Structural characterization and photocatalytic properties of novel Bi2YVO8
Materials Research Bulletin(2008)
摘要
Bi 2 YVO 8 was prepared by solid-state reaction for the first time. The structural and photocatalytic properties of Bi 2 YVO 8 were studied. The results showed that this compound has the tetragonal crystal system with space group I 4/ mmm . The band gap of Bi 2 YVO 8 was estimated to be about 2.09 eV by plotting ( αhν ) 2 versus hν and obtaining the hν axis intercept value according to Tauc's equation. For the photocatalytic water splitting reaction, H 2 or O 2 evolution was observed from pure water with Bi 2 YVO 8 as the photocatalyst under ultraviolet light irradiation (wavelength = 390 nm). Degradation of aqueous methylene blue photocatalyzed by this compound was investigated under visible light irradiation. Bi 2 YVO 8 showed higher photocatalytic activity compared to Bi 2 YTaO 7 , Bi 2 InTaO 7 or TiO 2 (P-25). Complete removal of aqueous methylene blue was achieved after visible light irradiation for 170 min. The decrease of the total organic carbon and the formation of inorganic products such as SO 4 2− and NO 3 − revealed the continuous mineralization of aqueous methylene blue during photocatalytic reaction. Keywords A. Inorganic compounds A. Oxides C. X-ray diffraction D. Catalytic properties D. Optical properties 1 Introduction Since water splitting catalyzed by TiO 2 was discovered in 1972 [1] , photocatalysis has attracted extensive attention from both academic and industrial organizations [2–13] . In particular, water splitting by the photocatalytic approach has been considered as a promising process to acquire clean and renewable H 2 source [4,14–15] . In addition, plentiful researches about the photocatalytic degradation of aqueous organic pollutants have been reported [2,16–21] . Methylene blue (MB) dye is usually used as a probe pollutant to assess the activity of a photocatalyst owing to its high stability against degradation in the natural environment [22–24] . However, there are only a few studies about MB dye degradation under visible light irradiation [22,25] . It is noteworthy that a slight modification of a semiconductor structure will cause a distinct change in photocatalytic properties [16] . We consider that Y 3+ and V 5+ occupying the B site, at the same time Bi 3+ and Bi 5+ occupying the A site in the A 2 B 2 O 8 compound may cause a slight modification of crystal structure, which may result in a change in photophysical properties. However, the space group data, the lattice constants and the photocatalytic properties of Bi 3+ Bi 5+ Y 3+ V 5+ O 8 have not been investigated so far. In this contribution, the structural and photocatalytic properties of Bi 2 YVO 8 were investigated. The photocatalytic properties of Bi 2 YVO 8 were compared with those of Bi 2 InTaO 7 , Bi 2 YTaO 7 and TiO 2 (P-25) in order to elucidate its structure-photocatalytic activity relationship. 2 Experimental The polycrystalline samples of the photocatalysts were synthesized by a solid-state reaction method. Y 2 O 3 , V 2 O 5 , In 2 O 3, Ta 2 O 5 , Bi 2 O 3 and TiO 2 with purity of 99.99% (Sinopharm Group Chemical Reagent Co., Ltd., Shanghai, China) were used as raw materials. Prior to catalyst preparation, all chemicals were dried at 200 °C for 4 h. For the preparation of Bi 2 YVO 8 , the precursors were stoichiometrically mixed, pressed into small columns and charged into an alumina crucible (Shenyang Crucible Co., Ltd., China). Then a calcination was carried out at 920 °C for 50 h in an electric furnace (KSL 1700X, Hefei Kejing Materials Technology Co., Ltd., China). Similarly, Bi 2 YTaO 7 and Bi 2 InTaO 7 were prepared by calcination at 1080 °C for 52 h and at 1050 °C for 46 h, respectively. For H 2 evolution reaction, loaded cocatalysts such as Pt, NiO and RuO 2 were prepared. Pt was loaded on the catalyst surface by an in situ photodeposition method using aqueous standard solution of H 2 PtCl 6 (Shanghai Chemical Reagent Research Institute, China) [26] . Under light irradiation, an equivalent molar amount of H 2 PtCl 6 in solution was reduced to the metallic state and deposited onto the surface of the catalyst, forming Bi 2 YVO 8 loaded by Pt. Loaded cocatalysts such as NiO and RuO 2 were prepared by the impregnation method using standard Ni(NO 3 ) 2 or RuCl 3 solution (Sinopharm Group Chemical Reagent Co., Ltd.), separately [27] . The precursors were dried at 325 K and heated at 778 K for 2 h in air. The crystal structure of Bi 2 YVO 8 was analyzed by the powder X-ray diffraction method (D/MAX-RB, Rigaku Corporation, Japan) with Cu Kα radiation ( λ = 1.54056). The data were collected at 295 K with a step scan procedure by the range of 2 θ from 10 to 100°. The step interval was 0.02° and the time per step was 1.2 s. The chemical composition of the compound was determined by scanning electron microscope-X-ray energy dispersion spectrum (SEM-EDS, LEO 1530VP, LEO Corporation, Germany) and X-ray fluorescence spectrometer (XFS, ARL-9800, ARL Corporation, Switzerland). The oxygen content, Bi 3+ content and Bi 5+ content of Bi 2 YVO 8 was determined by X-ray photoelectron spectroscopy (XPS, ESCALABMK-2, VG Scientific Ltd., U.K.). The chemical composition in the depth profile of Bi 2 YVO 8 was determined by the argon ion denudation method when X-ray photoelectron spectroscopy was used. The optical absorption of Bi 2 YVO 8 was analyzed with an UV–Vis spectrophotometer (Lambda 35, Perkin-Ebmer Corporation, USA). The surface areas of the samples were measured with the BET method (MS-21, Quantachrome Instruments Corporation, USA) by N 2 adsorption at liquid nitrogen temperature. The photocatalytic degradation of aqueous MB (Tianjin Kermel Chemical Reagent Co., Ltd.) was performed within a pyrex glass cell (Jiangsu Yancheng Huaou Industry, China) equipped with a 300 W Xe arc lamp (Nanjing JYZCPST CO., Ltd.) and a cut-off filter ( λ > 420 nm, Jiangsu Nantong JSOL Corporation, China). The incident photon flux I o was determined to be 4.51 × 10 −6 Einstein L −1 s −1 under visible light irradiation (wavelength range of 420–700 nm). The structural formula of MB is shown in Fig. 1 . The photocatalyst concentration was 3 g L −1 and the concentration of MB was 0.0506 mmol L −1 (mM) with the initial pH value 7.0. The concentration of MB was monitored by an UV–Vis spectrometer (Lambda 35, Perkin-Ebmer Corporation, USA) with the detecting wavelength at 670 nm. The inorganic products obtained from MB degradation were analyzed by ion chromatograph (DX-300, Dionex Corporation, USA). Total organic carbon (TOC) was determined with a TOC analyzer (TOC-5000, Shimadzu Corporation, Japan). In order to elucidate the influence of dissolved O 2 on MB photodegradation, a control experiment was fulfilled. The anoxic reaction conditions were achieved by purging MB solution with N 2 and the concentration of dissolved O 2 was monitored by Logging Do Meter (HI2400, Hanna Instruments, Romania). The photonic efficiency was calculated according to Eq. (1) [28,29] : (1) φ = R I o where φ is the photonic efficiency (%), R is the rate of MB degradation (Mol L −1 s −1 ), and I o is the incident photon flux (Einstein L −1 s −1 ). The photocatalytic water splitting was conducted under ultraviolet light irradiation in a closed gas circulation system with an inner irradiation type reactor (quartz cell). A light source (400 W high-pressure Hg lamp, Beijing Dongsheng Glass Light Source Factory, China) showing the incident photon flux I o of 6.01 × 10 −6 Einstein L −1 s −1 was focused through a shutter window and a 390 nm cut-off filter was placed onto the window face of the cell. The evolved gases were determined with a TCD gas chromatogragh (6890N, Agilent Technologies, USA), which was connected to the closed gas circulation system. For photocatalytic H 2 or O 2 evolution reaction from pure water, 1 g catalyst was suspended in 300 mL H 2 O by using stirrer. Contrastively, H 2 evolution reaction was also carried out in CH 3 OH/H 2 O solution (50 mL CH 3 OH, 300 mL H 2 O) over Bi 2 YVO 8 loaded by Pt, NiO or RuO 2 cocatalyst. Before reaction, the closed gas circulation system and the reaction cell were degassed until O 2 and N 2 can not be detected. Then 35 Torr of Argon was charged into the system. 3 Results and discussion XRD patterns of Bi 2 YVO 8 , Bi 2 YTaO 7 [29] and Bi 2 InTaO 7 are shown in Fig. 2 . It is clear that Bi 2 YTaO 7 and Bi 2 InTaO 7 crystallize with the pyrochlore-type structure and have cubic system with space group Fd3m. SEM-EDS spectrum of Bi 2 YVO 8 is illustrated in Fig. 3 . The average particle size of Bi 2 YVO 8 was determined to be 1.3 micron. The chemical composition of the compound was determined by characteristic X-rays of BiM, BiL, VK, YL and OK with the ZAF (element number, absorption and fluorescence corrections) quantification method. The SEM-EDS analysis revealed that Bi 2 YVO 8 had a homogenous atomic distribution without other detectable impurities besides a little aluminum coming from alumina crucible. Figs. 4–6 show the XPS spectrum of oxygen, Bi 3+ and Bi 5+ coming from the Bi 2 YVO 8 photocatalyst. An average atomic ratio of Bi:Y:V:O = 2.00:0.98:1.03:7.95 for Bi 2 YVO 8 was obtained based on XPS analysis, SEM-EDS analysis and XFS analysis. In addition, XRD results also showed that Bi 2 YVO 8 was a single phase, which was consistent with SEM-EDS results. According to XPS analysis ( Figs. 5 and 6 ), the oxidation state of Bi is mixture of +3 and +5, and an average atomic ratio of Bi 3+ :Bi 5+ = 0.53:0.47 for Bi 2 YVO 8 was obtained. The oxidation state of Y, V, and O ion is +3, +5 and −2, respectively. Based on above analysis, it could be concluded that the chemical formula of the novel compound was Bi 3+ 1.06 Bi 5+ 0.94 Y 3+ 0.98 V 5+ 1.03 O 2− 7.95 (predigested as Bi 2 YVO 8 ). The symmetry property of Bi 2 YVO 8 was that Bi 2 YVO 8 belonged to the tetragonal crystal system and the space group of Bi 2 YVO 8 was I 4/ mmm . The lattice parameters of Bi 2 YVO 8 were found to be a = 3.9188(2) Å, b = 3.9188(2) Å, c = 15.3105(9) Å. All diffraction ( h k l ) peaks of Bi 2 YVO 8 were shown in Fig. 2 . Greenblatt and coworkers [30–35] studied Y 2 FeMoO 7 , Sr 3 Fe 1.25 Mo 0.75 O 6.9 , La 3 Ni 2 O 6.35 , Sr 3 FeMoO 6.88 , Ca 3− x Th x Mn 2 O 7 ( x = 0–0.2), Eu 2 VO 4 and also observed the space group of I 4/ mmm . Fig. 7 shows the results of diffuse reflection spectra of Bi 2 YVO 8 , Bi 2 YTaO 7 [29] and Bi 2 InTaO 7 . In contrast to TiO 2 with absorption edge at about 400 nm, Bi 2 YVO 8 shows obvious absorption in visible light region up to 593 nm, indicating that Bi 2 YVO 8 is capable of responding to visible light irradiation. For a crystalline semiconductor, the optical absorption near the band edge follows the Eq. (2) [36] : (2) α h ν = A ( h ν − E g ) n where A , α , E g , ν and n are proportional constant, absorption coefficient, band gap, light frequency and parameter characteristic of the transition in a semiconductor, respectively. E g and n can be calculated by the following steps: plot ln( αhν ) versus ln( hν − E g ) using an approximate value of E g , then decide the value of n according to the slope of the straight line near the band edge. In the end, plot ( αhν ) 1/ n versus hν and evaluate the band gap E g by extrapolating the straight line to the hν axis intercept. Based on this method, the value n of Bi 2 YVO 8 was calculated to be 0.51 from Fig. 6 , indicating that the optical transition in this oxide is directly allowed. Fig. 8 shows the Plot of ( αhν ) 2 versus hν for Bi 2 YVO 8 . The band gap of Bi 2 YVO 8 was found to be 2.09 eV, indicating narrower band gap compared to that of Bi 2 InTaO 7 (2.85 eV). According to Fig. 6 the band gaps of Bi 2 YTaO 7 and Bi 2 InTaO 7 were estimated to be 2.23 and 2.85 eV, respectively. This implys that the photoabsorption of Bi 2 YVO 8 is stronger than that of Bi 2 InTaO 7 , which may result in a higher photocatalytic activity of Bi 2 YVO 8 than that of Bi 2 InTaO 7 . Fig. 9 shows the photocatalytic H 2 evolution from pure water (300 mL H 2 O, 1.0 g catalyst) under UV light irradiation with Bi 2 YVO 8 as the photocatalyst. H 2 evolution rate was estimated to be 40.7 μmol h −1 with a photonic efficiency of 0.628%. In addition, H 2 evolution could be terminated by switching off the UV light, indicating that H 2 evolution is induced by UV light irradiation. Fig. 9 also shows the photocatalytic O 2 evolution from pure water under UV light irradiation with Bi 2 YVO 8 as the photocatalyst. Similar to H 2 evolution, the amount of evolved O 2 increased with illumination time and the O 2 evolution rate was estimated to be 20.7 μmol h −1 . For Bi 2 YVO 8 , the turnover number – the ratio of total amount of gas evolved to catalyst – exceeded 1.0113, after 24 h of reaction time under ultraviolet light irradiation. The turnover number was enough to prove that the reaction occurred catalytically. In order to compare the catalytic activities of Bi 2 YVO 8 with that of TiO 2 , water splitting with TiO 2 (P-25) as the catalyst was conducted. By means of the TiO 2 (P-25) photocatalyst, the rate of H 2 evolution from pure water was about 1.4 μmol h −1 in the first 15 h, indicating a much lower activity than that of Bi 2 YVO 8 . Based on the observed results about H 2 and O 2 evolution from pure water, it can be concluded that the lowest conduction band level of Bi 2 YVO 8 is more negative than that of H 2 evolution and the highest valence band level is more positive than that of O 2 evolution. Namely, Bi 2 YVO 8 has proper band structure for the reduction of H + to H 2 and oxidation of H 2 O to O 2 , respectively. The proposed band structure of Bi 2 YVO 8 is described in Fig. 10 . The band structure of Bi 2 YVO 8 is similar to InMO 4 (M = V, Nb and Ta) and BiVO 4 . Recently, the electronic structures of InMO 4 (M = V, Nb and Ta) and BiVO 4 were reported by Oshikiri et al. based on the first principles calculations [37] . The conduction bands of InMO 4 (M = V, Nb and Ta) are mainly composed of a dominant d orbital component from V 3d, Nb 4d and Ta 5d orbitals, respectively. The valence bands of BiVO 4 are composed of a small Bi 6s orbital component and a dominant O 2p orbital component. Based on above analysis, the conduction band of Bi 2 YVO 8 is composed of V 3d orbital component. The valence band of Bi 2 YVO 8 is composed of a small Bi 6s orbital component and a dominant O 2p orbital component. In order to find the most optimum amount of the cocatalyst loaded on the surface of Bi 2 YVO 8 , the optimum conditions loading the three kinds of Pt, NiO and RuO 2 cocatalysts on the Bi 2 YVO 8 photocatalyst have been investigated. Fig. 11 shows the photocatalytic H 2 evolution rates under ultraviolet light irradiation over Bi 2 YVO 8 loaded by different amounts of the Pt, NiO or RuO 2 cocatalyst. In these experiments, Bi 2 YVO 8 (1.0 g) was suspended in an aqueous CH 3 OH/H 2 O solution and a 400 W high-pressure Hg lamp was used. The amount of the Pt cocatalyst was varied from 0.05 to 0.40 wt%. It can be seen from Fig. 11 that, at 0.2 wt% loading of Pt on the Bi 2 YVO 8 photocatalytic, the maximum photocatalytic activity for H 2 evolution was obtained. The amount of the NiO cocatalyst was varied between 0.20 and 1.80 wt%. At 1.0 wt% loading of NiO on the Bi 2 YVO 8 photocatalyst, the maximum photocatalytic activity for H 2 evolution was obtained. The amount of the RuO 2 cocatalyst was varied from 0.20 to 1.80 wt%. At 1.0 wt% loading of RuO 2 on the Bi 2 YVO 8 photocatalyst, the maximum photocatalytic activity for H 2 evolution was obtained. Fig. 12 shows the photocatalytic H 2 evolution rates under ultraviolet light irradiation over Bi 2 YVO 8 loaded by Pt, NiO and RuO 2 cocatalysts (50 mL CH 3 OH, 300 mL H 2 O, 1.0 g catalyst). In order to improve the photocatalytic activity of Bi 2 YVO 8 , the cocatalyst was used in our experiment because the photoinduced electrons preferentially enriched on the surface of cocatalyst particles, as a result, the recombination of the photoinduced electrons and the photoinduced holes were markedly suppressed. We concluded that the most suitable amounts of cocatalysts on Bi 2 YVO 8 were 0.2 wt% for Pt cocatalyst and 1 wt% for NiO and RuO 2 cocatalysts, respectively. Thus the 0.2 wt%-Pt/Bi 2 YVO 8 , 1.0 wt%-NiO/Bi 2 YVO 8 and 1.0 wt%-RuO 2 /Bi 2 YVO 8 were prepared. The photonic efficiency of 0.2 wt%-Pt/Bi 2 YVO 8 was found to be 5.329%, indicating higher photocatalytic activity compared to the 1.0 wt%-NiO/Bi 2 YVO 8 ( φ = 3.943%) and the 1.0 wt%-RuO 2 /Bi 2 YVO 8 ( φ = 1.641%) for the H 2 evolution. In addition, Pt metal is regarded as the most suitable cocatalyst for enhancing the photocatalytic activity of Bi 2 YVO 8 . For 0.2 wt%-Pt/Bi 2 YVO 8 , 1.0 wt%-NiO/Bi 2 YVO 8 and 1.0 wt%-RuO 2 /Bi 2 YVO 8 , the turnover numbers in terms of reacted electrons relative to the amount of catalyst exceeded 3.8477, 2.6256 and 1.1348, respectively, after 14 h of reaction time under ultraviolet light irradiation. The turnover number was enough to illustrate that hydrogen production is of photocatalytic nature. Fig. 13 shows the photocatalytic H 2 evolution from the aqueous CH 3 OH/H 2 O solution (50 mL CH 3 OH, 300 mL H 2 O, 1.0 g catalyst) with Bi 2 YVO 8 , Bi 2 YTaO 7 or Bi 2 InTaO 7 as the photocatalyst under ultraviolet light irradiation. Similarly, 0.2 wt% Pt cocatalyst was loaded on the surface of above photocatalysts. The H 2 evolution rates were estimated to be 0.40 mmol h −1 for Bi 2 YVO 8 ( φ = 5.329%), 0.11 mmol h −1 for Bi 2 YTaO 7 ( φ = 1.453%) and 0.36 mmol h −1 for Bi 2 InTaO 7 ( φ = 4.754%), demonstrating that Bi 2 YVO 8 exhibits higher activity than that of Bi 2 YTaO 7 and Bi 2 InTaO 7 . For 0.2 wt%-Pt/Bi 2 YVO 8 , 0.2 wt%-Pt/Bi 2 InTaO 7 and 0.2 wt%-Pt/Bi 2 YTaO 7 , the turnover numbers in terms of reacted electrons relative to the amount of catalyst exceeded 3.8477, 4.1267 and 1.2217, respectively, after 14 h of reaction time under ultraviolet light irradiation. The turnover number was enough to illustrate that hydrogen production is of photocatalytic nature. MB degradation with Bi 2 YVO 8 , Bi 2 YTaO 7 [29] , Bi 2 InTaO 7 or TiO 2 (P-25) as the photocatalyst under visible light irradiation ( λ > 420 nm) in oxygen-saturated suspension ([O 2 ] sat = 1.02 × 10 −3 M), are shown in Fig. 14 . By means of the Bi 2 YVO 8 photocatalyst, the results showed that the solution color changed from deep blue to colorless and MB was not detected after visible light irradiation for 170 min. Simultaneously, the initial rate of MB degradation was about 4.96 × 10 −6 mM s −1 and SO 4 2− ion concentration of 0.0339 mM was detected in the solution after the photocatalytic reaction for 170 min, indicating that 67% of sulfur from MB was converted into sulfate ion. During the removal of MB, the photodegradation intermediates of MB were azure B, azure A, azure C, thionine and phenothiazine [38] , then the methyl groups were converted to smaller organic species and ultimately mineralized to inorganic products such as CO 2 , NH 4 + , NO 3 − and SO 4 2− [38] . The sulfur was first hydrolytically removed, then subsequently oxidized and transformed into SO 4 2− , at the same time, nitrogen atoms in the −3 oxidation state produced NH 4 + cations, subsequently converted into NO 3 − ions [39] . It is clear that aqueous MB is mainly mineralized rather than being bleached under our experimental conditions due to the decrease of the TOC (100%) after 180 min irradiation. The initial photonic efficiency was estimated to be 0.11% for Bi 2 YVO 8 . By means of the Bi 2 InTaO 7 photocatalyst, MB concentration decreased only from 0.0506 to 0.0365 mM after visible light irradiation for 170 min. In parallel, the initial rate of MB degradation and the photonic efficiency were about 1.38 × 10 −6 mM s −1 and 0.031%, respectively. By means of the Bi 2 YTaO 7 photocatalyst, MB concentration decreased from 0.0506 to 0.0089 mM after visible light irradiation for 170 min. The initial rate of MB degradation and the photonic efficiency were 4.09 × 10 −6 mM s −1 and 0.091%, respectively. The surface areas of Bi 2 YTaO 7 , Bi 2 InTaO 7 and Bi 2 YVO 8 were 1.23, 1.26 and 1.12 m 2 g −1 , respectively. This clearly points out that the higher photocatalytic activity of Bi 2 YVO 8 is not attributed to the large surface area. In comparison with above results, photobleaching of MB (MB photolysis) in the absence of catalyst was also carried out under visible light irradiation and the results are described in Fig. 14 . The photonic efficiency was estimated to be 0.027%. By means of the TiO 2 photocatalyst, visible light irradiation for 170 min led to the decrease of MB concentration from 0.0506 to 0.0359 mM by a photonic efficiency of 0.027% and SO 4 2− ion was not detected in the solution after the photoreaction. The results indicate that the rate of MB photolysis is almost identical to that of MB degradation with TiO 2 as the catalyst, suggesting that TiO 2 is completely inactive to MB photocatalytic degradation under visible light irradiation. Zhao et al. reported that alizarin red and X3B dyes could be degraded over the TiO 2 photocatalyst based on visible light driven dye-sensitized mechanism [40] . Tang et al. also concluded that the photocatalytic degradation of MB over TiO 2 based on dye-sensitized process were very limited under visible light irradiation [16] . The ultimate goal of the organic pollutants photodegradation is to completely convert the toxic organic compounds into inorganics, such as CO 2 , SO 4 2− or NO 3 − , etc. By means of the Bi 2 YVO 8 photocatalyst, the dependence of MB degradation products on the irradiation time is compared in Fig. 15 . The concentration of SO 4 2− or NO 3 − ions increased with increasing irradiation time. It was noteworthy that the amount of SO 4 2− ions released into the solution was lower than the stoichiometric amount. The first possible reason is due to the loss of volatile sulfur-containing compounds such as SO 2 during photoreaction. The second explanation is given by the partially irreversible adsorption of SO 4 2− ions on the surface of the photocatalyst as already observed by Lachheb et al. [39] . The total organic carbon was also determined during visible light irradiation and the results are shown in Fig. 16 . The results showed that 27.8 or 82.3% of TOC decrease was obtained after visible light irradiation for 170 min with Bi 2 InTaO 7 or Bi 2 YTaO 7 [29] as the photocatalyst. By means of the Bi 2 YVO 8 photocatalyst, a significantly enhanced decrease of the TOC (99.9%) was obtained after visible light irradiation for 170 min, in succession, the complete mineralization of MB was achieved after 180 min irradiation due to the decrease of the TOC (100%). In order to understand the influence of the dissolved O 2 on the catalytic activity of Bi 2 YVO 8 for MB photodegradation, the dissolved O 2 in MB solution was removed by purging the solution with N 2 for 3 h prior to the photoreaction. The photodegradation of MB catalyzed by Bi 2 YVO 8 under visible light irradiation in the absence of dissolved O 2 is described in Fig. 17 . The initial rate of MB degradation was 2.56 × 10 −6 mM s −1 , and the photonic efficiency was 0.057%, which was obvious lower compared to MB photodegradation by the existence of dissolved O 2 owing to the photocatalyzed hydration effect. Above results points out that the existence of dissolved O 2 enhances the catalytic activity of Bi 2 YVO 8 for MB photodegradation. In principle, the direct absorption of photons by Bi 2 YVO 8 leads to the generation of excited electrons in the conduction band and photogenerated holes in the valence band of Bi 2 YVO 8 . The excited electrons in the conduction band of Bi 2 YVO 8 can be captured by surface sorbed O 2 , which eventually suppress the recombination of photogenerated electrons and photogenerated holes. As a result, the photogenerated holes in the valence band of Bi 2 YVO 8 can serve as the oxidizing species for inducing MB photodegradation. Fig. 18 shows the effect of Pt, NiO and RuO 2 cocatalysts on the photocatalytic methylene blue degradation activity under visible light irradiation with oxygen-saturated suspension ([O 2 ] sat = 1.02 × 10 −3 M) by means of Bi 2 YVO 8 . By means of the 0.2 wt%-Pt/Bi 2 YVO 8 photocatalyst, the solution color changed from deep blue to colorless and MB was not detected after visible light irradiation for 120 min, and the initial rate of MB degradation and the photonic efficiency were 7.03 × 10 −6 mM s −1 and 0.156%, respectively. By means of the 1.0 wt%-NiO/Bi 2 YVO 8 photocatalyst, the solution color changed from deep blue to colorless and MB was not detected after visible light irradiation for 140 min, and the initial rate of MB degradation and the photonic efficiency were 6.02 × 10 −6 mM s −1 and 0.133%, respectively. By means of the 1.0 wt%-RuO 2 /Bi 2 YVO 8 photocatalyst, the solution color changed from deep blue to colorless and MB was not detected after visible light irradiation for 150 min, and the initial rate of MB degradation and the photonic efficiency were 5.62 × 10 −6 mM s −1 and 0.125%, respectively. Based on above analysis, the photocatalytic methylene blue degradation activity was enhanced by loading Pt, NiO or RuO 2 cocatalyst on the surface of Bi 2 YVO 8 . The 0.2 wt%-Pt/Bi 2 YVO 8 photocatalyst showed higher photocatalytic activity compared to the 1.0 wt%-NiO/Bi 2 YVO 8 photocatalyst and the 1.0 wt%-RuO 2 /Bi 2 YVO 8 photocatalyst for the photocatalytic methylene blue degradation. 4 Conclusions Bi 2 YVO 8 was prepared by solid-state reaction method and the structural, optical absorption and photocatalytic properties of Bi 2 YVO 8 were investigated. Bi 2 YVO 8 has the tetragonal crystal system with space group I 4/ mmm and the band gap of Bi 2 YVO 8 is 2.09 eV, indicating that Bi 2 YVO 8 can be used as a visible light responsible photocatalyst. In addition, Bi 2 YVO 8 is capable of catalyzing water splitting to generate H 2 or O 2 under ultraviolet light irradiation. By means of the Bi 2 YVO 8 photocatalyst, photocatalytic decomposition of aqueous MB can be achieved under visible light irradiation. The mineralization of aqueous MB leads to the generation of SO 4 2− and NO 3 − , then result in the marked decrease of TOC during the reaction, which suggests that Bi 2 YVO 8 /VIS system can be used as an effective method for the treatment of the wastewater from the textile industry. Acknowledgement This work is supported by a grant from the Natural Science Foundation of Jiangsu Province (No. BK2006130, BK2007717). References [1] K. Honda A. Fujishima Nature 238 1972 37 [2] B. Neppolian H. Yamashita Y. Okada H. Nishijima M. Anpo Catal. Lett. 105 2005 111 [3] M. Takeuchi G. Martra S. Coluccia M. Anpo J. Phys. Chem. B 109 2005 7387 [4] Z.G. Zou J.H. Ye K. Sayama H. Arakawa Nature 414 2001 625 [5] S.R. Trenor T.E. Long B.J. Love J. Adhes. 81 2 2005 213 [6] S.R. Trenor T.E. Long B.J. Love Eur. Polym. J. 41 2 2005 219 [7] T.P. Treynor S.G. Boxer J. Phys. Chem. A 108 10 2004 1764 [8] S.R. Trenor T.E. Long B.J. Love Macromol. Chem. Phys. 05 6 2004 715 [9] S.R. Trenor A.R. Shultz B.J. Love T.E. Long Chem. Rev. 104 6 2004 3059 [10] T.P. Treynor S.S. Andrews S.G. Boxer J. Phys. Chem. 107 40 2003 11230 [11] S. Trenor K. Renshaw M. Marek K.E. Forsten B. Love J. Adhes. Sci. Technol. 15 10 2001 1189 [12] J.B. Macchesney D.W. Johnson S. Bhandarkar M.P. Bohrer J.W. Fleming E.M. Monberg D.J. Trevor J. Non-crystalline Solids 226 3 1998 232 [13] J.L. Trevor K.R. Lykke M.J. Pellin L. Hanley Langmuir 14 7 1998 1664 [14] Z.G. Zou J.H. Ye H. Arakawa J. Mater. Sci. Lett. 19 2000 1909 [15] M. Anpo M. Takeuchi J. Catal. 216 2003 505 [16] J. Tang Z.G. Zou J. Yin J.H. Ye Chem. Phys. Lett. 382 2003 175 [17] M.M. Haque M. Muneer D.W. Bahnemann Environ. Sci. Technol. 40 15 2006 4765 [18] J. Marugan D. Hufschmidt M.J. Lopez-Munoz V. Selzer D.W. Bahnemann Appl. Catal. B 62 3–4 2006 201 [19] M. Muneer D.W. Bahnemann M. Qamar M.A. Tariq M. Faisal Appl. Catal. A 289 2 2005 224 [20] D. Bahnemann Sol. Energy 77 5 2004 445 [21] H. Yamashita K. Maekawa H. Nakao M. Anpo Appl. Surf. Sci. 237 1–4 2004 393 [22] R. Asahi T. Morikawa T. Ohwaki K. Aoki Y. Taga Science 293 2001 269 [23] P. Mohapatra K.M. Parida J. Mol. Catal. A: Chem. 258 1–2 2006 118 [24] Y.J. Jang C. Simer T. Ohm Mater. Res. Bull. 41 1 2006 67 [25] X.L. Yan T. Ohno K. Nishijima R. Abe B. Ohtani Chem. Phys. Lett. 429 4–6 2006 606 [26] Z. Zou J. Ye K. Sayama H. Arakawa J. Photochem. Photobiol. A: Chem. 148 2002 65 [27] J.H. Wang Z.G. Zou J.H. Ye J. Phys. Chem. Solids 66 2005 349 [28] J. Marugán D. Hufschmidt G. Sagawe V. Selzer D. Bahnemann Water Res. 40 2006 833 [29] J.F. Luan X.P. Hao S.R. Zheng G.Y. Luan X.S. Wu J. Mater. Sci. 41 23 2006 8001 [30] G.M. Veith M.V. Lobanov T.J. Emge M. Greenblatt M. Croft F. Stowasser J. Hadermann G. Van Tendeloo J. Mater. Chem. 14 10 2004 1623 [31] L.W. Whaley M.V. Lobanov D. Sheptyakov M. Croft K.V. Ramanujachary S. Lofland P.W. Stephens J.H. Her G. Van Tendeloo M. Rossell M. Greenblatt Chem. Mater. 18 15 2006 3448 [32] V.V. Poltavets K.A. Lokshin T. Egami M. Greenblatt Mater. Res. Bull. 41 5 2006 955 [33] G.M. Veith M. Greenblatt M. Croft K.V. Ramanujachary J. Hattrick-Simpers S.E. Lofland I. Nowik Chem. Mater. 17 10 2005 2562 [34] M.V. Lobanov S.W. Li M. Greenblatt Chem. Mater. 15 6 2003 1302 [35] K.V. Ramanujachary J.E. Sunstrom I. Fawcett P. Shuk M. Greenblatt M. Croft I. Nowik R.H. Herber S. Khalid Mater. Res. Bull. 34 5 1999 803 [36] J. Tauc R. Grigorovici A. Vancu Phys. Stat. Sol. 15 1966 627 [37] M. Oshikiri M. Boero J.H. Ye Z.G. Zou G. Kido J. Chem. Phys. 117 2002 7313 [38] T.Y. Zhang T. Oyama A. Aoshima H. Hidaka J.C. Zhao N. Serpone J. Photochem. Photobiol. A: Chem. 140 2001 163 [39] H. Lachheb E. Puzenat A. Houas M. Ksibi E. Elaloui C. Guilard J.M. Herrmann Appl. Catal. B 39 2002 75 [40] G. Liu T. Wu J. Zhao H. Hidaka N. Serpone Environ. Sci. Technol. 33 1999 2081
更多查看译文
关键词
Photocatalyst,Bi,2,FeVO,7,structural characterization,photocatalytic properties,photocatalytic water splitting reaction,photocatalytic degradation
AI 理解论文
溯源树
样例
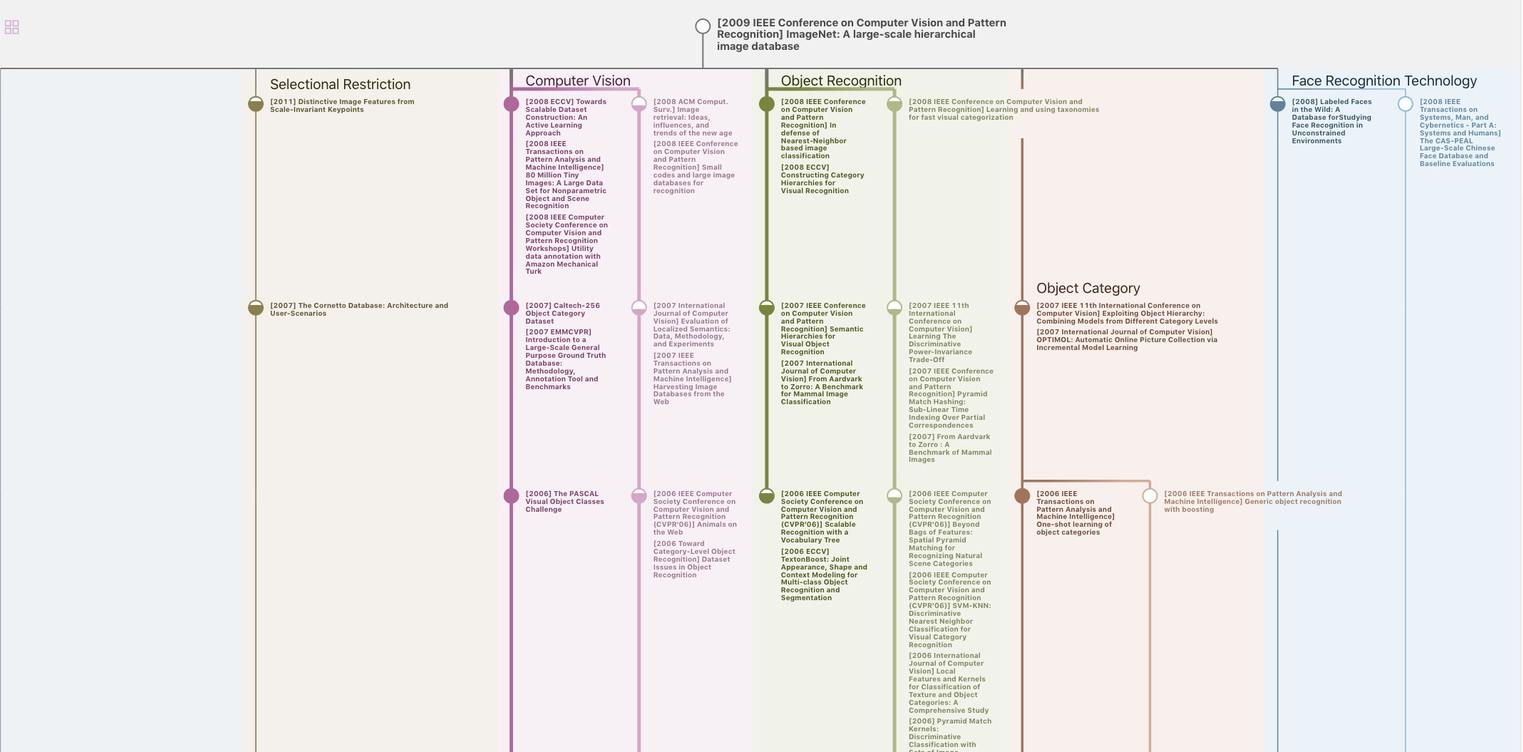
生成溯源树,研究论文发展脉络
Chat Paper
正在生成论文摘要