant increase in the number and size of the acinar cells and a decline in the number of granular convoluted tubule cells ( Peter et al., 1995 ). The increase in number (hyperplasia) and size (hypertrophy) of acinar cells concurs to gland's enlargement ( Seyle et al., 1961; Onofre et al., 1997; Schneyer, 1962 ). This growth is accompanied by an increase in the synthesis of DNA of protein and changes in RNA transcription with up- or down-regulation of genes expressed in acinar cells ( Hagen et al., 2002 ). The present study was aimed at analyzing the involvement of NO on morphological changes in salivary glands induced by subchronic isoproterenol treatment. We investigated the effects of pretreatment with NADPH-d/NOS inhibitors on isoproterenol-induced hypertrophy on major salivary glands through evaluation of gland weight and ducts per unit area. 2 Material and methods 2.1 Animals Male Wistar rats (250 ± 30 g) with free access to food and water were housed, four per cage. Room temperature was 23 °C (±1) and the daylight cycle was maintained artificially (lights on from 07:00 to 19:00 h). All rats used in the study were drug naive. All experiments were carried out in accordance to standard protocols of the Ethic Committee of Sao Paulo University (USP) and all efforts were made to minimize animal suffering. 2.2 Drugs Isoproterenol (+)-bitartrate salt (Sigma, USA) N ω -nitro- l -arginine (L-NOARG, Sigma, USA), N ω -nitro- l -arginine methyl ester (L-NAME, Sigma, USA) and Urethane 99% (H 2 NCO 2 C 2 H 5 , Aldrich, USA) were dissolved in 0.9% saline and injected in a volume of 2 ml/kg. 2.3 Experimental design For 8 days animals received a single daily intraperitoneal (i.p.) injection of saline, L-NAME or L-NOARG followed, 30 min later, by saline or isoproterenol (subcutaneous, s.c., either 2 or 5 mg/kg). The doses of L-NAME and L-NOARG were chosen based on studies from the literature showing that they can inhibit in vivo NOS activity in the central nervous system ( Salter et al., 1995 ). The route and doses of isoproterenol administration were also based on other studies ( Tomich and Eversole, 1972; Chisholm and Adi, 1995; Kawaguchi et al., 1997 ). The following experiments were performed: Experiment 1: Isoproterenol-induced hypertrophy on salivary glands . In our hands the dose of 10 mg/kg (daily) of isoproterenol induced a high mortality rate. Therefore, rats (5–10 per group) received daily single injections of saline or isoproterenol (2 or 5 mg/kg, s.c.), for 8 days. Experiment 2: Effects of L-NAME on isoproterenol-induced hypertrophy . Rats (5–6 per group) received daily (8 days) single injections of saline or L-NAME (40 mg/kg, i.p.) followed, 30 min later, by saline or isoproterenol (2 and 5 mg/kg, s.c.). Experiment 3: Effect of L-NOARG on isoproterenol-induced hypertrophy. Rats (5–8 per group) received daily (8 days) single injections of saline or L-NOARG (40 mg/kg, i.p.) followed, 30 min later, by saline or isoproterenol (2 mg/kg, s.c.). 2.4 Glandular tissue One day after the end of the treatments the animals were anaesthetized with urethane 37.5% (1.5 g/kg; 4 ml/kg, i.p.). Parotid, submandibular and sublingual glands were bilaterally removed. Connective tissue and lymph nodes were carefully removed from glands that were individually weighed (BG 200-GEHAKA ® , USA) then soaked for 2 h in a 4% paraformaldehyde/0.1 M phosphate-buffer solution (pH 7.4, 4 °C) and cryoprotected in 15% saccharose/0.1 M phosphate-buffer solution overnight. Glands were quick-frozen in dry ice and stored at −70 °C. 2.5 NADPH-d/NOS histoenzymology Fourteen-micron sections were then cut with a cryostat and collect on gelatin-covered slides. Tissue was processed for routine HE staining and NADPH-d/NOS histochemistry according to Vincent and Kimura (1992) . Briefly, sections were incubated in 0.1 M phosphate buffer, pH 7.4, containing 0.3% Triton X-100, 0.1 mg/ml nitrobluetetrazolium and 1.0 mg/ml β-NADPH at 37 °C for 60 min in the dark. The slides were then dipped for 10 min in phosphate buffer, dried, rinsed in distilled water, dried again and mounted for microscopic observation. Glandular tissue from the same experiment was always processed in the same assay. 2.6 Ducts per unit area evaluation Stained ducts on adjacent sections were analyzed using a computerized image analysis system from the National Institute of Health (Image software 9.0, W. Rasband, National Institute of Mental Health http://rsb.info.nih.gov/nih-image/ ). Images were captured from tissue slices using a Leica microscope and CCD camera. The criteria used to select one to three sections in each slice were staining homogeneity and histological integrity. For evaluation of NADPH-d and HE stained ducts per unit area the average number of striated and intercalated ducts from three randomly selected gland regions of a fixed size/area (0.5 mm 2 ) was measured. A mean value representing ducts per unit area (0.5 mm 2 ) in each gland section was then calculated. The measurements were conducted bilaterally by an experimenter blind to the treatment groups. 2.7 Statistical analyses Data were analyzed by repeated-measures analyses of variance (ANOVA) with treatment as between-subjects and side (right or left gland) as repeated measure factors. Since there was no difference between right and left glands, the results were presented as means of both sides. The effects of drug treatment were compared by one-way analysis of variance (ANOVA) followed by the Duncan test for multiple comparisons ( p < 0.05). A possible association between NADPH- and HE-duct density and gland weight was test by Pearson's correlation analysis. 3 Results 3.1 Isoproterenol-induced hypertrophy of salivary glands Isoproterenol (2 and 5 mg/kg) promoted a significant increase (2–3-fold) in parotid [ F (2,21) = 90.14; Duncan p < 0.05] and submandibular wet glandular weights [ F (2,21) = 35.57; Duncan p < 0.05]. No weight change was found in the sublingual gland ( p = 0.139; Fig. 1 ). Treatment with L-NAME or L-NOARG did not modify glandular weights ( Tables 1 and 2 ). L-NAME induced a small but significant attenuation of the weight gain induced by isoproterenol 2 mg/kg in the submandibular gland [ F (5,36) = 13.59; Duncan p < 0.05, Table 1 ]. L-NOARG, however, failed to do so ( Table 2 , p > 0.05). 3.2 Salivary glands histological parameters The parenchyma of the salivary glands consists of intercalated ducts, convoluted granular ducts, striated ducts and acinar cells. In the submandibular gland of control rats NADPH-d/NOS positive signal was present in salivary ducts ( Fig. 2 ; 1, 2 and 4), blood vessels ( Fig. 2 , 3[B]), axons ( Fig. 2 , 2[A]) and autonomic ganglia ( Fig. 2 , 3[C]). Salivary ducts showed great variation in staining intensity between individual epithelial cells so that in the same duct some cells showed intense reactivity while others were completely non-reactive. In the parotid gland isoproterenol (2 mg/kg) induced a decrease on ducts per unit area measured either by HE [ F (7,46) = 18.12; Duncan p < 0.05; Fig. 3 ] or NADPH-d/NOS histochemistry [ F (7,46) = 40.15; Duncan p < 0.05; Fig. 4 ]. NADPH-d/NOS inhibitors did not prevent this effect. However, the decrease in NADPH-d/NOS ducts per unit area induced by isoproterenol was enhanced by pre-treatment with L-NAME ( Fig. 4 ). In the submandibular gland isoproterenol 2 mg/kg increased the HE-labeled ducts [ F (7,39) = 13.79; Duncan p < 0.05; Fig. 3 ]. NADPH-d/NOS inhibitors did not prevent this effect. In addition NOS inhibitors by themselves induced an increase of HE [ F (7,46) = 13.00; Duncan p < 0.05; Fig. 3 ] and NADPH-d/NOS-positive ducts [ F (7,47) = 13.79; Duncan p < 0.05; Fig. 4 ]. In the sublingual gland isoproterenol did not change ducts per unit area. L-NOARG induced a discrete increase in positive NADPH-d/NOS ducts [ F (7,38) = 4.92; Duncan p < 0.05; Fig. 4 ]. In the parotid gland NAPDH-d/NOS and HE-labeled cells were inversely correlated with the weight increase ( r = −0.81 and r = −0.88, respectively, Person's coefficient, p < 0.01; Fig. 5 ). In contrast there was a positive correlation between NADPH-d/NOS and HE-labeled ducts in the submandibular gland (L-NAME group, parotid r = 0.88, and submandibular r = 0.68; L-NOARG group, parotid r = 0.79, and submandibular r = 0.68). 4 Discussion As in our experiments, earlier studies have shown that NOS-positive cells are present in the salivary glands. For example, Takai et al. (1999) and Lohinai et al. (1995) report that NADPH-d/NOS-positive nerve fibers were richly distributed around acini in the submandibular gland. In the rat submandibular gland, Soinila et al. (1996) reported that NADPH-d/NOS-positive nerve fibers occur occasionally, but they did not have a periacinar pattern. However, Alm et al. (1997) reported a rather different distribution pattern of NADPH-d/NOS-positive fibers in this gland, i.e., the positive fibers were more richly distributed around acini and granular ducts. This evidence suggests that neuronal-constitutive NADPH-d/NOS fibers in the salivary glands are secretomotor in nature. The presence of NADPH-d/NOS-positive fibers surrounding the duct cells suggests a functional role for NO. Saliva is initially produced as an isotonic primary secretion in the salivary acini ( Tandler et al., 1998 ). The primary fluid is modified by ductal reuptake of sodium and chloride and secretion of potassium and bicarbonate as it flows through the ducts. The saliva is finally discharged as a hypotonic fluid into the oral cavity ( Nezu et al., 2005 ). It seems that these ducts may play a subsidiary role in the formation of saliva. Ducts, however, can function as reservoirs of pluripotent cells that can differentiate into acinar cells ( Tandler et al., 1998 ). Moreover, in the rat submandibular gland the duct tend to become hyperplasic with advancing age ( Slavin et al., 1989 ). The presence of NADPH-d/NOS immunoreactivity in the duct epithelium may be the source of nitrite secreted into the human saliva ( Soinila et al., 2006 ). These NADPH-d/NOS-containing may have a paracrine function involved in nitrergic regulation of saliva secretion ( Kugler et al., 1994 ). Under physiological conditions, the release of NO contributes to the regulation of vascular resistance and blood flow ( Vág et al., 1998; Lohinai et al., 1996 ). NO can also be a signaling molecule for the maintenance of salivary gland homeostasis ( Looms et al., 2002 ). It can diffuse outside the acinar cells to the surrounding tissue promoting adequate blood supply during long-term secretion and regulating cell growth and differentiation ( Ozmeric and Çankal, 2006 ). The L-arginine/NO pathway may also play a negative modulatory role in the cholinergic control of salivary fluid and amylase secretion ( Lohinai et al., 1997, 1999 ). L-NAME, markedly decreased parasympathetic secretory responses in the submandibular gland of cats ( Edwards et al., 1996 ) and ferrets ( Tobin et al., 1997 ). Elevated NO production may contribute to inflammatory damage and acinar cell atrophy in Sjogren's syndrome and could acts as a mediator of dry mouth ( Konttinen et al., 1997 ). Further studies confirmed a role of NO in mediating protein secretion in response to parasympathetic and sympathetic stimulation ( Proctor and Carpenter, 2007 ). It is also possible that NO participates in the feedback process modulating pre-synaptic release of neurotransmitters/peptides from the nerve endings ( Proctor and Carpenter, 2007 ). Such effect allows an interaction between the two branches of the autonomic nervous system working concurrently to create secretory activation of the salivary gland ( Proctor and Carpenter, 2007 ). Isoproterenol is a potent sialogogo that stimulates the increase of NO on acinar cells ( Looms et al., 2002 ) . In vitro responses of rat parotid tissue to isoproterenol include a NO-dependent rise in cGMP ( Tritsaris et al., 2000; Ekström et al., 2004 ). Moreover, increases in the synthesis of secretory proteins and mitotic activity following electrical stimulation of the sympathetic nerves are to a large extent dependent on β-adrenoceptor-mediated generation of NO ( Sayardoust and Ekström, 2004; Ekström et al., 2004 ). Early studies reported a decrease in the number of HE-labeled ducts in parotid gland following isoproterenol treatment ( Tomich and Eversole, 1972 ). Schneyer (1962) suggested that hyperplasic acinar cells could replace part of the ductal system, with the remaining ducts becoming increasingly compressed. Accordingly, Onofre et al. (1997) suggested that acinar cells are more prone to suffer hypertrophy processes than the duct system following isoproterenol. We cannot exclude the possibility that in the parotid hypertrophied tissue the ducts could be farther from each other, in consequence their number in a unit area will decrease but not in total tissue. Several studies in the literature suggest functional differences among the salivary glands. The response of salivary cells appears to be different to similar stimulus depend on the subtype of receptor stimulated and probably also on the differences in receptor regulation within each gland ( Bush et al., 2002 ). For example, the regulatory mechanisms of amylase secretion differ between the parotid and submandibular glands ( Bush et al., 2002 ). Also, there is a considerable difference in radiosensitivity between the two glands ( Coppes et al., 2002 ). Interestingly, NO-dependent vasoregulation may vary between the glands. In the cat NO generation in the parotid gland is less important to blood flow control than in the submandibular gland ( Lohinai et al., 1996 ). Takai et al. (1999) suggested that NO influences parasympathic-mediated effects in the submandibular but not in the sublingual gland. Rosignoli and Peres Leirós (2002) demonstrated that muscarinic type 3 (M 3 ) acetylcholine receptors are expressed in the rat parotid gland. M 3 receptor is coupled to constitutive NOS activation and NO-mediating signaling sensitive to protein kinase C regulation. In contrast, both M 3 and muscarinic type 1 receptor are expressed in the submandibular gland. Isoproterenol induces increase of cAMP in both salivary glands, but while in the parotid it triggers amylase release, in the submandibular it does not ( Bush et al., 2002 ). Acknowledgements The authors are grateful to Ms. Renata Ferreira for helpful technical support and to Prof. John Nicholls of Department of Neurobiology—SISSA Trieste for the manuscript discussion and suggestions. This research program has been supported by FAPESP, CNPq and CAPES. References Alm et al., 1997 P. Alm J. Ekström B. Larsson G. Tobin K.E. Andersson Nitric oxide synthase immunoreactive nerves in rat and ferret salivary glands and effects of denervation Histochem. J. 29 1997 669 676 Buckle et al., 1995 A.D. Buckle S.J. Parker S.R. Bloom A.V. Edwards The role of nitric oxide in the control of protein secretion in the submandibular gland of the cat Exp. Physiol. 80 1995 019 1030 Bush et al., 2002 L. Bush L.S. Borda E. Borda Differences in the regulatory mechanism of amylase release by rat parotid and submandibular gland Arch. Oral Biol. 47 2002 717 722 Chisholm and Adi, 1995 D.M. Chisholm M.M. Adi Cell proliferation and apoptosis in isoprenaline-induced sialosis in the rat submandibular glands Int. J. Exp. Pathol. 76 1995 263 269 Coppes et al., 2002 R.P. Coppes A. Vissink A.W. Konings Comparison of radiosensitivity of rat parotid and submandibular glands after different radiation schedules Radiother. Oncol. 63 2002 321 328 Edwards et al., 1996 A.V. Edwards G. Tobin J. Ekström S.R. Bloom Nitric oxide and release of the peptide VIP from parasympathetic terminals in the submandibular gland of the anaesthetized cat Exp. Physiol. 80 1996 349 359 Ekström et al., 2004 J. Ekström S. Sayardoust H. Cevit Nitric oxide-dependent mitotic activity in salivary glands of the rats upon sympathetic stimulation Arch. Oral Biol. 49 2004 889 894 Hagen et al., 2002 K.G.T. Hagen M.M. Balys L.A. Tabak J.E. Melvin Analysis of isoproterenol-induced changes in parotid gland gene expression Physiol. Genomics 8 2002 107 114 Hope et al., 1991 B.T. Hope G.J. Michael K.M. Knigge S.R. Vincent Neuronal NADPH diaphorase is a nitric oxide synthase Proc. Natl. Acad. Sci. 88 1991 2811 2814 Kawaguchi et al., 1997 T. Kawaguchi S. Murai H. Saito T. Itoh Changes in the noradrenaline and acetylcholine content of three major salivary glands and in the salivation and protein component patterns of whole saliva in chronically isoprenaline-administered mice Arch. Oral Biol. 42 1997 225 234 Konttinen et al., 1997 Y.T. Konttinen L.A. Platts S. Tuominen K.K. Eklund N. Santarvirta J. Törnwall T. Sorsa M. Hukkanen J.M. Polak Role of nitric oxide in Sjögren's syndrome Arthritis Rheum. 40 1997 875 883 Kugler et al., 1994 P. Kugler D. Höfer B. Mayer Drenckhahn Nitric oxide synthase and NADP-linked glucose-6-phosphate dehydrogenase are co-localized in brush cells of rat stomach and pancreas J. Histochem. Cytochem. 42 1994 1317 1321 Lohinai et al., 1995 Z. Lohinai A.D. Székely L. Soós E. Fehér Distribution of nitric oxide synthase containing elements in the feline submandibular gland Neurosci. Lett. 192 1995 9 12 Lohinai et al., 1996 Z. Lohinai I. Balla J. Marczis Z. Vass A.G.B. Kovách The effect of a nitric oxide donor and an inhibitor of nitric oxide synthase on blood flow and vascular resistance in feline submandibular, parotid and pancreatic glands Arch. Oral Biol. 41 1996 699 704 Lohinai et al., 1997 Z. Lohinai B. Burghardt T. Zelles G. Varga The effect of L-arginine/nitric oxide pathway on salivary amylase secretion in conscious rats J. Physiol. Paris 91 1997 217 221 Lohinai et al., 1999 Z. Lohinai B. Burghardt T. Zelles G. Varga Nitric oxide modulates salivary amylase and fluid, but not epidermal growth factor secretion in conscious rats Life Sci. 64 1999 953 963 Looms et al., 2002 D.K. Looms K. Tritsaris A.M. Pedersen B. Nauntofte S. Dissing Nitric oxide signaling in salivary glands J. Oral Pathol. Med. 31 2002 569 584 Mitsui and Furuyama, 2000 Y. Mitsui S. Furuyama Characterization of nitric oxide synthase in the rat parotid gland Arch. Oral Biol. 45 2000 531 536 Mitsui et al., 1997 Y. Mitsui N. Yasuda S. Furuyama H. Sugiya Nitric oxide synthase activities in mammalian parotid and submandibular salivary glands Arch. Oral Biol. 42 1997 621 624 Morris et al., 1997 B.J. Morris C.S. Simpson S. Mundell K. Maceachern H.M. Johnston A.M. Nolan Dynamic changes in NADPH-diaphorase staining reflect activity of nitric oxide synthase: evidence for a dopaminergic regulation of striatal nitric oxide release Neuropharmacology 36 1997 1589 1599 Nezu et al., 2005 A. Nezu T. Morita A. Tanimura Y. Tojyo Comparison of agonist-induced Ca 2+ responses in rat submandibular acini and ducts Arch. Oral Biol. 50 2005 585 592 Onofre et al., 1997 M.A. Onofre L.B. Souza A.J. Campos R. Taga Stereological study of acinar growth in the rat parotid gland induced by isoproterenol Arch. Oral Biol. 42 1997 333 338 Ozmeric and Çankal, 2006 N. Ozmeric D.U. Çankal A multifaceted molecule, nitric oxide in oral and periodontal diseases Clin. Chim. Acta 366 2006 90 100 Peter et al., 1995 B. Peter M.A. Van Waarde A.E.J. Vissink Degranulation of rat salivary glands following treatment with receptor-selective agonists Clin. Exp. Pharmacol. Physiol. 22 1995 330 336 Proctor and Carpenter, 2007 G.B. Proctor G.H. Carpenter Regulation of salivary gland function by autonomic nerves Autonomic Neurosci. Basic Clin. 133 2007 3 18 Rosignoli and Peres Leirós, 2002 F. Rosignoli C. Peres Leirós Activation of nitric oxide synthase through muscarinic receptors in rat parotid gland Eur. J. Pharmacol. 439 2002 27 33 Rosignoli et al., 2004 F. Rosignoli V. Roca R. Meiss N. Pregi C.P. Leirós Inhibition of calcium-calmodulim kinase restores nitric oxide production and signaling in submandibular glands of a mouse model of salivary dysfunction Br. J. Pharmacol. 143 2004 1058 1065 Salter et al., 1995 M. Salter C. Duffy R. Hazelwood Determination of brain nitric oxide synthase inhibition in vivo: ex vivo assays of nitric oxide synthase can give incorrect results Neuropharmacology 34 1995 327 334 Sayardoust and Ekström, 2003 S. Sayardoust J. Ekström Nitric oxide-dependent in vitro secretion of amylase from innervated or chronically denervated parotid glands of the rat in response to isoprenaline and vasoactive intestinal peptide Exp. Physiol. 88 2003 381 387 Sayardoust and Ekström, 2004 S. Sayardoust J. Ekström Nitric oxide dependent protein synthesis in parotid and submandibular glands of anaesthetized rats upon sympathetic stimulation or isoprenaline administration Exp. Physiol. 89 2004 219 227 Schneyer, 1962 C.A. Schneyer Salivary gland changes after isoproterenol-induced enlargement Am. J. Physiol. 203 1962 232 236 Seyle et al., 1961 H. Seyle R. Veilleux M. Cantin Excessive stimulation of salivary gland growth by isoproterenol Science 133 1961 44 45 Slavin et al., 1989 B.G. Slavin W.J. Paule S. Bernick Morphological changes in the submandibular gland of aging rats Gerodontology 8 1989 53 58 Soinila et al., 1996 S. Soinila S. Vanhatalo A. Lumme N. Back J. Soinila Nitric oxide synthase in the autonomic and sensory ganglia innervating the submandibular salivary gland Microsc. Res. Tech. 35 1996 32 43 Soinila et al., 2006 J. Soinila K. Nuorva S. Soinila Nitric oxide synthase in human salivary glands Histochem. Cell Biol. 125 2006 717 723 Sugiya et al., 1998 H. Sugiya H. Michikawa Y. Mitsui J. Fujita- Oshigaki M. Hara-Yokoyama S. Furuyama Ca 2+ -nitric oxide-cGMP signalling in rabbit parotid acinar cells Eur. J. Morphol. 36 1998 194 197 Takai et al., 1999 N. Takai K. Uchihashi K. Higuchi Y. Yoshida M. Yamaguchi Localization of neuronal-constitutive nitric oxide synthase and secretory regulation by nitric oxide in the rat submandibular and sublingual glands Arch. Oral Biol. 44 1999 745 750 Tandler et al., 1998 B. Tandler T. Nagato K. Toyoshima C.J. Phillips Comparative ultrastructure of intercalated ducts in major salivary glands: a review Anat. Rec. 252 1998 64 91 Tobin et al., 1997 G. Tobin A.V. Edwards S.R. Bloom J. Ekström Nitric oxide in the control of submandibular function in the anaesthetized ferret Exp. Physiol. 82 1997 825 836 Tomich and Eversole, 1972 C.E. Tomich L.R. Eversole The enzyme histochemistry of isoproterenol-induced salivary gland hyperplasia Oral Surg. 33 1972 57 871 Tritsaris et al., 2000 K. Tritsaris D.K. Looms B. Nauntofte S. Dissing Nitric oxide synthesis causes inositol phosphate production and Ca 2+ release in rat parotid acinar cells Pflügers Arch. 440 2000 223 228 Vág et al., 1998 J. Vág C. Hably Z. Csabai H. Tost J. Bartha A. Fazekas Blood flow of the submandibular gland in sodium-depleted and -loaded rats: effect of nitric oxide synthase inhibition Res. Exp. Med. (Berl.) 198 1998 101 108 Vincent and Kimura, 1992 S.R. Vincent H. Kimura Histochemical mapping of nitric oxide synthase in the rat brain Neuroscience 46 1992 755 784 Xu et al., 1997 X. Xu W. Zeng J. Diaz K.S. Lau A.C. Gukovskaya R.J. Brown S.J. Pandol S. Muallem nNOS and Ca 2+ influx in rat pancreatic acinar and submandibular salivary gland cells Cell Calcium 22 1997 217 228 Zhang and Snyder, 1995 J. Zhang S.H. Snyder Nitric oxide in the nervous system Annu. Rev. Pharmacol. Toxicol. 35 1995 213 233
更多查看译文
关键词
Nitric oxide,Nitric oxide synthase inhibitors,NADPH-diaphorase,Salivary gland hypertrophy,Isoproterenol
AI 理解论文
溯源树
样例
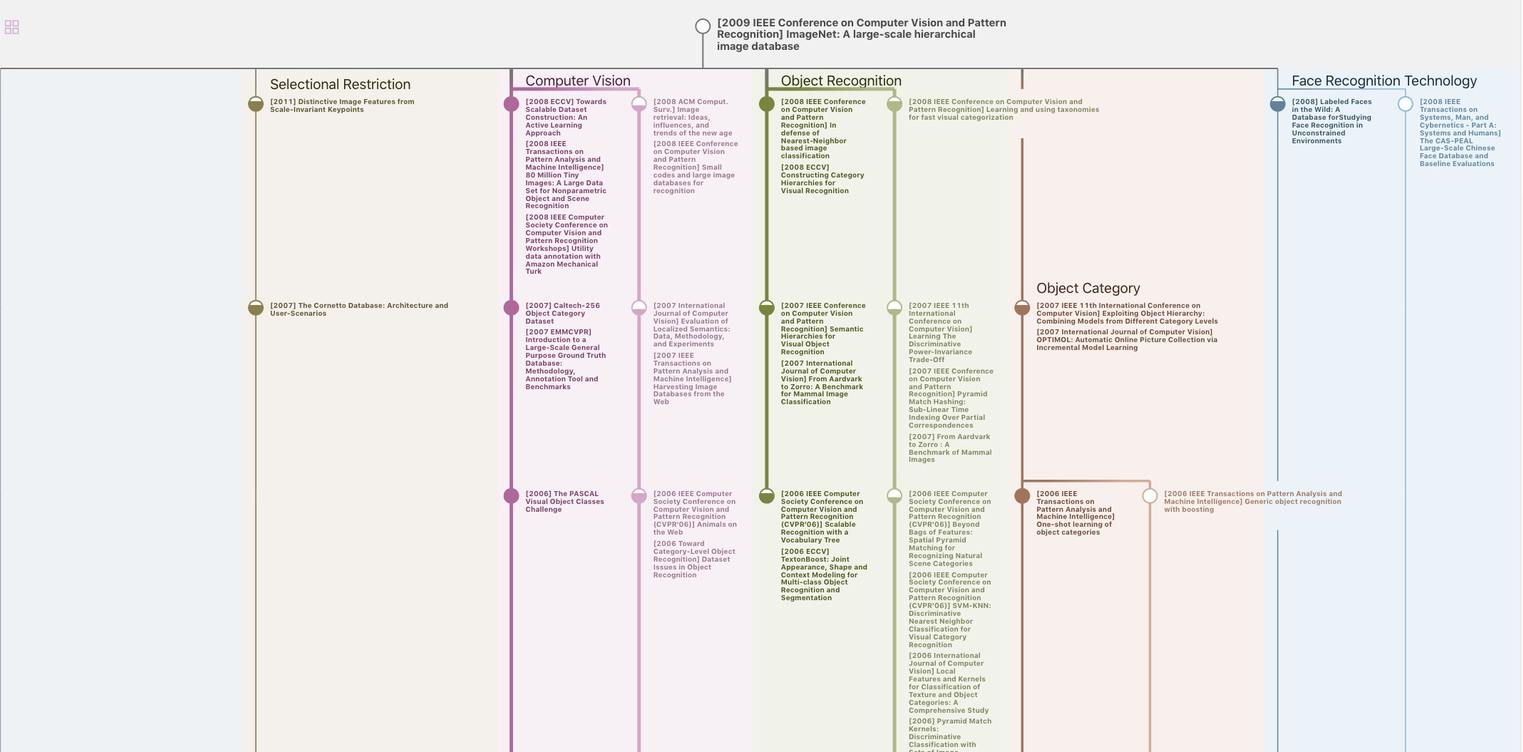
生成溯源树,研究论文发展脉络
Chat Paper
正在生成论文摘要