Electrical conductivity of textured Sm3+ and Nd3+ Co-doped CeO2 thin-film electrolyte
Electrochimica Acta(2011)
Abstract
Research highlights ► Sm 3+ and Nd 3+ co-doped ceria thin-film electrolytes are deposited by RF magnetron sputtering. ► With increase in the substrate temperature from room temperature to 600 °C, the structure of the film varies from (1 1 1) preferred orientation to random orientation. ► The (1 1 1) textured film has lower electric conductivity in comparison with the random oriented film. ► The SNDC film deposited at 600 °C shows the maximum conductivity of 0.006 S cm −1 at 500 °C. Abstract Nanocrystalline thin-film electrolytes of Sm 3+ and Nd 3+ co-doped ceria have been deposited on polycrystalline alumina substrates via RF magnetron sputtering. It is found that with the increase of substrate temperature from room temperature to 600 °C, the structure of the film varies from (1 1 1) preferred orientation to random orientation, accompanied by an enhancement in electrical conductivity. It is estimated that the activation energy for oxygen ion migration along (1 1 1) orientation may be higher than other crystal orientations, resulting in a lower conductivity of the (1 1 1) barrier textured co-doped ceria film electrolyte. It also indicates that the electrical conduction is predominantly due to the oxygen ions. Keywords Solid oxide fuel cells Co-doped ceria RF magnetron sputtering Thin-film electrolyte Preferred orientation 1 Introduction Solid oxide fuel cells (SOFCs) have attracted a considerable attention due to their high energy conversion efficiency and fuel flexibility [1,2] . As solid electrolyte ceria-based oxide with lower activation energy for oxygen diffusion has been greatly investigated to the alternative of yttria-stabilized zirconia for medium/low-temperature applications in SOFCs, however it shows a mixed ionic–electronic conducting behavior either at high temperature or in a reducing atmosphere [3,4] . Some researchers indicated that an effective solution to overcome this issue was to use co- or multi-doping [4–7] , which is due to the decreased association enthalpy between oxygen vacancies and doping cations [7] . On the other hand, considerable efforts have been focused on thin-film electrolytes with lower ohmic losses for SOFCs, driven by the urgent need to compensate for the performance degradation encountered in lowering the operating temperature [8,9] . Compared with the corresponding bulk materials, thin films can be fabricated at a lower sintering temperature or even without sintering at all. Moreover, the conductivities of thin films can be effectively modulated by controlling nanocrystalline microstructure. The use of thin films as solid electrolytes is also a great driving force for developing micro-SOFCs with volume and stability superiority, which supplies a great application potential for miniature mobile energy storage devices [8,10] . Many researchers have been attempting to reduce the thickness of the electrolyte via a variety of thin-film techniques. Texture can be easily formed in thin films using these techniques as reported in CeO 2 /ZrO 2 films deposited by EB-PVD [11] , magnetron sputtering [12] , and e-beam evaporation [13] , for which effects of substrate category, deposition temperature, atmosphere pressure and power on the texture formation have been discussed. Magnetron sputtering, due to its significant superiorities such as the general high deposition rate, high quality of the deposit, and scalability to large substrates, has been widely used for the fabrication of oxide films [14,15] . It is conceivable that such a texture in the film may have an influence on the transfer of the conduction ions, since the crystal plane having relatively large conduction channel may facilitate the ionic conduction. Up to now, there are many publications about the textured CeO 2 thin films, but few have dealt with the relationship between the texture and the electrical conductivity. In the present report, Sm 3+ and Nd 3+ co-doped ceria (SNDC) of composition Ce 0.8 Sm 0.1 Nd 0.1 O 1.9 thin-film electrolytes were prepared by RF magnetron sputtering, and the texture in the film was tuned by substrate temperature. Further, detailed investigation of texture effect on the electrical conductivity of the SNDC film has been carried out. 2 Experimental The target for RF magnetron sputtering was obtained by hot-pressed sintering from SNDC powders synthesized through citric-nitrate combustion [4] . The SNDC films with ∼7 μm thickness were then deposited on polycrystalline alumina substrates (10 mm × 10 mm × 1 mm), under a sputtering pressure of 2.0 Pa and RF power of 300 W, in argon (20 sccm) and oxygen (5 sccm) mixture atmosphere. The substrate temperature was controlled as room temperature (RT), 400, 500 and 600 °C. The samples were then post-annealed in air at 800 °C for 2 h. For the electrical conductivity measurements, Pt electrodes in stripes were formed by DC sputtering on the SNDC film surface. X-ray diffraction (XRD) (D/MAX-RB X-ray diffractometer, Rigaku, Akishima-Shi, Japan) was carried out on the SNDC thin film electrolyte for phase identification. The surface morphologies and thicknesses were examined using scanning electron microscopy (SEM) (JSM-6460LV, JEOL, Tokyo, Japan). The element valence state was determined by X-ray photoelectron spectroscopy (XPS) (PHI Quantera SXM, ULVAC-PHI, Japan). Electrical conductivities of the samples were measured in air and N 2 by the two-point probe ac impedance spectroscopy (IM6, Zahner, Kronach, Germany) at the frequency range from 0.10 Hz to 8 MHz, and the temperature range was from 300 to 700 °C. 3 Results and discussion As shown in Fig. 1 (a)–(c) , to have a better microscopic insight of the texture-dependent of conductivity, the homogeneous surface morphologies of SNDC films were investigated by SEM, from which it can be seen that there is hardly any difference in the morphological features among the films deposited at various temperatures. According to Scherrer formula, the grain size of SNDC films against the substrate temperature is shown in Fig. 1 (d), indicating the grain size remains almost unchanged no matter at what substrate temperature in our study. Fig. 2 (a) displays the X-ray diffraction patterns for the CeO 2 bulk and SNDC films deposited at various substrate temperatures. All the peaks of SNDC films exhibit the single phase CeO 2 with a cubic fluorite structure without any other traces, demonstrating that the CeO 2 is fully stabilized by Sm 2 O 3 and Nd 2 O 3 . As shown in Fig. 2 (b), the pole figure of the (1 1 1) plane in the SNDC film deposited at RT indicates that the (1 1 1) orientation in the film is extended parallel to the substrate surface. As for the effect of co-doping of Sm and Nd on the CeO 2 lattice changes, detailed analysis has been done in our previous report [4] . In order to study the texture in SNDC films, orientation factors of the films grown at different substrate temperatures were calculated. The definition of orientation factor can be expressed as follows: (1) f = I ( 1 1 1 ) I ( 1 1 1 ) + I ( 2 2 0 ) + I ( 3 1 1 ) where I (1 1 1) , I (2 2 0) , and I (3 1 1) indicate the (1 1 1), (2 2 0) and (3 1 1) peak intensity, respectively. And (1 1 1), (2 2 0) and (3 1 1) peaks are the three strongest peaks of the cubic fluorite CeO 2 . When f equals to 1, the crystal of SNDC in the film is completely (1 1 1) orientated. The decrease in f value implies the intensity of random orientation increases. The XRD results show that the SNDC film deposited at RT has a (1 1 1) highly preferred orientation with an orientation factor being close to 0.9, and the intensity of (1 1 1) orientation decreases with the increase of substrate temperature. The orientation factor of the film deposited at 600 °C approaches 0.53, indicating a random orientation exists in the film. Similar results were reported on the CeO 2 film grown on Si substrate [16] . It is noticed that the preferred orientation of the SNDC film shows a strong dependence on substrate temperature, which is because the substrate temperature significantly affects the surface diffusion coefficient of deposited atoms. The diffusion coefficient can be expressed as follows: (2) D = D 0 exp − Δ E k T where T indicates the absolute temperature, k the Boltzmann constant, Δ E the surface diffusion activation energy, and D 0 is the diffusion constant [17] . When the substrate temperature is relatively low, the deposition rate of crystal plane is determined by the surface diffusion activation energy. As is well known, CeO 2 has a face-centered cubic fluorite-type structure. The most close-packed (1 1 1) plane is that of lowest surface energy and would be preferred thermodynamically in the film growth process, resulting in the highest deposition rate and a texture of the (1 1 1) plane. As the substrate temperature rises, the increased energy of deposited atoms could contribute to a higher possibility of diffusion and collision, indicating the bombarding atoms would arrive at the substrate at random angles. Additionally, the thin film tends to follow the crystal orientation of the substrate when the deposition rate decreases at high substrate temperature [18,19] . Therefore, the SNDC film deposited at a high temperature shows a random oriented behavior. The temperature dependence of the electrical conductivity in the form of an Arrhenius plot is depicted in Fig. 3 , derived from impedance spectra. The inset in Fig. 3 (a) shows that there is only one semicircular arc observed at high and intermediate frequencies corresponding to the contribution from grain resistance to the overall conductivity [12] . That means the resistances at the grain boundary are very small, and the grain boundary performs the high conduction route for the oxygen ions. Additionally, the activation energy of the SNDC film (0.780 ± 0.031 eV for the film deposited at 600 °C) which is closer to that of grain (0.850 ± 0.018 eV) than that of grain boundary (1.17 ± 0.039 eV) of polycrystalline, resulting in the dominated grain conduction in our study. It is also observed that the maximum value of electrical conductivity reaches to 0.006 S cm −1 at 500 °C for the SNDC film deposited at 600 °C. As shown in Fig. 2 (a), there is (1 1 1) preferred orientation in the film deposited on cold substrate even after annealing in air at 800 °C. Corresponding to the electrical conductivity measurement results shown in Fig. 3 , the conductivity of the SNDC film increases with the reduction in the intensity of the (1 1 1) preferred orientation. The conductivity at selected temperatures against the orientation factor derived from formula (1) was plotted in Fig. 4 . It is clear that the conductivity of the SNDC film decreases when the preferred orientation is increased. As the in-plane type electrode geometry we adopted for testing the conductivity, the current flow in the SNDC film is parallel to the substrate surface during measurement, hence the direction also parallels the (1 1 1) planes for the highly orientated grains in SNDC films. Fig. 1 (d) demonstrates that the influence of the grain size on electrical conductivity can be neglected by reason of the unchanged grain size of the films deposited at different temperatures. Moreover, the conduction in SNDC films is influenced by lattice-controlled diffusivity because of the micro-scale film thickness [20] . Since the crystal of doped ceria is typical cubic fluorite structure (1 1 1), plane has the highest atom density, which supplies a higher migration barrier for oxygen ions to cross over, resulting in a higher activation energy and lower electrical conductivity for the (1 1 1) texture film. In contrast, the film deposited at 600 °C with the most random orientation of the crystal structure shows the highest electrical conductivity in the situation of present research. It is reasonable that the orientation of the lower atomic density plane will be the fast oxygen ion transfer direction with lower migration barrier for oxygen ion hopping, but at present stage, it is difficult to prepare and measure the lower atomic density plane orientated thin films. As the movement of the ion is constrained by the crystal lattice and the mobility of ion is much lower than that of electron, the strong texture dependent conductivity also suggests the mechanism of electrical conductivity in SNDC films might be mainly dominated by oxygen ions. However, more evidence have been made to prove the total electrical conductivities measured by AC impedance spectroscopy are truly ionic conductivities. The possibility of SNDC films undergoing any electronic conduction due to the reduction of Ce 4+ to Ce 3+ was monitored by XPS, as shown in Fig. 5 (a) . The spectral features in the energy range of 880–900 eV and 900–920 eV correspond to the Ce 3d 5/2 signal and the Ce 3d 3/2 signal, respectively [21,22] . Comparing with the published results [22,23] , all the peaks show the presence of Ce 4+ only in the samples, indicating that there is not Ce of the trivalence state and electronic conduction existing in the SNDC films. Furthermore, if the reduction of Ce 4+ to Ce 3+ occurs, the ceria-based electrolytes will have a mixed ionic–electronic conduction behavior in reducing atmosphere, showing a higher total conductivity. Choi et al. [24] reported that the oxygen partial pressure-independent electrical conductivity of the sputtered Gd 2 O 3 doped CeO 2 thin film indicated that the electrical conduction originated mainly from oxygen ions, not electrons. As shown in Fig. 5 (b), compared with the conductivity measured in N 2 , the total conductivity in air of the SNDC film was not changed by variation in pO 2 , suggesting that the electrical conduction of the film originates mainly from oxygen ions. Therefore, the electrical conductivity of SNDC films is predominantly due to the ionic conductivity. 4 Conclusions In summary, Sm 3+ and Nd 3+ co-doped ceria thin-film electrolytes were prepared on polycrystalline alumina substrate by RF magnetron sputtering. Texture of the SNDC film could be controlled by selected substrate temperature, demonstrating that the film with random orientation showed a higher conductivity than the (1 1 1) orientated film. It was confirmed that the electrical conductivity was mainly due to the ionic conductivity. It was also observed that the maximum electrical conductivity value was as high as 0.006 S cm −1 at 500 °C for the film deposited at 600 °C. Acknowledgment This research was supported by TOYOTA MOTOR Corp . References [1] B.C.H. Steele A. Heinzel Nature 414 2001 345 [2] J.W. Fergus J. Power Source 162 2006 30 [3] B. Zhu J. Power Sources 114 2003 1 [4] Y.Y. Liu B. Li X. Wei W. Pan J. Am. Ceram. Soc. 91 2008 3926 [5] D.A. Andersson S.I. Simak N.V. Skorodumova I.A. Abrikosov B. Johansson PNAS 103 2006 3518 [6] S. Omar E.D. Wachsman J.C. Nino Appl. Phys. Lett. 91 2007 144106-1 [7] W. Zając J. Molenda Solid State Ionics 179 2008 154 [8] D. Beckel A. Bieberle-Ḧutter A. Harvey A. Infortuna U.P. Muecke M. Prestat J.L.M. Rupp L.J. Gauckler J. Power Sources 173 2007 325 [9] A.M. El-Toni T. Yamaguchi S. Shimizu Y. Fujishiro M. Awano J. Am. Ceram. Soc. 91 2008 346 [10] A. Evans A. Bieberle-Hütter H. Galinski J.L.M. Rupp T. Ryll B. Scherrer R. Tolke L.J. Gauckler Monatsh. Chem. 140 2009 975 [11] P. Scardi M. Leoni F. Cernuschi A. Figari J. Am. Ceram. Soc. 84 2001 827 [12] G. Chiodellia L. Malavasi V. Massarotti P. Mustarelli E. Quartarone Solid State Ionics 176 2005 1505 [13] A. Karthikeyan C.L. Chang S. Ramanathan Appl. Phys. Lett. 89 2006 183116-1 [14] S. Kalpat K. Uchino J. Appl. Phys. 90 2001 2703 [15] L.Y. Chen W.H. Chen J.J. Wang F.C.N. Hong Appl. Phys. Lett. 85 2004 5628 [16] W.C. Tsi T.Y. Tseng J. Mater. Sci.: Mater. Electron. 8 1997 313 [17] Z.E. Liu Fundamentals of Materials Science 2003 Northwestern Polytechnical University Press Xi’an [18] S. Gnanarajan N. Savvides Thin Solid Films 350 1999 124 [19] L. Kim J. Kim D. Jung C.Y. Park C.W. Yang Y. Roh Thin Solid Films 360 2000 154 [20] I. Kosacki C.M. Rouleau P.F. Becher J. Bentley D.H. Lowndes Electrochem. Solid-State Lett. 7 2004 A459 [21] J.L.M. Rupp T. Drobek A. Rossi L.J. Gauckler Chem. Mater. 19 2007 1134 [22] S. Banerjee P. Sujatha Devi D. Topwal S. Mandal K. Menon Adv. Funct. Mater. 17 2007 2847 [23] H.J. Ko J.J. Lee S.H. Hyun Electrochem. Solid-State Lett. 13 2010 B113 [24] S.H. Choi C.S. Hwang H.W. Lee J. Kim J. Electrochem. Soc. 156 2009 B381
MoreTranslated text
Key words
Solid oxide fuel cells,Co-doped ceria,RF magnetron sputtering,Thin-film electrolyte,Preferred orientation
AI Read Science
Must-Reading Tree
Example
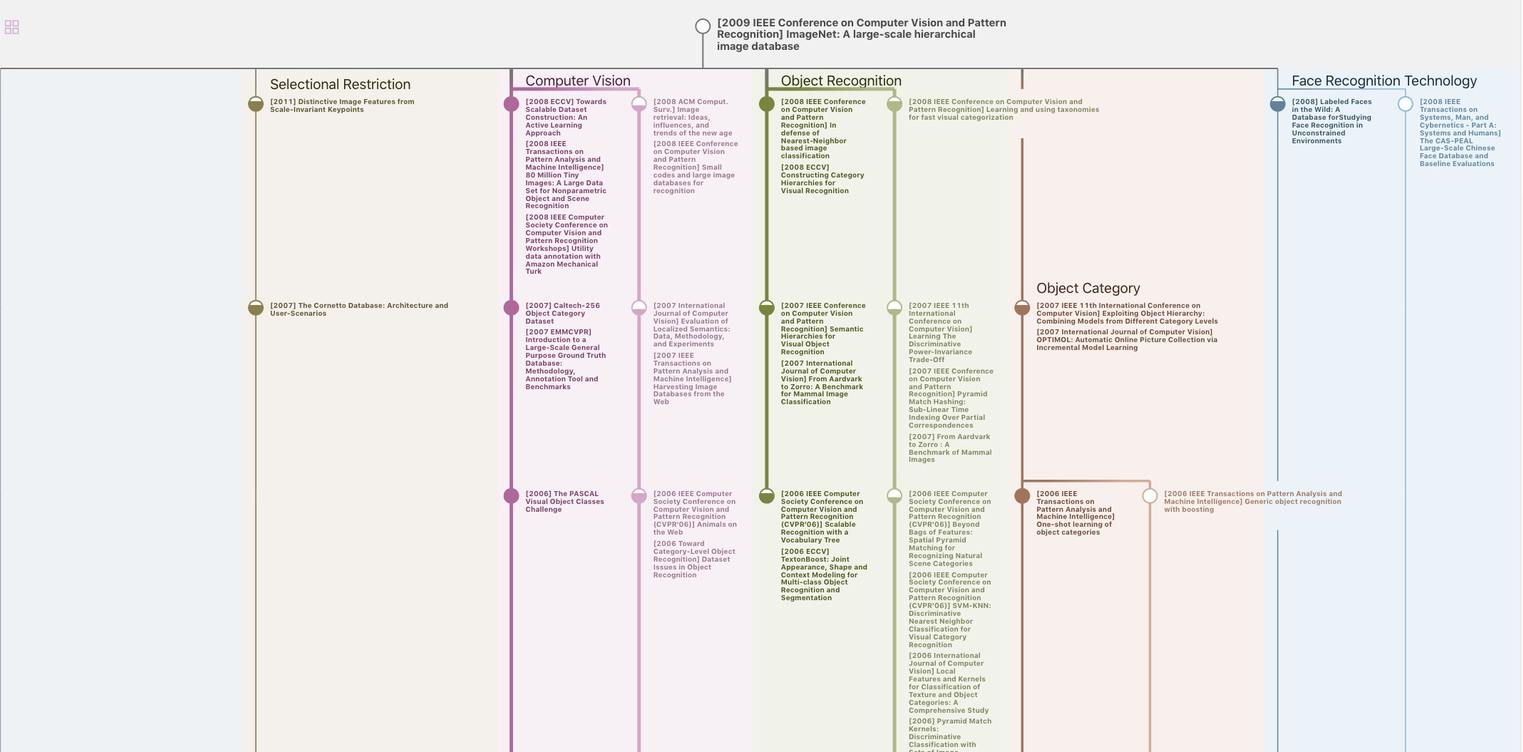
Generate MRT to find the research sequence of this paper
Chat Paper
Summary is being generated by the instructions you defined