Estimated Dose to the Rectum and Colon in Prostate Cancer Patients Treated with Exclusive Radiation Therapy Presenting a Secondary Colorectal Malignancy
Clinical Oncology(2009)
摘要
Results The median maximum, mean and minimum doses delivered to the colon or rectum affected by the sCRC were 39.3 (range 0.2–66.0), 5.4 (range 0.2–41.3) and 0.6 (range 0.2–7.8) Gy, respectively. All but three sCRCs occurred outside the treatment fields. The estimated rectal doses after prostate radiation therapy were substantially higher than those delivered to non-rectal colic structures (mean dose 47.2 ± 16.6 vs 9.4 ± 6.4Gy), but only one (9%) patient presented a rectal cancer. The differential mean doses given to the rectosigmoid junction and sigmoid colon, with or without sCRC, were not different. Conclusions These data suggest that the administered dose after EBRT for prostate cancer to the colon, excluding the rectum, may be below the Gy unit in sCRC patients. Key words Prostate cancer radiation therapy secondary colorectal cancer Introduction Prostate cancer is the most common malignancy among men, with a lifetime risk of about 17% [1] in the USA and is associated with a substantial cancer death rate (5–10 per 100 000 men) [2] . Surgery, external beam radiation therapy (EBRT) and brachytherapy are definitive therapies for patient with localised prostate cancer. Recent data suggest that these treatments yield similar results in the prostate-specific antigen-based area, even if EBRT data are fraught with selection bias and differences in clinical vs pathological staging. As such, the long-term adverse effects of these curative treatments are of paramount importance for patients. Radiation therapy has been linked with secondary cancers, including, but not limited to [3–5] , bladder [6,7] , colon [8] and rectal [8,9] malignancies. The consequential contribution of radiation therapy for prostate cancer to the risk of secondary cancers remains somewhat controversial. Radiation-induced cancers should occur within the radiation fields and after a latency period of >5 years [10] , with peak frequency between 5 and 10 years [8] . However, several investigators have not observed an increase in secondary bladder or rectal cancers after prostate radiation therapy [6,7,11,12] , or have reported that a majority of these secondary malignancies occurs synchronously with the prostate cancer [13] . These discrepant results may be due to confounding factors, such as the non-assessable individual genetic predisposition to spontaneous cancer in cancer registry studies, differences in underlying rates in the populations studied or differences among patients regarding their indication for radical treatment or smoking history, among other factors. A major shortcoming of epidemiological data suggesting an increased risk of secondary colorectal cancers (sCRCs) after radiation therapy is the absence of information regarding radiation target volume, radiation dose, fractionation scheme and doses delivered to the organs at risk in the vicinity of the target volume. Here we report the estimated dose to the colorectal structures of study patients, modelled on index prostate cancer patients with sCRCs treated at our institution. Patients and Methods Patient Population Men with localised prostate cancer diagnosed between January 1980 and December 1998 were identified from the Geneva Cancer Registry, which records all incident cancer cases occurring in the population of the canton since 1970 [14] . In total, there were 1134 men in the database who had localised prostate cancer as their first primary tumour who survived ≥5 years after their initial diagnosis, and who received either radiation therapy ( n = 264), with or without androgen deprivation therapy or surgery without radiation ( n = 870) as their first course of therapy. Of these former 264 individuals, 11 (4.2%) presented with sCRC at follow-up. No index patient presented with a family history of colorectal cancer. The index patients' characteristics are detailed in Table 1 . No patient underwent androgen deprivation therapy. A median dose of 66 Gy (range 4–70) was delivered to these patients. EBRT had to be interrupted in one patient after two fractions (4 Gy), as he presented a continuous state of acute alcoholic intoxication. Another patient refused the boost delivery after pelvic EBRT (50 Gy). The median weight loss during EBRT (index patients) was 0 kg (range −0.5 to +0.6). The median estimated doses administered to the prostate and seminal vesicles are detailed in Table 2 . sCRCs were observed 75–145 months (median 96.5) after prostate EBRT. sCRC locations were: transverse colon ( n = 3), rectosigmoid junction (RSJ; n = 2), sigmoid colon ( n = 2), ascending colon ( n = 1), descendant colon ( n = 1), caecum ( n = 1) and rectum ( n = 1). The characteristics of these tumours, with the type of surgery, adjuvant treatment and presenting symptoms, are detailed in Table 3 . One patient presented with two asynchronous sCRCs ( Table 3 ; patient no. 11; first descending, then ascending colic adenocarcinoma). The departmental files and portal simulations for two-dimensional EBRT ( n = 9) or 3D conformal radiotherapy (CRT; n = 2) of these patients were searched and information regarding treatment technique (pelvic EBRT and boost, skin to surface distance [SSD]), total dose and dose per fraction, target volumes and individual patient characteristics (patient's weight and thickness) were extracted. The treatment was delivered with 10 MV photon beams for all but two patients (18 MV, 3D-CRT). A four- and six-field beam arrangement was chosen for patients treated with conventional EBRT and 3D-CRT, respectively. Typically, the size of these fields was 10 × 10 and 7 × 7 cm for the ‘pelvic’/boost fields and 8.5–8.6 × 8.5–8.6 cm for the conventional and 3D-CRT fields, respectively. As such, the conventional radiation therapy field size can be defined as ‘mini-pelvis’ [15] . Patient Models To estimate the dose delivered to the colon and rectum, each individual index patient, treated between June 1980 and January 1994, had to be modellised with a matching study case, as the former patients did not have planning computed tomography. These study cases were identified using computed tomography datasets in our institutional database. These computed tomography datasets were acquired between November 2000 and January 2006. First, to optimally define the index patient model, 22 prostate cancer patients' computed tomography scans, matching the studied cases' individual (weight and thickness), and treatment (SSDs) characteristics, were pre-selected. All patients had undergone between one and seven (median: four) computed tomography scans for EBRT simulation and positioning weekly verification. One index patient had only one computed tomography simulation (patient no. 11), as the matched study case underwent only a computed tomography scan procedure for simulation. The weekly imagery prerequisite was felt necessary, when possible, as the volumes of colon or rectum can substantially alter during EBRT [16] , thus modifying substantially the estimated dose to these non-target structures. It was our central tenet that evaluating the dose to non-target volumes on only one computed tomography dataset was too imprecise an evaluation to precisely define the effective administered dose to these patients with sCRCs. As such, all calculated colic or rectal doses on serial computed tomography were averaged. Second, each index patient's characteristics (weight and thickness) were plotted in a scatter diagram, relative to the characteristics of the 11 best study cases ( Fig. 1 ). Index cases were identified by numbers (1–11), whereas study cases were identified by letters (a–v). The most suitable study patient for the index case was chosen from this pictogram ( Fig. 1 ). As such, 11 of the 22 study cases were matched with the 11 index individuals with secondary colon or rectum cancer ( Table 1 ). To validate the model of the present study, four patients recorded in our institutional database were chosen using this selection formula ( Fig. 1 ). Planning was carried out using the typical treatment of the index cases (median value pelvic field). The minimum doses computed for the rectum, RSJ, sigmoid, descending colon, ascending colon and ceacum were 11.8 ± 0.7, 0.8 ± 0.2, 0.3 ± 0.1, 0.1 ± 0.0, 0.1 ± 0.0 and 0.13 ± 0.1 Gy, respectively. The median weight loss during EBRT for the study patients was 1.3 kg (range 0–3.6). The best matching study case was selected and dose calculations, with the index case treatment characteristics, were carried out ( Table 1 ). Prostate, Seminal Vesicles, Colon and Rectum Delineation All volumes were outlined by one investigator to minimise potential biases associated with multiple planners. The target volumes (prostate and seminal vesicles), colon and rectum were outlined in the appropriate axial computed tomography slices on a virtual simulation workstation (AcQSim System, Philips Medical System, Cleveland, OH, USA) by a senior radiation oncologist (HW) and the colic structures were reviewed by a senior diagnostic radiologist specialised in gastrointestinal radiology (AR). No attempt was made to delineate the small intestine, as no secondary cancer was observed on this structure. Table 4 details the prostate/seminal vesicles and colon/rectum volumes. Planning of External Beam Radiation Therapy on Study Cases All study cases were transferred to the virtual simulation workstation. First, the isocentre of the index case was duplicated in the study case, using the technical data recorded in the medical file and portal films. Block apertures of index patients were reproduced after measuring their respective position relative to the field borders. Second, the pelvic and boost fields of the index cases were created on the study cases' computed tomography datasets. Blocks were reproduced and wedges, if applicable, introduced. Third, dose calculations were carried out with our treatment planning system (Eclipse, Varian Medical Systems, Paolo Alto, CA, USA) using 6 MV photons, except for the two 3D-CRT cases treated with 18 MV photon beams. Statistics For descriptive characteristics of participants, models and treatments we used means, medians, ranges and standard deviations. To assess the possible contribution of radiation in the development of secondary malignancies in patients with sCRCs, we compared the mean dose received by organs that developed cancers with the mean dose received by organs that did not develop secondary cancers. To account for patients' individual treatment characteristics, we ranked the dose received by each individual organ for each patient from 1 (smallest dose) to 7 (highest dose). Each patient was then paired to himself and an overall comparison of the mean radiation scores received by the organs with and without sCRC using the Wilcoxon signed rank test. A value of P < 0.05 was considered significant. We carried out all analyses using the Statistical Package for Social Sciences (SPSS-Version 15.0.1 SPSS Inc, Chicago, IL, USA). Results Table 5 displays a summary of the estimated dose delivered to the gastrointestinal structures with and without sCRCs. Only a minority of sCRC patients ( n = 3) received doses ≥25 Gy to the colon or rectum affected by the malignancy ( Table 5 ). All but three sCRCs occurred outside the treatment fields ( Table 5 ; RSJ, two patients; rectum, one patient). Interestingly, although the estimated rectal doses were substantially higher than those delivered to non-rectal colic structures, only one (9%) patient presented a rectal cancer (patient no. 6; Table 5 ). The estimated mean doses delivered to the rectum ranged between 3.1 (2.1–4.2) and 64.1 (23.9–68.2) Gy, whereas the mean doses delivered to non-rectal structures ranged between 0.5 (0.0–4.1) and 18.9 (0.0–67.0) Gy ( Table 5 ). When comparing the estimated mean dose received by gastrointestinal structures with and without sCRCs, no significant difference was observed ( P = 0.47). The estimated mean doses administered to the gastrointestinal structures with sCRC ranged between <0.1 (0.0–0.1) and 41.3 (7.2–50.5) Gy. For the gastrointestinal structures without sCRC, the observed estimated mean doses ranged between 1.1 (0.0–4.2) and 31.4 (0.4–68.2) Gy ( Table 6 ). Discussion EBRT for prostate cancer has been associated with a small, but statistically significant, enhancement in the risk of secondary cancers in many cancer registry-based studies [3,5] . This increasing risk of cancers increases with longer survival (≥5 years and ≥10 years) in the EBRT group compared with the surgery-only group [5] . These secondary solid tumours are mainly located in the pelvis (bladder, rectum), but a substantial number of cancers have been observed in extra-pelvic location, such as the lung [3,5] , stomach, oesophagus, transverse colon, brain or larynx [3] . Radiation-induced cancers are classically defined by the following criteria: (1) tumours should have a different histological diagnosis (i.e. non-adenocarcinoma or non-prostate-specific antigen immunohistochemistry staining adenocarcinoma); (2) an appropriate latent period should be observed between the treatment of the primary tumour and the secondary cancer; (3) secondary cancers should be within the radiation treatment field. Many investigators consider that secondary cancer occurring out of treatment fields makes radiation an unlikely cause of cancer induction [12] . Applying strictly these criteria to our data, only a minority of our observed sCRCs (3/11) could be considered to be radiation induced. However, in a large Surveillance, Epidemiology, and End Results (SEER) study, patients receiving EBRT for prostate cancer had significantly higher odds of secondary cancers occurring at supra-diaphragmatic sites (i.e. out field locations) or other areas not directly involved with radiation therapy [3] . Likewise, the risk of developing lung cancer ≥10 years was significantly ( P = 0.02) larger after EBRT compared with surgery in another prostate cancer SEER study [5] , although the effect of potential confounders, such as smoking history, was not directly assessed in this study. As such, epidemiological data suggest that very low dose radiation delivered to structures at a distance from the target volume may indeed increase the probability of cancer induction. The magnitude of this scattered dose is below the Gy unit. Regarding lung cancers, Francois et al. [17] , analysing the dose distribution to organs outside the treatment beams for prostate cancer, observed an average of 0.6 Gy delivered to the lung. Likewise, the average lung dose associated with pelvic radiation therapy (cervical cancer) was about 0.3 Gy [18,19] . For colon cancer, the scattered dose estimated in our study was on average 0.6 Gy for the ascending, transverse and descending colon ( Table 5 ), more than two orders of magnitude less than the dose to the prostate. Considering the lack of published dosimetric data, we decided to model our index sCRC patients to analyse specifically the dose deposition to colon and rectal structures. The main assumption was that our study patients would approximately reproduce the colon and prostate anatomy of the index cases, based on SSD, body weight and body thickness. The estimated dose delivery to the prostate and seminal vesicles seems to be appropriate ( Table 2 ). We assumed that, all things being equal, the study patients would match the index sCRC case anatomy, providing that no significant weight loss was observed in both groups during radiation therapy and that the study patient was imaged repeatedly to account for organ motion. The weight loss in our cohort was ≤1% in both groups and all but one patient had multiple computed tomography. The mean estimated dose delivered to the colon or rectum with sCRCs was 5.4 Gy ( Table 5 ). Although the rectum received a higher estimated dose compared with non-rectal structures, only one cancer occurred at this site. Additionally, the dose analysis showed that structures, with or without sCRC, received an estimated similar dose. These data may suggest that radiation has no cancer-induction threshold and that the probability does not increase with dose ( Table 5 ). As such, this study may add dosimetric discordance to the stochastic effect of radiation-induced carcinogenesis. These results may be in keeping with the SEER data that showed that men receiving radioactive implants, when compared with those receiving EBRT, had the lowest odds of developing second cancers [3] , albeit receiving a higher radiation dose to the prostate, although this difference was not significant in a subsequent analysis using eligible patients accrued in an extended period of time [20] . At this juncture, given the potential increase in secondary cancers with radiation and the scattered dose delivered to organs at risk during prostate EBRT, a strong case could be argued against intensity-modulated radiation therapy. Intensity-modulated radiation therapy increases substantially the integral patient dose and pelvic organs at risk at the low-dose level for prostate cancer [21] . Several counterarguments should, however, be considered. First, epidemiological data are inconsistent. A cohort study in the Detroit metropolitan area showed an increase in urinary bladder cancers after EBRT, but after excluding the first 2 months after prostate cancer diagnosis, this increase was no longer significant [13] . Likewise, the risk of second malignancy was assessed in prostate cancer patients using the British Columbia tumour registry [12] . A significant excess of bladder and testis cancer was observed in the non-radiation therapy group. As such, the magnitude of absolute and relative risks of secondary cancers after EBRT does not suggest deterring radiation therapy for prostate cancer. Second, late radiation-induced symptoms may trigger investigations that lead to cancer diagnosis. In the British Columbia study, about 50% of patients had one or more episodes of rectal bleeding [12] . It may well be that the observed increase in colon cancer in the radiation therapy group may be due to gastrointestinal investigations for these patients treated mainly with 60 Co units, that have led consequently to diagnosis of occult colon cancers that might have gone undiagnosed. Third, EBRT patients are often at high risk of secondary cancers because of their age and their co-morbidity/life style, and this factor may be more dominant than the radiation risk. Lastly, a minority of prostate cancer patients may have malignancy susceptibility and are thus prone to carcinogenesis. The occurrence of prostate cancer in hereditary non-polyposis colorectal cancer has been anecdotally reported [22] . One index patient in our study (patient no. 11; Table 5 ) subsequently presented with two asynchronous sCRCs and myelodysplastic syndrome. He subsequently died of acute myeloid leukaemia. This remarkable patient emphasises that a minority of cancer patients will ultimately develop non-treatment-related carcinogenesis, possibly as a result of an inherited or acquired genomic instability. There were several limitations of our study. First, our study was limited by the small sample size. Second, index patient modelling does not actually document the administered dose to these patients. Modelling was based on available parameters and it was assumed that the computed tomography from the study cases was an appropriate substitute for the index patient's dose computation. Third, dose calculations were carried out in all but two patients (index case was treated with 18 MV) using 6 and not 10 MV. It was assumed that the dose deposition during prostate EBRT is approximately identical for these two energies [23] . Fourth, an imbalance in tumour stage was observed between index and study cases ( Table 1 ), as a consequence of to the work-up used routinely at the time of diagnosis for these patients (i.e. digital rectal examination vs MRI). However, this stage imbalance did not have an effect on dose calculations, as the fields of the index cases were applied to the study patients. Moreover, the differential neutron dose generated with 6 and 10 MV EBRT was not accounted for in our dose computation model [24] . Finally, the statistical dose comparison of gastrointestinal structures with or without sCRC may have been underpowered, although the number of metrics per patients was substantial and an appropriate statistical method (each patient was assigned dose ranks) was used. Again, as mentioned earlier in the discussion, these data are in line with series on atomic bomb survivors suggesting an increased risk of cancer below the Gy unit, with no safe dose threshold. Conclusions Our data suggest that colon structures, excluding the rectum, RSJ or sigmoid, are irradiated to a mean dose <1 Gy during EBRT for prostate cancer. No increase in secondary cancer probability was observed with colorectal dose, as shown by this dose-computation analysis in 11 study patients, modellising 11 index cases. Acknowledging the limitation of this study, further research regarding dose administration to the colon/rectum and tumour induction after prostate cancer EBRT is justified in the framework of a future dose analysis study. References 1 Cancer Society Cancer statistics. American Cancer Society, cancer facts and figures Available at: http://www.cancer.org/downloads/STT/CAFF_finalPWSecured.pdf 2004 2 Albano JD, Ward E, Jemal A, et al. Cancer mortality in the United States by education level and race. J Natl Cancer Inst 2007; 99: 1384–1394. 3 K. Moon G.J. Stukenborg J. Keim Cancer incidence after localized therapy for prostate cancer Cancer 107 2006 991 998 4 P.A. Johnstone C.R. Powell R. Riffenburgh Second primary malignancies in T1-3N0 prostate cancer patients treated with radiation therapy with 10-year followup J Urol 159 1998 946 949 5 D.J. Brenner R.E. Curtis E.J. Hall Second malignancies in prostate carcinoma patients after radiotherapy compared with surgery Cancer 88 2000 398 406 6 A.I. Neugut H. Ahsan E. Robinson Bladder carcinoma and other second malignancies after radiotherapy for prostate carcinoma Cancer 79 1997 1600 1604 7 K. Chrouser B. Leibovich E. Bergstralh Bladder cancer risk following primary and adjuvant external beam radiation for prostate cancer J Urol 174 2005 107 110 [discussion 110–111] 8 S.W. Jao R.W. Beart Jr. H.M. Reiman Colon and anorectal cancer after pelvic irradiation Dis Colon Rectum 30 1987 953 958 9 N.N. Baxter J.E. Tepper S.B. Durham Increased risk of rectal cancer after prostate radiation: a population-based study Gastroenterology 128 2005 819 824 10 D.E. Thompson K. Mabuchi E. Ron Cancer incidence in atomic bomb survivors. Part II: solid tumors, 1958–1987 Radiat Res 137 1994 S17 S67 11 B. Movsas A.L. Hanlon W. Pinover Is there an increased risk of second primaries following prostate irradiation? Int J Radiat Oncol Biol Phys 41 1998 251 255 12 T. Pickles N. Phillips The risk of second malignancy in men with prostate cancer treated with or without radiation in British Columbia, 1984–2000 Radiother Oncol 65 2002 145 151 13 K.S. Pawlish D. Schottenfeld R. Severson Risk of multiple primary cancers in prostate cancer patients in the Detroit metropolitan area: a retrospective cohort study Prostate 33 1997 75 86 14 E. Rapiti G. Fioretta H.M. Verkooijen Increased risk of colon cancer after external radiation therapy for prostate cancer Int J Cancer 123 2008 1141 1145 15 M. Roach 3rd M. DeSilvio R. Valicenti Whole-pelvis, “mini-pelvis,” or prostate-only external beam radiotherapy after neoadjuvant and concurrent hormonal therapy in patients treated in the Radiation Therapy Oncology Group 9413 trial Int J Radiat Oncol Biol Phys 66 2006 647 653 16 C. Fiorino F. Foppiano P. Franzone Rectal and bladder motion during conformal radiotherapy after radical prostatectomy Radiother Oncol 74 2005 187 195 17 P. Francois C. Beurtheret A. Dutreix Calculation of the dose delivered to organs outside the radiation beams Med Phys 15 1988 879 883 18 R.A. Kleinerman J.D. Boice Jr. H.H. Storm Second primary cancer after treatment for cervical cancer. An International Cancer Registries study Cancer 76 1995 442 452 19 J.D. Boice Jr. N.E. Day A. Andersen Second cancers following radiation treatment for cervical cancer. An international collaboration among cancer registries J Natl Cancer Inst 74 1985 955 975 20 M. Abdel-Wahab I.M. Reis K. Hamilton Second primary cancer after radiotherapy for prostate cancer—a SEER analysis of brachytherapy versus external beam radiotherapy Int J Radiat Oncol Biol Phys 72 2008 58 68 21 Y. Tao D. Lefkopoulos D. Ibrahima Comparison of dose contribution to normal pelvic tissues among conventional, conformal and intensity-modulated radiotherapy techniques in prostate cancer Acta Oncol 47 3 2008 442 450 22 C. Soravia H. van der Klift M.A. Brundler Prostate cancer is part of the hereditary non-polyposis colorectal cancer (HNPCC) tumor spectrum Am J Med Genet A 121 2003 159 162 23 J.R. Tanner Measurement and comparison of prostate treatment dose to the femoral heads with 6-MV versus 10-MV photon energies Med Dosim 24 1999 279 285 24 F. d'Errico M. Luszik-Bhadra R. Nath Depth dose-equivalent and effective energies of photoneutrons generated by 6–18 MV X-ray beams for radiotherapy Health Phys 80 2001 4 11
更多查看译文
关键词
Prostate cancer,radiation therapy,secondary colorectal cancer
AI 理解论文
溯源树
样例
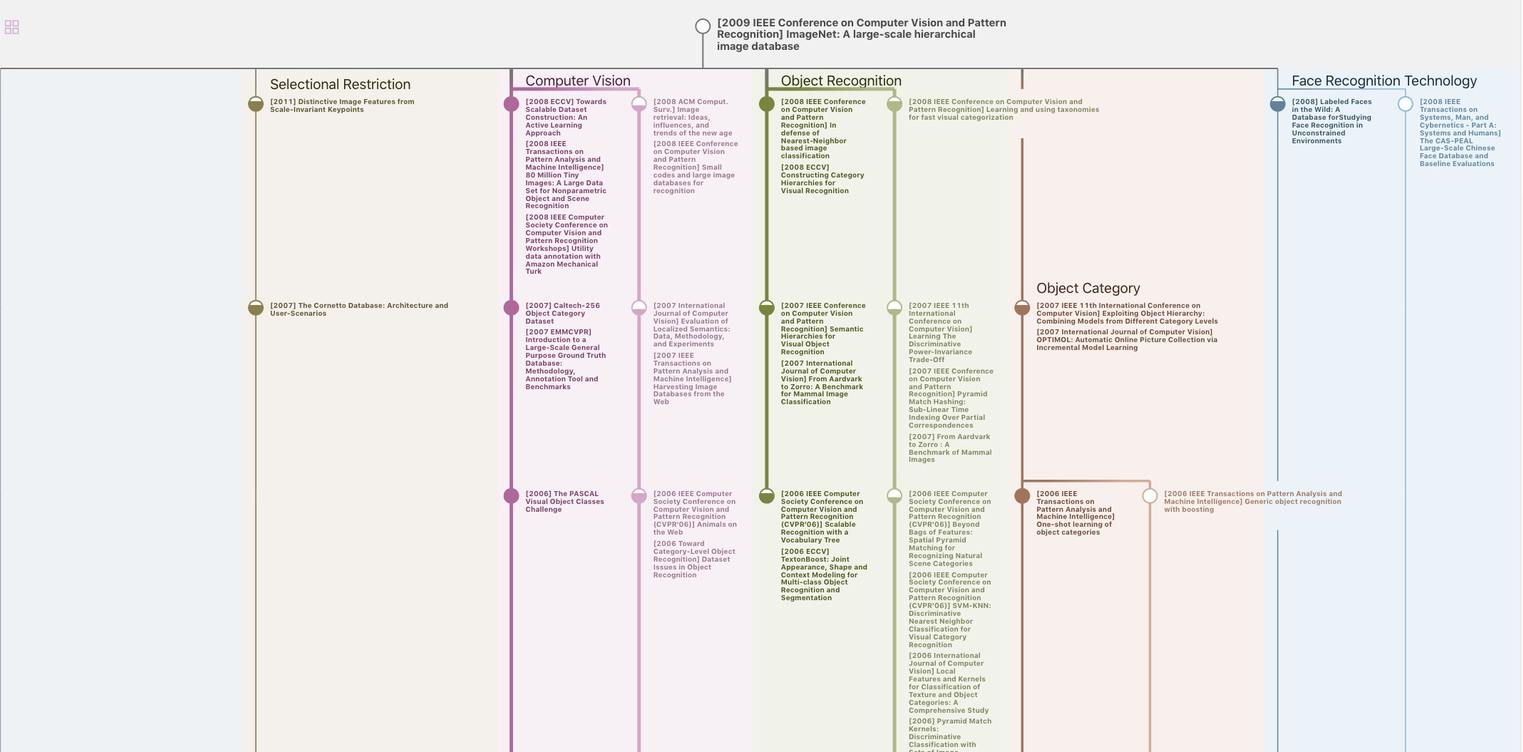
生成溯源树,研究论文发展脉络
Chat Paper
正在生成论文摘要