Preparation of carriers based on magnetic nanoparticles grafted polymer and immobilization for lipase
Biochemical Engineering Journal(2011)
Abstract
Highlights ► Super paramagnetic nanoparticles were prepared by a surface-initiated radical polymerization. ► This nanoparticle was provided with positive charge and active epoxy groups simultaneously. ► The candida rugosa lipase (CRL) was immobilized via electrostatic adsorption and covalent binding. ► The lipase loading amount was 68.3 ± 0.5 mg CRL/g support and the activity recovery reached to 60.4% (±1.6%). Abstract The super paramagnetic nanoparticles Fe 3 O 4 /(2-hydroxyethty methacrylate-co-dimethyl dially ammonium chloride) copolymer, i.e. Fe 3 O 4 /P(HEMA–DMDAAC), with positive charge and active epoxy groups simultaneously, were synthesized by a surface-initiated radical polymerization and activation reaction with epoxy chloropropane. The production particles were characterized by Fourier transform infrared spectroscopy (FT-IR), thermogravimetric analysis (TG), transmission electron microscopy (TEM), and vibrating sample magnetometry (VSM). It was verified that the magnetic microspheres held small diameters of 80–100 nm and displayed super paramagnetic property with saturation magnetization of 38.9 emu/g. The candida rugosa lipase (CRL), meanwhile, was immobilized onto the magnetic microspheres via electrostatic adsorption and covalent binding, the loading amount of lipase was 68.3 ± 0.5 mg CRL/g support and the activity recovery of the obtained immobilized lipase reached to 60.4% (±1.6%). Keywords Super paramagnetism Electrostatic adsorption Covalent binding Immobilized CRL Enzyme technology Reusability 1 Introduction Enzyme, as well known, is preferred to chemical catalyst because of its high effectiveness, high specificity and mild reaction conditions [1] . In recent years, enzyme has become a viable alternative for many applications [2] . Lipase (triacylglycerol acyl hydrolases) is a kind of ubiquitous enzymes with various biological activities, including enantioselective hydrolysis and esterification, chiral resolution, synthesis of enantioenriched monomers and macromolecules for polymerization reactions, and other enzymatic reactions [3–5] . Candida rugosa lipase (CRL), among the lipases from various sources, received much attention due to its high activity and broad specificity [6] . However, the industrial applications of biocatalysts have not yet reached a significant level due to the high costs of the enzymes and the inability in their separation, recycling, and reusing [7] . Thus, the technology of enzyme immobilization, which could achieve the reusability of enzyme and prove the stability of enzyme [8] , was developed to overcome these inconveniences [9] . Until now, a variety of nano-sized particles have been used to immobilize enzyme [10] . However, it is difficult to recover small immobilized enzyme particles from reaction system using conventional carriers. In view of the above disadvantages, functional super paramagnetic iron oxides have attracted great interest in enzyme immobilization [11] . On one hand, the functional magnetic microspheres can be covalently binded with different groups (e.g. amino, hydroxyl, or thiol moieties) on the protein surface to form strong linkages, which can prove the stabilities of enzymes [12] . On the other hand, enzymes immobilized by magnetic microspheres could be separated easily and rapidly from the reaction system to enhance the reusability of enzyme [13] , and they can also be stabilized in a fluidized-bed reactor by applying an external magnetic field [14,15] . In order to increase the loading amount of enzyme on magnetic particles and improve the stability of immobilized enzyme, different organic macromolecules has been used to functionalizing the surface of magnetic particles, such as glycidyl methacrylate (GMA), methyl methacrylate (MMA), 2-hydroxyethty methacrylate (HEMA), methacryloxyethyl trimethyl ammonium chloride (MATAC), etc. [16–18] . During the process of enzyme immobilization, different methods could be adopted to immobilize enzyme, such as covalent binding [11] , entrapment [19] or adsorption [20] , etc. However, it has been proved that immobilization with two methods would greatly increase the enzyme loading amount and improve the stability of the enzyme [21] . Thus, the carrier, which has positive charge and active groups (such as epoxy group, amino group, etc.) simultaneously, would be a kind of efficient supports. In this paper, super paramagnetic Fe 3 O 4 nanoparticles modified by vinyltriethoxysilicane (VTES) were prepared firstly. Then, 2-hydroxyethty methacrylate (HEMA) and dimethyl dially ammonium chloride (DMDAAC) were grafted onto modified nanoparticles by surface-initiated radical polymerization. At last, the magnetic nanoparticles were activated by epoxy chloropropane. HEMA is well known for its non-toxicity, biocompatibility and widespread biomedical applications [22] , while DMDAAC can provide the carriers positive charge. Thus, candida rugosa lipase (CRL) was immobilized on the resulting magnetic microspheres by electrostatic adsorption and covalent binding. The structure and properties of the magnetic carriers were characterized by Fourier transform infrared spectroscopy (FT-IR), thermogravimetric analysis (TG), transmission electron microscopy (TEM), and vibrating sample magnetometry (VSM). Additionally, the optimal conditions of immobilizing lipase were determined and the properties of the immobilized lipase (such as activity recovery, thermal stability reusability and storage ability) were investigated. 2 Chemicals and methods 2.1 Chemicals 2-Hydroxyethty methacrylate (HEMA) and dimethyl dially ammonium chloride (DMDAAC) were obtained from Ciba Specialty Chemicals (China) Ltd., Guangzhou; vinyl triethoxy silane (VTES) was chemical grade and purchased from Wuhan New Materials Co.; azobisisobutyronitrile (AIBN) was chemical grade and purchased from Forth Shanghai Reagent Factory (China); candida rugosa lipase (CRL), Type VII, 1180 units/mg (solid) and bovine serum albumin (BSA) were analytic grade and purchased from Sigma Chemical Co.; other chemicals and solvents were all of analytical grade and obtained from Tianjing Chemical Reagent Company (China). All the reagents of chemical grade were used with further purification. 2.2 Preparation of Fe 3 O 4 nanoparticles Fe 3 O 4 nanoparticles were prepared by a chemical co-precipitation method described previously [23] with some modifications. 35 ml of 1 M FeCl 2 and 1.5 M FeCl 3 solution were added into a three-necked flask to make the molar ratio of Fe 2+ and Fe 3+ maintaining 1:1.5, and stirred together under nitrogen. When the solution was heated to 60 °C, ammonia (25%, w/w) was added to regulate the pH value of reaction system to 10–11, and solution became dark after base addition. Then the solution was heated at 80 °C for 1 h with vigorous stirring. After solution cooled, the precipitates were isolated from reaction system and washed several times with distilled water until the solution became neutral. Finally, the resulting magnetic nanoparticles were obtained after dried at room temperature under vacuum. 2.3 Modification of Fe 3 O 4 nanoparticles with VTES Modification of Fe 3 O 4 nanoparticles with VTES is achieved by the reaction between the hydroxyl groups of the hydrolytic VTES and the hydroxyl groups on the surface of magnetite. 1.0 g of Fe 3 O 4 nanoparticles were dispersed in 60 ml of ethanol and vibrated with ultrasonic for 1 h, then certain NH 3 ·H 2 O (NH 3 ·H 2 O:VTES = 2:1, v/v) was added and ultrasonic vibrated to homogenize for 10 min. With continuous mechanical stirring, 6.0 ml of VTES was added into the reaction mixture. The reaction was heated at 50 °C for 8 h under continuous stirring and nitrogen atmosphere. At last, the products were separated by permanent magnet and then washed thoroughly with ethanol and distilled water until neutral. Finally, the VTES-modified magnetic nanoparticles were obtained after dried at room temperature under vacuum. 2.4 Preparation of magnetic Fe 3 O 4 /P(HEMA–DMDAAC) nanoparticles The magnetic polymeric nanoparticles were synthesized according to the following steps: 0.5 g of VTES-modified Fe 3 O 4 nanoparticles, certain KPS and NaHSO 4 with molar ratio of 1:1, 44 ml of ethanol and 10 ml of distilled water were put in a three-necked flask and vibrated with ultrasonic for 30 min under nitrogen to be dispersed uniformly. After these mixtures were placed at 4 °C overnight, the flask was moved into a water bath at 70 °C and mechanically stirred under a nitrogen atmosphere. Then a mixture of HEMA (4.8 ml, 0.04 mol), DMDAAC (10% of the total weight of monomers), ethanol (7 ml) and deionized water (7 ml) was added dropwise into the flask in 1 h. After this, the polymerization was taken out at 70 °C for 6 h. At last, the products were isolated from solution by magnetic decantation and washed repeatedly with distilled water and ethanol. Then the nanoparticles were extracted with ethanol in soxhlet extraction for 48 h and dried at room temperature in vacuum drying oven for 24 h. 2.5 Activation of magnetic Fe 3 O 4 /P(HEMA–DMDAAC) nanoparticles The hydroxyl group could not combine with lipase efficiently, so the magnetic polymer carriers should be activated by epoxy chloropropane. 4 g of magnetic Fe 3 O 4 /P(HEMA–DMDAAC) nanoparticles were swelled in dimethyl sulfoxide overnight. Then 16 ml of epoxy chloropropane were added into the mixture and the reactants were heated to 40 °C, and 40 ml of NaOH solution (3 mol/l) were added drop-wise into the reaction system. After 4 h, the reaction was finished. The activated products were filtered and washed several times with distilled water until the solution became neutral. Finally, the resulting magnetic nanoparticles were obtained after dried at room temperature under vacuum. Scheme 1 shows the preparation of magnetic epoxy supports including the synthesis of magnetic Fe 3 O 4 /P(HEMA–DMDAAC) nanoparticles and the activation of magnetic supports. 2.6 Characterization of magnetic polymer supports FT-IR spectra from the KBr pellet with OA-Fe 3 O 4 and the magnetic polymer microspheres, respectively, were recorded by a Fourier Transform infrared spectrophotometer (Nicole NEXUS 670, USA). Thermogravimetric analysis was observed by a TG-DSC apparatus (NETZSCH STA 449C) by heating the samples from room temperature to 900 °C under Ar atmosphere at a heating rate of 20.0 K/min. The morphologies of magnetic polymer microspheres were observed by a transmission electron microscopy (TEM, FEI Tecnai G20). The magnetization curves of the magnetic polymer microspheres were measured with a vibrating sample magnetometer (LAKESHORE-7304, USA) at room temperature. 2.7 Lipase immobilization The obtained Fe 3 O 4 /P(HEMA–DMDAAC) supports were used for immobilizing lipase. On one hand, this composite support has good adsorption effect for lipase because of the positive electrical charges on the surface of supports. On the other, the active epoxy groups in the activated magnetic polymer supports can react with the amino group of lipase in gentle conditions [24,25] . Therefore, the lipase immobilization was carried out by reaction of lipase solution with magnetic polymer supports directly. Firstly, 1 g of magnetic nanoparticles supports had been dipped in 50 ml of phosphate buffer (0.1 M, pH = 7.0) for 24 h. After separated, the supports were transferred into 50 ml of candida rugosa lipase solution (m/v 0.2%). Then the lipase immobilization was completed after stirred magnetically for 8 h. At last, the immobilized CRL was obtained by magnetic separation and washed with phosphate buffer (0.1 M, pH = 7.0) several times to remove the unreacted lipase. Especially, the reaction solution and washing solution were collected to assay the amount of residual enzyme. The resulting immobilized CRL was kept at 4 °C prior to use. During the immobilization procedure, the effect of pH value, reaction time and the amount of lipase added on the activity of immobilized lipase were investigated, and the relative activity was obtained after incubation under a variety of pH values (6.5–9.0), immobilizing time (2–10 h) and the amount of lipase added (37.5 mg/g support–137.5 mg/g support), respectively. 2.8 Determination of immobilization efficiency and lipase activity The immobilization efficiency was expressed by the amounts of enzyme bounded on supports of unite mass, and the amount of enzyme was determined by the Bradford method [26] , using BSA as the standard. The enzymatic activities of free and immobilized lipase were measured by the titration of the fatty acid which comes from the hydrolysis of olive oil [27] and reverse titration was adopted. One unit of lipase activity (U) is defined as the amount of enzyme needed to hydrolyze olive oil liberating 1.0 μmol fatty acid per minute in the assay condition. The efficiency of immobilization was evaluated in terms of activity yields and immobilization yield as follows: Activity yield ( % ) = C A × 100 Immobilization yield ( % ) = A − B A × 100 where A is the activity of lipase added in the initial immobilization solution, B is the total activity of the residual lipase in the immobilization and washing solution after the immobilization procedure, and C is the activity of the immobilized lipase, respectively. The relative activity (%) is the ratio between the activity of every sample and the maximum activity of the sample. All data used in these formulas are the average of triplicate of experiments. 2.9 Thermal stability and store stability of FCRL and ICRL Thermal stabilities of FCRL and ICRL were studied by measuring the residual activities of the enzymes after incubation in phosphate buffer (0.1 M, pH 7.0) at 50 °C for 210 min with continuous stirring. A sample was removed with 30 min time interval and assayed for enzymatic activity. Relative activity was calculated as mentioned above and plotted against time. To assay the store stability of FCRL and ICRL, the lipases had been placed at room temperature for 30 days and the residual activities of the lipases were measured with a time interval of 5 days. 2.10 Reusability of ICRL The reusability of the immobilized CRL was determined by hydrolysis of olive oil with the recovered immobilized CRL. Finally, the activities of the subsequent enzymatic reaction were compared with that of the first run (activity defined as 100%). 3 Results and discussion 3.1 Preparation and characterization of magnetic supports 3.1.1 The activation mechanism of magnetic nanoparticles The hydroxyl groups on the initial magnetic Fe 3 O 4 /P(HEMA–DMDAAC) nanoparticles are not able to react with lipase directly, so epoxy chloropropane was used to activate the supports, as shown in Scheme 2 . Thus, the supports with epoxy groups can bind with lipases easily under gentle conditions. After activation, the contents of epoxy group (Ep) in the carriers, which were measured with sodium thiosulfate method according a former literature [28] , reached 0.96 ± 0.08 mmol/g support. 3.1.2 FT-IR analysis As shown in Fig. 1 , the structures of Fe 3 O 4 nanoparticles, VTES modified Fe 3 O 4 nanoparticles and magnetic Fe 3 O 4 /P(HEMA–DMDAAC) carriers were characterized by FT-IR spectroscopy. According to Fig. 1 , the characteristic absorption peak of Fe-O bonds in Fe 3 O 4 could be found at 574 cm −1 clearly in the three spectra. In Fig. 1 (b), the adsorption peak appeared at 1629 cm −1 belonged to the stretching vibrations of υ C C, and the adsorption peaks presented at 1426 cm −1 and 1278 cm −1 should be ascribed to the bending vibration of υ CH 2 and υ Si–C, respectively. In addition, the symmetric and asymmetric stretching vibrations of υ Si–O should be at 1121 cm −1 , 1043 cm −1 , 983 cm −1 and 750 cm −1 in both of spectra b and spectra c. According to the above data, it could be indicated that the Fe 3 O 4 nanoparticles were modified by VTES successfully. Comparing with Fig. 1 (b), the intensity of adsorption peak at 3420 cm −1 increased obviously in spectra (c), because of the hydroxyl group from the HEMA. Additionally, in spectra (c), the stretching vibration of υ C O could be found at 1725 cm −1 , and the adsorption peaks presented at 1454 cm −1 and 1384 cm −1 should be attached to the bending vibration of υ –CH 2 – and υ –CH 3 . Thus, it could be confirmed that the HEMA enwrapped over the modified Fe 3 O 4 nanoparticles. However, the structure of DMDAAC is similar to that of HEMA, so most of adsorption peaks were overlapped reciprocally. Furthermore, the characteristic absorption peak of υ C–N from DMDAAC, which should be presented at 1100 cm −1 , were overlapped with the adsorption peak of υ Si–O. As a result, elementary analysis was adopted to investigate the amount of N in the complex support, showing that the amount of N was 0.54 mmol/g support. Consequently, the supports were prepared successfully. 3.1.3 TG analysis The polymeric extent of the synthesized magnetic microspheres was estimated by TG analysis, as shown in Fig. 2 . A weight loss of 1.63% was observed when the temperature up to 120 °C, which indicated that a trace amount of water was contained in the dried magnetic microspheres. When the temperature rose from 120 °C to 790 °C, another 39.91% weight loss occurred due to polymer decomposition under nitrogen atmosphere. Thus, polymeric extent of magnetic microspheres is 39.91%. 3.1.4 TEM analysis The morphologies of magnetic polymer nanoparticles were investigated by transmission electron micrographs, shown in Fig. 3 . According to Fig. 3 , it can be seen clearly that the particles are spherical and the dimension of the particles are of nanometer grade. As shown in Fig. 3 (a), the average diameter of Fe 3 O 4 nanoparticles can be obtained, which is about 10–20 nm. In addition, the dispersibility of Fe 3 O 4 nanoparticles is not ideal because of the interface effects of nanoparticles and the profusive hydroxyl on the surface of nanoparticles. However, Fig. 3 (b) shows clearly that diameter of magnetic polymer supports reached to 80–100 nm after polymerization. Simultaneously, the dispersibility of the magnetic polymer supports became better than that of Fe 3 O 4 nanoparticles. In additional, the polymer region and the magnetic nanoparticles could be distinguished clearly. 3.1.5 VSM analysis The magnetic properties of the magnetic polymer microspheres were analyzed by VSM at room temperature. According to Fig. 4 , the saturation magnetization of pure Fe 3 O 4 nanoparticles was found to be 72.3 emu/g Fe 3 O 4 , while the saturation magnetization of magnetic Fe 3 O 4 /poly(HEMA–DMDAAC) complex carriers was 38.9 emu/g carrier. Theses carriers can be separated from the reaction system rapidly and easily due to the large saturation magnetization. Additionally, there was no hysteresis in the magnetization with both remanence and coercivity, proving that these magnetic carriers are super paramagnetic. Thus, these magnetic supports could be responding to an applied magnetic field without any permanent magnetization and redispersed rapidly when the magnetic field disappeared [29] . 3.2 Optimum conditions of immobilizing lipase To optimize the activities of ICRL, various conditions were tried for the immobilization processes and the relative activities of ICRL were assayed. 3.2.1 Effect of pH value The relationship between incubation pH (6.5–9.0) and the relative activity of ICRL was investigated in phosphate solution at 30 °C for 8 h. As shown in Fig. 5 , when the pH value reached 8.0, the relative activity of ICRL was the highest. The relative activities of ICRL were all lower when the pH value was higher or lower than 8.0. And the similar results have been achieved in former literatures [8] . Protein denaturation is very prone to occur under conditions of excess acid or alkali, so it is necessary to select an appropriate pH value [17] . 3.2.2 Effect of reaction time Fig. 6 shows the effect of reaction time on relative activity of the immobilized lipase in phosphate solution (0.1 M, pH = 8.0) at 30 °C. As the plots changes shown in Fig. 6 , the relative activity of the immobilized lipase increased with prolonged reaction time at first, while the activity decreased when the reaction time was longer than 8 h. As a result, the optimum reaction time is 8 h. It is suggested that the overcrowding of lipase molecules on the surface of supports is the possible reason, as a result of which substrate diffusion limitations occur, and finally leading the decrease of relative activities of ICRL with the prolonged reaction time [8,17] . 3.2.3 Effect of lipase amount added Immobilization reaction was taken out in phosphate solution (0.1 M, pH = 8.0) at 30 °C for 8 h, as shown in Fig. 7 , the relationship between the enzyme amount added and the relative activity of the immobilized enzyme was obtained. According to the plots changes in Fig. 7 , the relative activities of ICRL increased obviously with the increasing amount of lipase until the relative activity of ICRL reached a maximum value, at which the amount of protein given was 100 mg/g support. After the lipase amount added was more than 2.0 ml, the relative activities of ICRL started to decrease. The same phenomenon has been reported in previous work [30] . For this phenomenon, two reasons were considered. On one hand, an intermolecular steric hindrance was formed with higher enzyme loading, on the other hand, the diffusion of the substrate and product was encumbered [8] . As a result, the optimum conditions of immobilizing lipase were obtained: reaction time was 8 h, enzyme amount added was 100 mg/g support, pH value of reaction system was 8.0. The ICRL was prepared under theses optimum conditions, shown in Scheme 2 . After determination, the loading amount of lipase was 68.3 ± 0.5 mg CRL/g support, and the activity retention of ICRL was 60.4% (±1.6%). Therefore, this immobilized lipase appears to be pretty promising. Several literatures have appeared describing the study of carriers with epoxy for lipase immobilization. For example, Bayramoglu et al. [31] prepared poly(GMA–HEMA–EGDMA) microspheres for immobilization of CRL, and the amount of available epoxy groups was determined to be 0.8 mmol/g microsphere, while the maximum lipase immobilization capacities was 16.1 mg/g. In our group, magnetic microspheres with epoxy group has been prepared for immobilization of CRL [32] as well, and the content of epoxy groups on the surface of the microspheres sample was determined to be 1.08 mmol/g microsphere, while the amount of lipase immobilization was 48.1 ± 0.6 mg/g support. In this paper, the amount of epoxy group is 0.96 ± 0.08 mmol/g support while the loading amount of lipase reached to 68.3 ± 0.5 mg CRL/g support. Thus, with the exception of covalent binding, the electrostatic adsorption of the resulted supports played an important part in the enhancement of lipase immobilization. Besides stable lipases, other enzymes also have been immobilized by epoxy activated carriers. For example, Xue et al. [33] immobilized penicillin G acylase onto epoxy-functionalized mesostructured carriers; Bai et al. [34] synthesized magnetic hydrophilic bead carriers containing epoxy groups for glucoamylase immobilization. For the mechanism of covalent binding between epoxy group of carriers and anime group of enzymes is same, the conjugation of two kinds of immobilization methods reported in this study could be extended to other enzymes as well. 3.3 Properties of immobilized lipase 3.3.1 The thermal stability of immobilized lipase As well known, free lipase is not stable in solution and its activity decreases quickly during the process of use. Fig. 8 gave the variation of relative activities of ICRL and FCRL used for hydrolysis of olive oil after incubating in phosphate buffer (0.1 M, pH 8.0) at 50 °C for different time interval. According to Fig. 8 , the relative activities of ICRL and FCRL show a similar trend, but it is obvious that the thermal stability of ICRL was better than that of FCRL. This result is similar with previous report [31,35] . After incubated for 180 min, the residual activity of ICRL was about 60% of the initial activity, while the activity of FCRL loss completely. This excellent performance of ICRL could be due to the formation of covalent bonds between enzyme and supports, which enhanced the enzyme rigidity and prevented the conformation transition of the enzyme at high temperature [36] . 3.3.2 The reusability of immobilized lipase The reusability of immobilized enzyme is a pretty important aspect in application, especially in industrial application. To study the reusability, the immobilized CRL was washed with phosphate buffer (0.1 M, pH 7.0) after one catalysis run and reintroduced into a fresh olive oil solution for another hydrolysis at 37 °C. The variation of the activity of ICRL after multiple-reuse was showed in Fig. 9 . According to Fig. 9 , it could be observed that the residual activity still remained about 75% of the initial activity after the 10th reuse. So the ICRL prepared has good stability and reusability. The decrease of activity was considered as the denaturation of protein and the leakage of protein from the supports during the process of use [32] . 3.3.3 The storage stability of immobilized lipase The storage stability of immobilized lipase is also an important factor in lipase application. To investigation the storage stability of ICRL, we measured the activity of ICRL after placed at room temperature with different time interval, and the result was shown in Fig. 10 . After placed at room temperature for 30 days, the relative activity of ICRL maintained above 75% of the initial activity, while that of FCRL was only left over 30% of the initial activity. Thus, the storage stability of lipase was enhanced significantly. 4 Conclusions In this study, the super paramagnetic immobilization supports were prepared by a polymerization of HEMA and DMDAAC, with an activation of the carriers by epoxy chloropropane. Thus, this carrier has positive charge and active epoxy simultaneously. Additionally, the resulting magnetic supports held diameters as small as 80–100 nm, and displayed super paramagnetic with high saturation magnetization (38.9 emu/g), and they can be separated easily and rapidly by a magnetic field. Furthermore, the immobilized lipase held high activity recovery (60.4% ± 1.6%) and expressed better stability and applicability than the free ones. Consequently, this magnetic polymer support is a kind of economical and efficient carriers not only for lipase immobilization but also for other enzymes immobilization. Acknowledgements The authors thank the financial supports from the National Natural Science Foundation of China (No. 21074049), the National Training Fund for Person with Ability of Basic Subjects established by the Natural Science Foundation of China (J0730425) and the Opening Foundation of State Key Laboratory of Applied Organic Chemistry (SKLAOC-2009-35). References [1] J. Hong D.M. Xu P.J. Gong H.W. Sun L. Dong S. Yao Covalent binding of α-chymotrypsin on the magnetic nanogels covered by amino groups J. Mol. Catal. B-Enzym. 45 2007 84 90 [2] P.D. Mari J.V. Sinisterra S.W. Tsai A.R. Alcantara Carica papaya lipase (CPL): an emerging and versatile biocatalyst Biotechnol. Adv. 24 2006 493 499 [3] Y. Omprakash I. Toyoko Covalent-bonded immobilization of lipase on poly(phenylene sulfide) dendrimers and their hydrolysis ability Biomacromolecules 6 2005 2809 2814 [4] K. Jaeger M. Reetz Microbial lipase form versatile tools for biotechnology Trends Biotechnol. 16 1998 396 403 [5] L. Gardossi D. Bianchi A.M. Klibanov Selective acylation of peptide catalyzed by lipases in organic solvents J. Am. Chem. Soc. 113 1991 6328 6329 [6] P.D. Marıa J.M.S. Montero J.V. Sinisterra A.R. Alcantara Understanding candida rugosa lipases: an overview Biotechnol. Adv. 24 2006 180 196 [7] S.W. Chang J.F. Shaw K.H. Yang S.F. Chang C.J. Shieh Studies of optimum conditions for covalent immobilization of candida rugosa lipase on poly(c-glutamic acid) by RSM Bioresour. Technol. 99 2008 2800 2805 [8] D.S. Jiang S.Y. Long J. Huang H.Y. Xiao J.Y. Zhou Immobilization of Pycnoporus sanguineus laccase on magnetic chitosan microspheres Biochem. Eng. J. 25 2005 15 23 [9] L. Lei Y.X. Bai Y.F. Li L.X. Yi Y. Yang C.G. Xia Study on immobilization of lipase onto magnetic microspheres with epoxy groups J. Magn. Magn. Mater. 321 2009 252 258 [10] G. Cristian P. Lucia D.F. Vincenzo S. Paoto Gold nanoparticles-based protease assay Proc. Natl. Acad. Sci. U.S.A. 103 2006 3978 3982 [11] J. Hong P.J. Gong J.H. Yu D.M. Xu H.W. Sun S. Yao Conjugation of α-chymotrypsin on a polymeric hydrophilic nanolayer covering magnetic nanoparticles J. Mol. Catal. B-Enzym. 42 2006 99 105 [12] R. Torres C. Mateo L.G. Fernandez C. Ortiz M. Fuentes J.M. Palomo J.M. Guisan L.R. Fernandez A novel heterofunctional epoxy-amino sepabeads for a new enzyme immobilization protocol: immobilization-stabilization of beta-galactosidase from Aspergillus oryzae Biotechnol. Prog. 19 2003 1056 1060 [13] C. Mateo R. Torres L.G. Fernandez C. Ortiz M. Fuentes A. Hidalgo G.F. Lopez O. Abian J.M. Palomo L. Betancor B.C.C. Pessela J.M. Guisan L.R. Fernandez Epoxy-amino groups: a new tool for improved immobilization of proteins by the epoxy method Biomacromolecules 4 2003 772 777 [14] Y. Ding Y. Hu L.Y. Zhang Y. Chen X.Q. Jiang Synthesis and magnetic properties of biocompatible hybrid hollow spheres Biomacromolecules 7 2006 1766 1772 [15] Z. Guo S. Bai Y. Sun Preparation and characterization of immobilized lipase on magnetic hydrophobic microspheres Enzyme Microb. Technol. 32 2003 776 782 [16] B. Gulay T. Yagmur M.Y. Arica Immobilization of b-galactosidase onto magnetic poly(GMA–MMA) beads for hydrolysis of lactose in bed reactor Catal. Commun. 8 2007 1094 1101 [17] G. Bayramoglu S. Kiralp M. Yilmaz L. Toppare M.Y. Arıca Covalent immobilization of chloroperoxidase onto magnetic beads: Catalytic properties and stability Biochem. Eng. J. 38 2008 180 188 [18] Y. Yang Y.X. Bai Y.F. Li L. Lei Y.J. Cui C.G. Xia Preparation and application of polymer-grafted magnetic nanoparticles for lipase immobilization J. Magn. Magn. Mater. 320 2008 2350 2355 [19] Y.J. Wang F. Caruso Mesoporous silica spheres as supports for enzyme immobilization and encapsulation Chem. Mater. 17 2005 953 961 [20] S. Koutsopoulos J. Oost W. Norde Adsorption of an endoglucanase from the hyperthermophilic Pyrococcus furiosus on hydrophobic (polystyrene) and hydrophilic (silica) surfaces increases protein heat stability Langmuir 20 2004 6401 6406 [21] B.C.C. Pessela C. Mateo A.V. Carrascosa A. Vian J.L. Garcia G. Rivas C. Alfonso J.M. Guisan R.F. Lafuente One-step purification, covalent immobilization, and additional stabilization of a thermophilic poly-His-tagged beta-galactosidase from Thermus sp. strain T2 by using novel heterofunctional chelate-epoxy Sepabeads Biomacromolecules 4 2003 107 113 [22] R. Bohuslav S. Alena H. Daniel Functionalised magnetic microspheres with hydrophilic properties for molecular diagnostic applications Food Res. Int. 42 2009 493 498 [23] R. Massart V. Cabuil Effect of some parameters on the formation of colloidal magnetite in alkaline-medium-yield and particle-size control J. Chim. Phys. Phys.-Chim. Biol. 84 1987 967 973 [24] F.J. Xu Q.J. Cai Y.L. Li E.T. Kang K.G. Neoh Covalent immobilization of glucose oxidase on well-defined poly(glycidyl methacrylate)-Si(111) hybrids from surface-initiated atom-transfer radical polymerization Biomacromolecules 6 2005 1012 1020 [25] A.B. Teke S.H. Baysal Immobilization of urease using glycidyl methacrylate grafted nylon-6-membranes Process Biochem. 42 2007 439 443 [26] M.M. Bradford A rapid and sensitive method for the quantitation of microgram quantities of protein utilizing the principle of protein–dye binding Anal. Biochem. 72 1976 248 254 [27] N. Watanabe Y. Ota Y. Minoda K. Yamada Isolation and identification of alkaline lipase producing microorganisms: cultural conditions and some properties of crude enzymes Agric. Biol. Chem. 41 1977 1353 1358 [28] L. Sundberg J. Porath Preparation of adsorbents for biospecific affinity chromatography J. Chromatogr. 90 1974 87 98 [29] Z.Y. Ma Y.P. Guan H.Z. Liu Superparamagnetic silica nanoparticles with immobilized metal affinity ligands for protein adsorption J. Magn. Magn. Mater. 301 2006 469 477 [30] Y.X. Bai Y.F. Li Y. Yang L.X. Yi Covalent immobilization of triacrylglycerol lipase onto functionalized nanoscale SiO 2 spheres Process Biochem. 41 2006 770 777 [31] G. Bayramoglu B. Kaya M.Y. Arıca Immobilization of candida rugosa lipase onto spacer-arm attached poly(GMA–HEMA–EGDMA) microspheres Food Chem. 92 2005 261 268 [32] Y. Yang Y.X. Bai Y.F. Li L. Lei Y.J. Cui C.G. Xia Characterization of candida rugosa lipase immobilized onto magnetic microspheres with hydrophilicity Process Biochem. 43 2008 1179 1185 [33] P. Xue F. Xu L.D. Xu Epoxy-functionalized mesostructured cellular foams as effective support for covalent immobilization of penicillin G acylase Appl. Surf. Sci. 255 2008 1625 1630 [34] Y.X. Bai Y.F. Li M.T. Wang Study on synthesis of a hydrophilic bead carrier containing epoxy groups and its properties for glucoamylase immobilization Enzyme Microb. Technol. 39 2006 540 547 [35] A. Chaubey R. Parshad S. Koul Arthrobacter sp. lipase immobilization for improvement in stability and enantio selectivity Appl. Microbiol. Biotechnol. 73 2006 598 606 [36] F.N. Xi J.M. Wu Z.S. Jia X.F. Lin Preparation and characterization of trypsin immobilized on silica gel supported macroporous chitosan bead Process Biochem. 40 2005 2833 2840
MoreTranslated text
Key words
Super paramagnetism,Electrostatic adsorption,Covalent binding,Immobilized CRL,Enzyme technology,Reusability
AI Read Science
Must-Reading Tree
Example
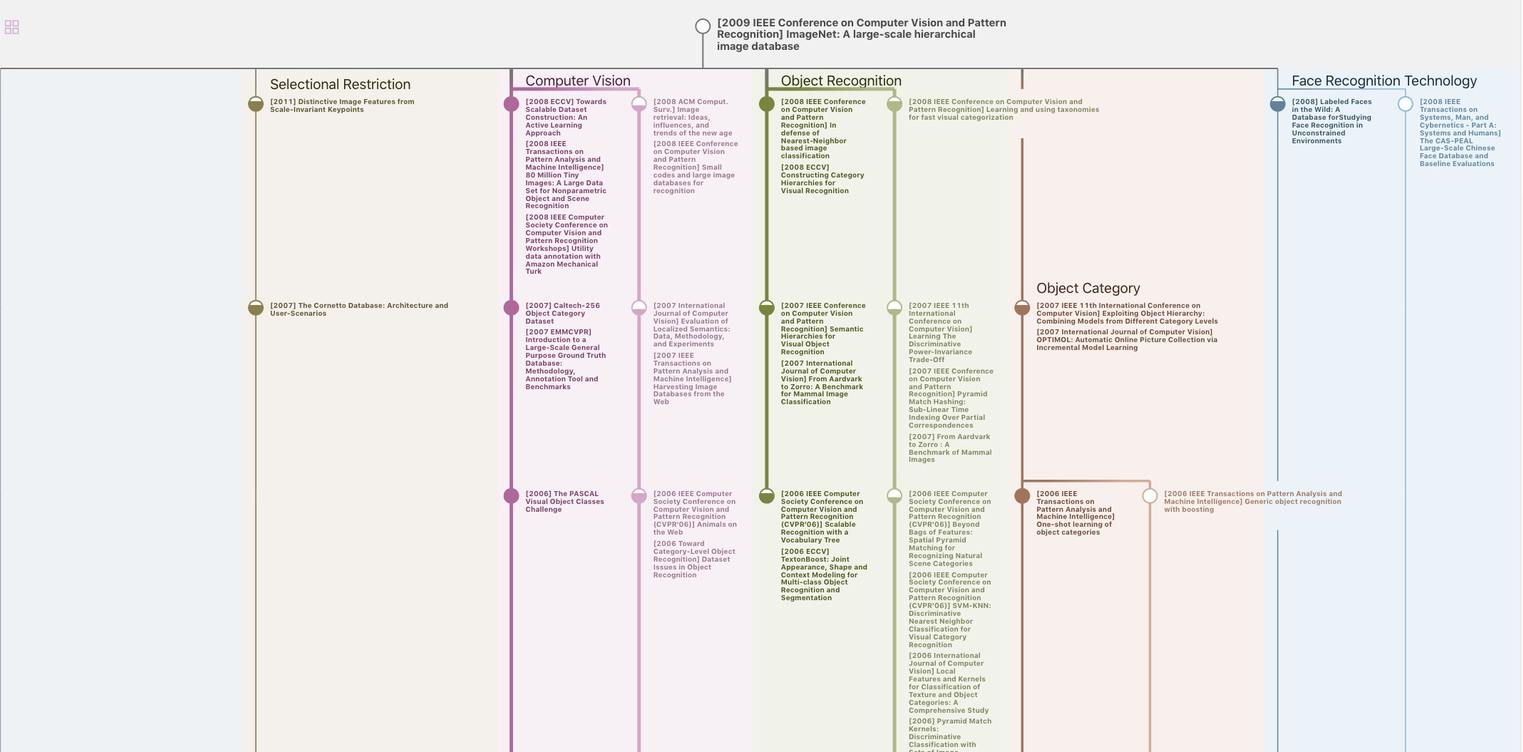
Generate MRT to find the research sequence of this paper
Chat Paper
Summary is being generated by the instructions you defined