Characterization of the novel intermetallic compounds U2Co3Ge, U6Co12Ge4 and U6Co12Ge4C
Journal of Alloys and Compounds(2011)
Abstract
The new ternary compounds U 2 Co 3 Ge and U 6 Co 12 Ge 4 have been discovered during the investigation of the U–Co–Ge system. The Laves phase, U 2 Co 3 Ge crystallizes in the hexagonal Mg 2 Cu 3 Si type structure ( P 6 3 / mmc ) with the lattice parameters a = 5.203(1) Å and c = 7.687(1) Å. U 6 Co 12 Ge 4 and its carbon-inserted derivative U 6 Co 12 Ge 4 C, crystallize, at room temperature in cubic unit cells, which derive from the Ca 3 Ag 8 type structure ( I m 3 ¯ m space group) with the lattice parameter a = 8.690(1) Å and a = 8.740(1) Å, respectively. An interesting feature of their crystal structure is the formation of [U6] octahedra, which can be either empty or occupied by light elements such as O, N, or C. Measurements of the physical properties revealed that U 2 Co 3 Ge and U 6 Co 12 Ge 4 are Pauli paramagnets with metallic character of their electrical conductivity. In turn, U 6 Co 12 Ge 4 C showed Curie–Weiss paramagnetism with an effective magnetic moment of 2.3(1) μ B per U atom. Keywords Actinide alloys and compounds Intermetallics Heavy fermions X-ray diffraction Magnetic measurements Electrical transport 1 Introduction Intermetallic binary phases containing uranium and an iron-triad metal (T = Fe, Co, Ni) show various magnetic behaviors ranging from weak paramagnetism to hard ferromagnetism. Some of them exhibit interesting electrical transport properties, such as low-temperature superconductivity discovered in the U 6 T binary compounds [1] . In turn, binary uranium germanides form an interesting series of compounds with unique properties, as exemplified by the coexistence of ferromagnetism and superconductivity under moderate pressure in UGe 2 [2] . The occurrence of unconventional superconductivity has also been observed in the family of ternary germanides, U–T–Ge, (T = Fe, Co, Ni) with the best example being the ferromagnetic heavy-fermion superconductor UCoGe [3] . In the course of our systematic investigations of the influence of d -electrons on the physical properties of the U–T–Ge phases [4,5] , including also the identification of new compounds, a detailed study on the U–Co–Ge system has been undertaken [6] . Up to now, eight ternary intermetallic phases have been reported in the literature, namely UCoGe [7] , UCo 2 Ge 2 [8] , UCo 6 Ge 6 [9] , U 3 Co 2 Ge 7 [10] , U 3 Co 4 Ge 7 [11] , U 2 Co 17− y Ge y (1.3 ≤ y ≤ 3) [12] , and the newly characterized intermediate compounds, U 3 Co 12− x Ge 4 (0 ≤ x ≤ 2) [13] and UCo 3− x Ge x (0.2 ≤ x ≤ 0.4) [14] . Recently, we have identified two novel ternaries U 2 Co 3 Ge and U 6 Co 12− x Ge 4 , which adopt a ternary ordered variant of the MgZn 2 Laves phase [15] (Mg 2 Cu 3 Si-type structure [16] ) and a ternary derivative of the Ca 3 Ag 8 structure [17] (Ce 3 Ni 6 Si 2 -type [18] ), respectively. In the present paper we describe the crystallographic structures of both compounds, as well as that of the carbon-inserted derivative of U 6 Co 12 Ge 4 compound, i.e., U 6 Co 12 Ge 4 C. Moreover, we report on the magnetic and electrical properties of these phases. 2 Experimental details Starting materials for the syntheses were uranium turnings, cobalt, germanium and carbon pieces (all purities above 99.9%). Calculated amounts of the components were melted in an arc-furnace under residual argon atmosphere. In order to ensure good homogeneity, the buttons were turned over and remelted. To promote homogenization and crystallization, various heat-treatments were performed. Samples were annealed at 973 K for 3 weeks in sealed silica tubes, followed by quenching down to room temperature or heat-treated in a high frequency furnace under residual atmosphere of argon, up to 1523 K, followed by slow cooling (0.5 K/min) down to 1083 K, temperature at which the power was turned off. All samples were examined by means of powder X-ray diffraction (XRD) and scanning electron microscopy coupled with energy-dispersive spectroscopy (SEM-EDS). A piece of each sample was embedded in epoxy resin and polished using SiC paper and diamond paste down to 1 μm. The compositional contrast among the various phases was revealed by means of a backscattered electron detector. The semi-quantitative analyses for elements with an atomic number greater than eleven were corrected through the use of external standards of stoichiometric compounds such as UGe 2 , UCo and U 3 Co 2 Ge 7 . The precision of these measurements is considered to be about 1 at.% for U, Co and Ge. The crystal structure of U 2 Co 3 Ge was investigated by powder XRD using a Bruker AXS D8 Advance ( θ –2 θ Bragg–Brentano geometry) diffractometer working with monochromatized Cu Kα 1 radiation ( λ = 1.5406 Å) and equipped with a LynxEye detector. The experiments were performed within the 10–135° 2θ data range with 2θ steps of 0.008° and counting time of 1.4 s per step, each step being measured 180 times. The diffraction data were analyzed using the Rietveld profile refinement method [19] implemented in the FULLPROF program [20] . Small single crystals of U 6 Co 12 Ge 4 and U 6 Co 12 Ge 4 C, suitable for crystal structure determination, were picked up from the heat-treated samples. The diffraction intensities were collected at room temperature on a Nonius Kappa CCD four circle diffractometer working with Mo Kα radiation ( λ = 0.71073 Å). The integration and reduction of redundant reflections of the different data sets as well as the cell refinements were performed using EvalCCD [21] . Semi-empirical absorption corrections were made with the use of the Multiscan software [22] . Structural models were determined by direct methods using SIR-97 [23] . All the structure refinements and Fourier syntheses were made with the help of SHELXL-97 [24] . The atomic positions have been standardized using STRUCTURE TIDY [25] . DC magnetic measurements were carried out using a Quantum Design MPMS-5 SQUID magnetometer. The data were collected in the 2–350 K temperature range with applied magnetic fields up to 5 T. The electrical resistivity was measured over the 4.2–300 K temperature interval employing a standard DC four-point technique. 3 Results 3.1 Phase formation The existence of the new compounds U 6 Co 12 Ge 4 and U 2 Co 3 Ge was revealed in the course of the systematic investigation of the U–Co–Ge ternary phase diagram. SEM observations coupled with EDS analysis of annealed samples with initial compositions in the vicinity of U 3 Co 12 Ge 4 and UCo 2.6 Ge 0.4 revealed secondary products having elemental compositions U:Co:Ge of 28(1):54(1):18(1) and 34(1):49(1):17(1). The corresponding powder XRD patterns showed the presence of diffraction peaks that could not be indexed with the structure-types of known U–Co binary or U–Co–Ge ternary phases. As a result of several other attempts, it was found that U 6 Co 12 Ge 4 could be described assuming the Ca 3 Ag 8 -type unit cell (space group I m 3 ¯ m ) and the lattice parameter a = 8.69(1) Å), whereas U 2 Co 3 Ge could be indexed with the MgZn 2 -type structure (space group P 6 3 / mmc ) with the lattice parameters a = 5.20(1) Å, c = 7.69(1) Å. It should be emphasized that the two novel compounds were hardly present in the arc-melted ingots with nominal compositions of U 2 Co 3 Ge and U 6 Co 12 Ge 4 but appeared as majority phases upon long-term annealing these buttons at 973 K. Methodical chemical analyses of several samples prepared in order to establish the phase equilibria did not reveal any homogeneity domain in neither of the two materials, hence suggesting that they are well-defined compounds. 3.2 Crystal structure refinements and descriptions 3.2.1 U 6 Co 12 Ge 4 and U 6 Co 12 Ge 4 C Single crystal of U 6 Co 12 Ge 4 (labelled “A” in the following) was selected from a melted button with the nominal composition U:Co:Ge of 25:62:13, annealed in a high frequency furnace, as described in the previous chapter. The Bragg reflections were indexed with a body-centred cubic unit cell with the lattice parameter at room temperature, a = 8.736(2) Å. The crystal structure was solved by direct methods in the space group I m 3 ¯ m (no. 229) with one U, one Co and one Ge atoms located on the 12 e , 24 h and 8 c Wyckoff positions, respectively. Difference Fourier maps revealed substantial electron densities of about 19.2 electrons per Å 3 on the 2 a (0, 0, 0) position, which corresponds to a vacancy inside the octahedron formed by the uranium atoms. This positive peak in the Fourier map, correlated with the uranium atom–void distance of 2.438(1) Å, features the presence of light elements such as C, N or O, which are commonly observed as contaminants. In this context it is worth noting that the lattice parameter a determined for the U 6 Co 12 Ge 4 sample annealed at 1573 K was significantly larger than that revealed for the specimen heat-treated in silica tube at 973 K ( a = 8.74(1) Å versus a = 8.69(1) Å). The observed enlargement of the unit cell can be rationalized by solubilisation of light element in the structure during crystal growth process at elevated temperatures. Subsequent refinements with the appropriate light elements selectively included on the 2 a site yielded the occupancy of 0.70 for oxygen, 0.94 for nitrogen and 1.17 for carbon atoms, while the final Fourier maps were featureless in each case. These results and the comparison with the typical interatomic distances, d (U–O) = 2.367 Å in UO 2 , d (U–N) = 2.440 Å in UN and d (U–C) = 2.475 Å in UC [26] , points to a probable insertion of nitrogen atoms inside the uranium octahedra. However, based on the crystallographic investigation only, the exact nature of the inserted element cannot be properly ascribed, as it has already been noticed for U 3 Ni 6 Ge 2 X [27] . To characterize more precisely the role of contaminants on the phase formation and the crystallographic relations in U 6 Co 12 Ge 4 , additional syntheses were carried out with intentionally added carbon in various amounts. Another single crystal (labelled “B” in the following) was extracted from a sample with the nominal composition U:Co:Ge:C of 25:50:17:8 that was annealed in a silica tube at 973 K for three weeks. Refinement of the collected X-ray data indicated a cubic structure with the lattice parameter a = 8.740(1) Å, in which the non-equivalent atoms, U, Co, Ge and C are located at the 12 e , 24 h , 8 c and 2 a Wyckoff positions, respectively. All the crystallographic sites were found fully occupied and the final difference Fourier map was featureless. For the quaternary compound, its right chemical formula, which requires integer numbers for elements is U 6 Co 12 Ge 4 C. Hence, to keep consistency in the relations between the host structure and the carbon inserted derivative, the chemical formula of the ternary compound appears better described as U 6 Co 12 Ge 4 formula ( Z = 2) instead of the commonly reported formula for compounds adopting the Ca 3 Ag 8 type ( Z = 4) [17] . The powder XRD pattern of the U:Co:Ge:C of 25:50:17:8 sample revealed a single phase character of this material. The refined lattice parameter a = 8.724(1) Å is close to that obtained for the single crystal “B”. In contrast, the XRD pattern of a quaternary sample with the nominal composition U:Co:Ge:C of 27:54:18:1 (i.e. substoichiomety in elemental carbon) presents clear splitting of all the diffraction peaks which is the signature of two cubic phases with the space goup I m 3 ¯ m , suggesting the presence of a U 6 Co 12 Ge 4 C carbon-rich phase ( a = 8.729(1) Å) and C free U 6 Co 12 Ge 4 phase ( a = 8.689(1)) ( Fig. 1 ). The performed experiments yielded the following conclusions: (i) the ternary compound U 6 Co 12 Ge 4 with the lattice parameter a = 8.690(1) Å coexists at the same thermodynamic conditions with quaternary phases U–Co–Ge–X (X = O, N, C) having the unit cell parameter about a = 8.736(1) Å, (ii) insertion of C atoms in the [U6] octahedral cages provokes an enlargement of the unit-cell volume of about 1.5% as it was already observed in the case of U 6 Fe 16 Si 7 and U 6 Fe 16 Si 7 C compounds [28] and (iii) the addition of X atom seems to ensure a better crystallization of the samples, but is not necessary to stabilize the ternary phase, as already noticed in ref [28] . The relevant crystallographic data and some details on the refinements performed for the single crystals “A” and “B”, are gathered in Table 1 . The atomic coordinates and the selected interatomic distances in U 6 Co 12 Ge 4 C are listed in Table 2 and Table 3 , respectively. Fig. 2 displays a perspective view of the crystal structure of U 6 Co 12 Ge 4 C, which can be described as being built up from regular [U6] octahedra containing one carbon atom and surrounded by [Ge8] cubes and [Co12] cuboctahedra. The [U6] octahedra lay on nodes of the body-centred cubic lattice and are separated by the U–U distances of 3.865(1) Å, which are slightly larger than the U–U distances of 3.447(1) Å within the [U6] motifs. Remarkably, the distance between the U and Co atoms, d (U–Co) = 2.704(1) Å, is shorter than the sum of the metallic radii of the corresponding elements ( r U + r Co = 2.812 Å) [29] . This short distance manifests strong hybridization between the 5 f and 3 d wave-functions, as observed for example in the binary phase UCo, where d (U–Co) = 2.645 Å [30] . 3.2.2 U 2 Co 3 Ge Due to the lack of single crystals of sufficient quality, the structural investigation of U 2 Co 3 Ge was performed on powder XRD data. Most of the observed diffraction peaks were indexed in the hexagonal space group P 6 3 / mmc (no. 194) with the lattice parameters a = 5.203(1) Å and c = 7.687(1) Å. This finding pointed to a possible crystal structure of the Mg 2 Cu 3 Si type [16] , that was previously established for the similar Fe-containing compound U 2 Fe 3 Ge [31] . After fitting of the background line (using selected points), profile of the diffraction peaks and lattice parameters, Rietveld refinement started by introducing the structural model of U 2 Fe 3 Ge. Noticeable diffraction peaks of U 6 Co 12 Ge 4 were observed, the latter compound was taken into account as a secondary phase. The micro-absorption correction parameters were refined to get physically reasonable values of the atomic displacement parameters [32] . The relevant experimental data are presented in Table 4 together with the main results of the refinements. Fig. 3 depicts a comparison between the observed and calculated powder patterns and difference curve of the Rietveld refinement. The positional parameters are listed in Table 5 , while a selection of the interatomic distances is given in Table 6 . The three non-equivalent atoms, one U, one Co and one Ge are located on 4 f , 6 h , 2 c Wyckoff positions of the P 6 3 / mmc space group, respectively. The shortest U–U interatomic distance of 2.728(1) Å is much smaller than the sum of the metallic radii of the element ( r U + r U = 3.12 Å [29] ). Similarly short U–U distances have been observed in the binaries UCo ( d (U–U) = 2.645 Å) and U 6 Co ( d (U–U) = 2.693 Å) [30] , as well as in the isostructural compound U 2 Fe 3 Ge [31] . The crystal structure of U 2 Co 3 Ge is shown in Fig. 4 . It can be described as a stacking of two kinds of layers along the c -axis. The non-planar U–Ge layer is composed of a network of the U atoms forming “chair”-like configuration of the [U 6 ] hexagons centred by a Ge-atom. The second layer is composed of the Co atoms forming a planar Kagome network. 4 Magnetic and electrical properties The temperature dependencies of the molar magnetic susceptibility χ (T) of U 6 Co 12 Ge 4 , U 6 Co 12 Ge 4 C and U 2 Co 3 Ge are presented in Fig. 5 (a) . The two ternary compounds show Pauli paramagnetic behavior with their temperature independent magnetic susceptibility of about 2 × 10 −3 emu mol U −1 . Similar magnitude of the susceptibility was reported for the binaries UCo [33] and UCo 1.981 [34] and the ternaries UCo 2.6 Ge 0.4 [14] and U 3 Co 12− x Ge 4 [13] , which also behave as Pauli paramagnets. Remarkably, in all these phases the ratio of the number of Co atoms over the total number of atoms is between 1/2 and 2/3. At room temperature, the molar magnetic susceptibility of U 6 Co 12 Ge 4 C is about 6.8 × 10 −3 emu mol U −1 , i.e. roughly four times that measured for U 6 Co 12 Ge 4 . With decreasing temperature the magnetic susceptibility of this compound considerably increases and can be described by the modified Curie–Weiss law χ ( T ) = χ 0 + 1 8 µ eff 2 T − θ P where μ eff is the effective magnetic moment, θ P is the paramagnetic Curie–Weiss temperature, and χ 0 is a sum of the temperature-independent contributions. The least squares fit of this equation to the experimental data yielded the values: χ 0 = 0.0051(1) emu mol U −1 , μ eff = 2.3(1) μ B per U atom, and θ P = −73(5) K. Similarly reduced effective paramagnetic moments (in respect to the free U 3+ or U 4+ ion values) were observed for numerous U-based compounds, such as U 3 Co 2 Ge 7 [10] , U 3 Co 4 Ge 7 [11] , U 6 Fe 16 Si 7 C [28] , U 1.2 Fe 4 Si 9 [35] or U 3 Pt 23 Si 11 [36] . In all these systems the U-5 f electronic states are believed to have an intermediate character between localized and delocalized models. Apparently, unlike the purely itinerant behavior of the 5 f electrons in U 6 Co 12 Ge 4 , some degree of localization is observed in U 6 Co 12 Ge 4 C, i.e. upon insertion of carbon atoms into the octahedral voids of the cubic unit cell. The magnetization isotherms of U 6 Co 12 Ge 4 , U 6 Co 12 Ge 4 C and U 2 Co 3 Ge, measured at 2 K, are linear and fully reversible when measured with increasing and decreasing magnetic field (see Fig. 5 (b)). Such a behavior of М(H) is entirely in line with the paramagnetic character of the studied compounds. The temperature variations of the electrical resistivity of U 6 Co 12 Ge 4 and U 2 Co 3 Ge are shown in Fig. 6 . Both materials exhibit a metallic character of the electronic transport. The bending over the ρ (T) dependencies likely signals some contribution to the carriers scattering due to spin fluctuations [37,38] , as previously reported for example for U 3 Co 12− x Ge 4 [13] . 5 Conclusions Two novel ternary compounds U 6 Co 12 Ge 4 and U 2 Co 3 Ge have been discovered and their crystal structures determined. The Laves phases U 2 Co 3 Ge crystallizes in the hexagonal Mg 2 Cu 3 Si structure type (space group P 6 3 / mmc) , which is an ordered variant of the MgZn 2 type. In turn, U 6 Co 12 Ge 4 adopts the cubic Ce 3 Ni 6 Si 2 unit cell (space group I m 3 ¯ m ) which is a ternary ordered variant of the Ca 3 Ag 8 type. The crystal structure of the latter compound comprises [U6] octahedra, which can be accommodated by light elements such as C, N, or O. The intentional insertion of carbon atoms yields an enlargement of the unit cell with the lattice parameter changing from a = 8.689 Å in U 6 Co 12 Ge 4 to a = 8.740 Å in U 6 Co 12 Ge 4 C. Remarkably, phase equilibrium between both the ternary and quaternary compounds occurs at the same thermodynamic conditions. Magnetic measurements revealed that U 2 Co 3 Ge and U 6 Co 12 Ge 4 are Pauli paramagnets. Both compounds exhibit metallic conductivity with significant contribution due to spin fluctuations. Interestingly, insertion of carbon into the unit cell of U 6 Co 12 Ge 4 promotes some localization of the 5f electrons, which manifests itself as modified Curie–Weiss character of the magnetic susceptibility of U 6 Co 12 Ge 4 C. Acknowledgement This work was partially supported by the French–Polish Integrated Activity Program “POLONIUM” no. 20080 PM (2009–2010). References [1] B.S. Chandrasckhar J.K. Hulm J. Phys. Chem. Solids 7 1958 259 [2] S.S. Saxena P. Agarwal K. Ahilan F.M. Grosche R.K.W. Haselwimmer M.J. Steiner E. Pugh I.R. Walker S.R. Julian P. Monthoux G.G. Lonzarich A. Huxley I. Sheikin D. Braithwaite J. Flouquet Nature 406 2000 587 592 [3] N.T. Huy A. Gasparini D.E. de Nijs Y. Huang J.C.P. Gortenmulder A. De Visser A. Hamann T. Görlach H.v. Löhneysen Phys. Rev. Lett. 99 2007 067006 [4] A. Perricone, Ph.D. Thesis, Université Rennes 1, Rennes, 2002. [5] D. Berthebaud, Ph.D. Thesis, Université Rennes 1, Rennes, 2007. [6] A. Soudé, Ph.D. Thesis, Université Rennes 1, Rennes, 2010. [7] R. Troć V.H. Tran J. Magn. Magn. Mater. 73 1988 389 [8] T. Endstra G.J. Nieuwenhuys A.A. Menovsky J.A. Mydosh J. Appl. Phys. 69 1994 4816 [9] W. Buchholz H.-U. Schuster Z. Anorg. Allg. Chem. 482 1981 40 [10] S. Bobev E.D. Bauer F. Ronning J.D. Thompson J.L. Sarrao J. Solid State Chem. 180 2007 2830 [11] R. Pöttgen B. Chevalier P. Gravereau B. Darriet W. Jeitschko J. Etourneau J. Solid State Chem. 115 1995 247 [12] B. Chevalier P. Gravereau T. Berlureau L. Fournès J. Etourneau J. Alloys Compd. 233 1996 174 [13] A. Soudé O. Tougait M. Pasturel D. Kaczorowski H. Noël J. Solid State Chem. 183 2010 1180 [14] A. Soudé, O. Tougait, M. Pasturel, D. Kaczorowski, H. Noël, Solid State Phenom., in press. [15] K.H. Leiser H. Witte Z. Metallkd. 43 1952 396 [16] H. Witte Z. Angew. Miner. 1 1938 255 [17] L.D. Calvert C. Rand Acta Crystallogr. 17 1964 1175 [18] E.I. Gladyshevskii P.I. Krypyakevych O.I. Bodak Z. Anorg. Allg. Chem. 344 1966 95 [19] H.M. Rietveld J. Appl. Crystallogr. 2 1969 65 [20] J. Rodriguez-Carvajal Physica B 192 1993 55 [21] A.J.M. Duisenberg, Ph.D. Thesis, Universiteit Utrecht, 1998. [22] R.H. Blessing Acta Crystallogr. Sect. A 51 1995 33 [23] A. Altomare M.C. Burla M. Camalli G.L. Cascarano C. Giacovazzo A. Guagliardi A.G.G. Moliterni G. Polidori R.J. Spagna J. Appl. Crystallogr. 32 1999 115 [24] G.M. Sheldrick Acta Crystallogr. Sect. A 64 2008 112 [25] E. Parthé K. Cenzual R. Gladyshevskii J. Alloys Compd. 197 1993 291 [26] R.E. Rundle N.C. Baenziger A.S. Wilson R.A. McDonald J. Am. Chem. Soc. 70 1948 99 [27] A. Perricone H. Noël J. Alloys Compd. 383 2004 251 [28] D. Berthebaud O. Tougait M. Potel E.B. Lopes A.P. Gonçalves H. Noël J. Solid State Chem. 180 2007 2926 [29] E. Teatum, K. Gschneidner, J. Waber, Compilation of calculated data useful in predicting metallurgical behaviour of the elements in binary alloy systems, 1960, Los Alamos Scientific Laboratory, LA-2345. [30] N.C. Beanziger R.E. Rundle A.I. Snow A.S. Wilson Acta Crystallogr. 3 1950 34 [31] M.S. Henriques O. Tougait H. Noël L.C.J. Pereira J.C. Waerenborgh A.P. Gonçalves Solid State Commun. 148 2008 159 [32] W. Pitschke N. Mattern H. Hermann Powder Diffract. 8 1993 223 [33] J. Turán A. Zentko J. Šternberk J. Hřebík Acta Phys. Slov. 31 1981 143 [34] A. Zentko J. Hřebík J. Šternberk J. Turán Physica B 102 1980 269 [35] S. Noguchi K. Okuda T. Adachi Y. Haga E. Yamamoto Y. Onuki J. Phys. Soc. Jpn. 66 1997 2572 [36] J.N. Chotard O. Tougait H. Noël P. Rogl A. Zelinsky O.I. Bodak J. Alloys Compd. 407 2006 36 [37] R. Troć Z. Bukowski C. Sułkowski H. Misiorek J.A. Morkowski A. Szajek G. Chełkowska Phys. Rev. B 70 2004 184443 [38] T. Nishioka K. Kimura H. Matsui M. Kontani J. Phys. Soc. Jpn. 63 1994 2722
MoreTranslated text
Key words
Actinide alloys and compounds,Intermetallics,Heavy fermions,X-ray diffraction,Magnetic measurements,Electrical transport
AI Read Science
Must-Reading Tree
Example
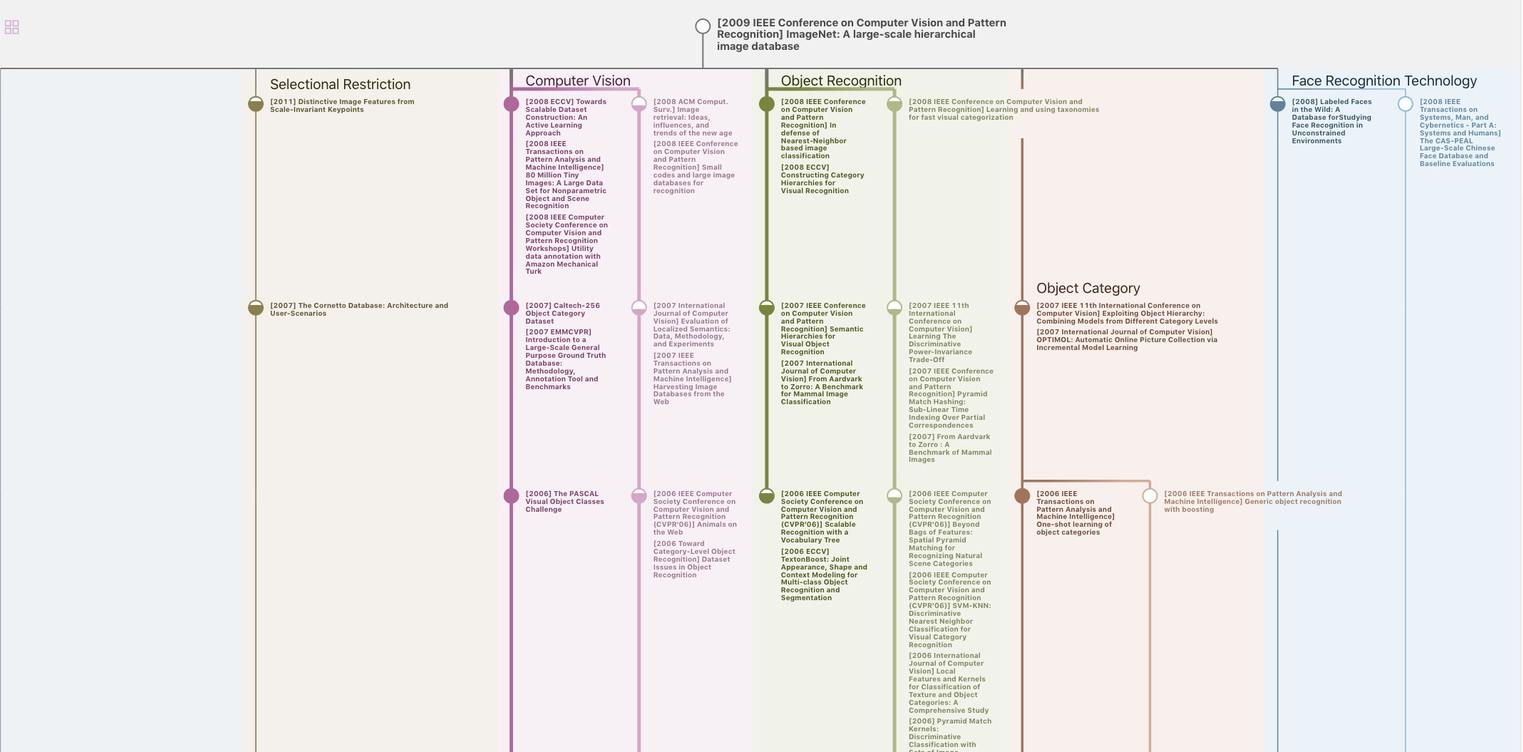
Generate MRT to find the research sequence of this paper
Chat Paper
Summary is being generated by the instructions you defined