Seasonal and diel patterns of total gaseous mercury concentration in the atmosphere of the Central Valley of Costa Rica
Applied Geochemistry(2011)
摘要
Research highlights ► Elevated seasonal and daily patterns of total gaseous mercury (TGM) were observed in the Central Valley of Costa Rica 27 km south-east of the Poas volcano. ► With the onset of the wet season TGM in late April, values increased from typical values near 10 to 905 ng m −3 . ► During the day, TGM values increased until midday along with temperature and radiation but relative humidity showed a reciprocal pattern. ► We conclude that elemental mercury from the volcano may react with atmospheric oxidants especially during the dry season and the mercury would be deposited to the ground. ► With the onset of heavy rains, mercury in the soil is reduced and re-volatilized resulting in the high levels in atmospheric air at the end of April. Abstract Monitoring of Hg in the atmosphere near volcanoes is limited with no previous data for Costa Rica. Seasonal and daily patterns of total gaseous mercury (TGM) were observed at the main sampling location at the Universidad Nacional, Heredia, Costa Rica. The area (lat. 10.000230 long. −84.109499) is located in the Central Valley of Costa Rica and is 27 km SE of the Poas volcano (lat. 10.199486 long. −84.231388). Measurements were made from May 2008 to May 2009 at this location with some additional values obtained at other sites near the Poas volcano including San Luis and Grecia as well as near, Turrialba and Irazu volcanoes. Total gaseous Hg was determined in samples collected at a height of 2 m using the Tekran 2537A (Tekran Inc.) gas-phase Hg vapor analyzer. Meteorological data (temperature, relative humidity, wind speed, wind direction, radiation and precipitation) were obtained from the airport weather station located at Alajuela. Monthly precipitation is typically 85 mm during the dry season (December to April) with winds from the west. The wet season begins in late April and continues to December with monthly rainfall of 328 mm and winds from the NE. The annual mean temperature is 20 °C. With the onset of the wet season TGM increased from typical values near 10 to 905 ng m −3 . Measurements made within 5 km of the Poas volcano were higher than at Heredia at that time. Diel values measured at the university site increased until midday along with temperature and radiation. Relative humidity showed a reciprocal pattern. It was found that high values of TGM were not related to wind velocity or direction. The strong diel pattern increased with sunrise, peaked at midday and was lowest during the night time. It would seem that Hg 0 from the volcano is oxidized and is deposited to the soils during the dry season when winds are blowing from the volcano. With the onset of heavy rains in April, Hg in the soil is reduced and re-volatilized resulting in the high levels in the atmosphere. Values at other volcano sites were provided. The role of atmospheric pollutants such as H 2 O 2 and O 3 should be included in future studies as they may result in oxidation of reduced Hg. The instability in the air masses may also be a factor and local pollution sources may result in high levels that is emitted from the volcanoes of Hg being circulated to ground level as radiation intensity increases. 1 Introduction Elemental Hg (Hg 0 ) is a volatile metal and represents the predominant form in the atmosphere and is the form involved in long range transport ( Schroeder and Munthe, 1998; Steffen et al., 2008; Sunderland and Mason, 2007; Li et al., 2008 ). It is also the principal form of Hg released from volcanoes ( Pirrone et al., 1996; Nriagu and Becker, 2003 ). Since elemental Hg is not very soluble in water, wet deposition occurs following an oxidation step or directly as particulate Hg ( O’Driscoll et al., 2005 ). Mercury in wet deposition is principally in the form of oxidized (Hg 2+ ) Hg ( Boszke et al., 2003; Lin and Pehkonen, 1997; Han et al., 2004; Lindqvist et al., 1991; Schroeder, 1996; O’Driscoll et al., 2005 , and references therein). Both wet and dry deposition processes contribute to removal of Hg from the atmosphere ( Krug and Winstanley, 2004 ). In addition, particulate Hg is also scavenged or washed out during rain events. Since chemical speciation of the atmospheric Hg remains incomplete, the operational term used to describe Hg in the atmosphere is total gaseous mercury (TGM). Upon deposition to soils and water, oxidized Hg can be reduced and re-emitted to the atmosphere as Hg 0 ( O’Driscoll et al., 2003; Poisant et al., 2004 ). This can be either a photochemical reaction or a chemical reaction. Perhaps the most complete overview of the topic is presented by Schroeder et al. (2005) . They compared both micrometeorological techniques and dynamic flux chambers or both and related flux values to meteorological data. Flux rates from soil and water are known to be related to temperature, wind velocity, humidity and Hg substrate level. Researchers who calculate global emissions for Hg ( Pacyna et al., 2006 ) are unable to distinguish re-emitted Hg from that coming from human activities ( UNEP, 2008 ). Nevertheless, volcanoes have been thought to be a major “natural” source of Hg. Pirrone et al. (1996) have estimated that 5220 tonnes of Hg are emitted from all sources and less than half is considered to be “natural”. The total global volcanic emission of Hg is between 20% and 40% of total natural emissions where 75% of these emissions are sporadic eruptions rather than the large quantities of explosive eruptions ( Pyle and Matter, 2003 ). This amount should however be put into perspective as about 40% of the anthropogenic emissions are from burning coal and only 2% of the total is from volcanoes ( Nriagu and Becker, 2003 ). At the study location, there are other sources of Hg due to industrial activity and urban pollution of the Central Valley. In addition, it is known that there is volatilization from the oceans but these concentrations tend to be low ( Sunderland and Mason, 2007 ). Other emissions occur from mineral deposits of Hg ( Ferrara et al., 2000; Pyle and Matter, 2003; Nriagu, 1989; Pirrone et al., 1996 ) but such sites have not been identified. In Costa Rica, there are more than 120 volcanic centres, most of them old and extinct. This unique geological feature makes Costa Rica, especially the Central Valley an ideal study site. This location is called the Central Volcanic Range and is surrounded by some of the largest volcanoes in the country with the most prominent being Poas, Irazu and Turrialba ( Martínez et al., 2000; Barquero and Fernandez, 1990; Nriagu and Becker, 2003 ). The tropical climate in Costa Rica is defined by the wet season from April to November and the dry season from December to April. The overall climate of Costa Rica is influenced by two types of winds. The trade winds from the NE present during the dry season and the equator winds from the west during the rainy season. The annual mean temperature is 20 °C and monthly precipitation is typically 85 mm during the dry months and 328 mm from April to November. The focus of the present paper is to document the diel and seasonal patterns of TGM at UNA in Heredia, Costa Rica 27 km from the Poas Volcano ( Martínez et al., 2000 ) in the Central Valley of Costa Rica and relate these values to temperature, solar radiation, wind speed and direction and compare these values to other sites. 2 Materials and methods 2.1 Sampling sites The longest and most detailed observations were made at the urban sampling point in Heredia at the facilities of the Laboratory for Atmospheric Chemistry (LAQAT) (10°00′00, 93″N and 84°06′34, 15″W). Samples were taken from May 2008 to early May 2009. Another sampling site was located on Pyroclasts Hill at Irazu Volcano (9°58′47, 87″N and 83°50′14, 43″W). This is located in the province of Cartago. Samples were taken from 3 to 9 March 2009. In the province of Alajuela, two sampling points were monitored. One was located in Poas Volcano National Park (10°11′45, 41″N and 84°13′50, 05″W). Another was located in the town of San Luis, Grecia (10°09′04, 8″N and 84°17′47, 3″W). It was monitored from October 31 to November 8, 2008. The above sampling points are located in rural areas. Sampling sites are shown in Fig. 1 . 2.2 Method of sampling and analysis Atmospheric air was sampled from a height of 2 m and fed to a total Hg vapor analyzer (Tekran 2537A). The flow rate of sampled air was 1.5 L min −1 and samples were collected over 5 min on the Au traps. Teflon filters (47 mm diameter, 0.2 μm pore size) were used at the inlet of the sample lines (unheated) to remove particulates. The two cartridges were packed with ultra high purity Au which has the ability to amalgamate Hg from the air sample. Once the amalgam is formed, it is thermally desorbed and detected using spectrophotometry by Cold Vapour Atomic Fluorescence (CVAFS). The detection limit is less than 0.1 ng m −3 and replication is within 5%. The instrument was equipped with an internal permeation source which allows automatic unattended recalibrations. 2.3 Meteorological data Temperature, wind speed, wind direction, radiation, and precipitation were obtained from the National Meteorological Institute (IMN) located on the Irazu Volcano and Juan Santamaría International Airport in Alajuela, about 11.3 km west from Heredia 09°59′North 84°10′West, altitude 913 m above sea level. Meteorological data collection in the volcanic areas was done by using a portable Campbell CR10 weather station equipped with an HMP155 air temperature and humidity probe, and a CNR4 Net Radiometer. 3 Results and discussion Soon after the instrument was installed the authors were surprised to observe values in excess of 50 ng m 3 but even more surprised when they reached a maximum of 905 ng m 3 ( Fig. 2 a). By chance, the work began soon after the onset of the rainy season. Suspecting that something was wrong the calibration was rechecked and local contamination sources were looked for without success. To ensure that there was no contamination deriving from the sample line it was replaced. The intake was extended so that samples would be collected far from the building. The first value was relatively low (15), as most of the sample came from the content of the line itself. With subsequent sampling, however, high values were again found and it was concluded that the values were reliable. Throughout the rest of the year and to May 2009 ( Fig. 2 a and b), values would occasionally reach 100 but were as low as 0.48 ng m 3 . The average was still quite high at 14.1 ng m 3 . Measurements made in February 2009 ( Fig. 2 b) were generally low but in May 2009 reached levels of over 100 ng m 3 . The onset of the rains was late that year and the extremely high values of the previous year were not observed. On a day when it was raining heavily in May 2009, a plastic sample collector was set out with a zip lock bag inserted inside. This was a make shift apparatus which illustrated that future work must be conducted with reliable estimates of wet deposition. Zip lock bags are known to be low in Hg so it was likely that the sample container was not contaminated. A wet deposition rate of 2 μg m −2 was obtained in one 24 h period. This should be placed in context with typical values for wet deposition in north temperate regions of about 10 or less μg Hg m −2 a −1 . Clearly, future work must include thorough measurements of wet and dry deposition rates. By the end of May 2008, values had declined to about 10–15 ng Hg m −3 and the equipment was taken to within 5 km of the volcano in Poas Volcano National Park. The average concentration obtained on 27 May was 583 ng Hg m −3 ( Table 1 ). The highest concentration measured was 807 ng Hg m −3 at 2:30 pm but by 3:45 the concentration was 403 ng Hg m −3 . No measurements were made at night in the park. Data were examined more closely and the patterns observed from days 138 to 143 were related to other environmental information. Strong diel patterns ( Fig. 3 ) were observed that were related to temperature, relative humidity, radiation and inversely with relative humidity. When the wind direction and velocity for this period were examined ( Fig. 4 a) it could be seen that the predominant air mass was from the NE direction. The highest values were recorded on day 141 on which there was almost no wind at all. Since the Poas volcano is located at about 300° but wind does come from that direction during the dry season. Additional data are provided in Table 1 . Values for San Luis and Grecia 10 km away from the Poas volcano are indeed elevated and much higher than at Heredia ( Fig. 2 a). The average daily concentration was 70 ng m −3 TGM ( Table 1 ). At the Irazu volcano, low values were recorded, with an average concentration of only 0.98 ng m −3 TGM. These values indicate that either not all volcanoes have the same capacity for emitting Hg, the plume may have been missed or measurements were made at the wrong time of the year. The remote sites would also not have the same level of oxidants in the atmosphere as at Heredia. A series of measurements were made at Alajuela ( Table 1 ) which is near the airport. The data were collected from 17 December 2008 to 25 January 2009 (days 351–25) ( Fig. 5 ). On 8 and 9 January, data were not recorded due to multiple power outages that occurred following a major earthquake at Cinchona on 8 January at 13:21 h. It had a magnitude of 6.2 on the Richter scale with an epicenter just 30 km from the sampling point. Following the earthquake, the concentrations were low ( Fig. 5 ). Earlier, on 21 Dec TGM concentrations ranged from 4.3 to 15.3 ng m −3 Hg with peak concentrations at midday. The average was 7.2 ng m −3 Hg. By 26 December values ranged from 1.7 to 8.4 but declined further to an average of 2.6 just before the earthquake. After the earthquake, values increased again so that by 12 January the concentrations were 2–3 ng m −3 Hg. On subsequent days TGM values were 0.44, 1.3, 1.6, 1.6, 1.3, 1.0 and 1.47 ng m −3 Hg. Clearly, the relationships between Hg emissions and tectonic activity are not yet obvious. This work comprises the first measurements of TGM in Costa Rica and those related to meteorological data relationships. There are many logistical problems in sampling volcanoes. The plume coming directly from the volcano is harmful to both instruments and people. Consequently, investigators are always faced with doing work at downstream locations where uncertainty of plume dispersion and direction is problematic. In addition, due to instabilities in the air mass, changes in temperature can result in mixing of tropospheric plumes resulting in a diel pattern that is not a true measure of emission patterns from the volcano itself. Nevertheless, this information provides new insights that may be useful for future investigations. It illustrates that very high values of TGM can be observed in air near volcanoes and suggests linkages to oxidants such as O 3 and H 2 O 2 . Measurements made by researchers at UNA have confirmed that levels are elevated in this region. As such, deposition rates of Hg from the atmosphere to soil and water may be elevated in areas where air pollution is elevated compared with non urban areas where levels are lower. This should be carefully investigated in further studies. Diel measurements of elemental Hg were also made by Tomiyasu et al. (2006) near the Sakurajima Volcano in Japan. They observed higher atmospheric Hg concentration during the daytime than nightime and showed a temperature dependency but their values were much lower (1.65–29.4 ng m −3 ) than were observed at UNA (up to 905 ng m −3 ). As in the present study, there was no relationship with wind direction. The day to night difference was greater at 3 m than at 12 m suggesting that these changes were driven by soil conditions. Elevated levels of Hg could potentially be influenced by both photochemical (uv radiation) and chemical reduction which gives rise to high levels of re-emission back to the atmosphere during the onset of the rainy season. The role of moisture due to the onset of the rainy season has only been discussed in a few papers previously. One paper ( Song and Van Heyst, 2005 ) showed that volatilization of Hg from soils increased when water was added to simulate a rain even. The experiments were conducted in controlled laboratory conditions. The soil was initially dry (5–6 vol.%) but volatilization of Hg was enhanced by 12–16 times after the soil moisture content was increased to >15 vol.%. If the soil was initially wet, no enhancement was observed. In other work, Poisant et al. (2004) used flux chambers to evaluate the emission of Hg 0 from bare and flooded wetland soils. They found that the principal mechanism controlling emission of H 0 from dry soil was diffusion and from wet soil it was due to photoreduction. Together, these studies support the influence soil moisture content has on volatilization rates. 4 Conclusions One hypothesis consistent with the observed concentrations is that Hg emitted from the volcanoes would become oxidized and then deposited to the soil during the dry season. This deposition may be influenced by atmospheric pollutants such as H 2 O 2 and O 3 . With the onset of the wet season, oxidized Hg (Hg 2+ ) may become reduced to Hg 0 in the presence of UV radiation and re-emitted into the atmosphere as Hg 0 resulting in high concentrations in ambient air. The highest concentrations of Hg were recorded during midday when radiation levels were highest. Measurements made at the Poas Volcano and San Luis confirmed that values were much higher near the volcano that at distance. It is unlikely that the elevated concentrations came directly from the volcano since one would not expect diel and seasonal patterns for volcanic emissions. In addition, the highest values were not observed when the winds were from the direction of the volcano. The exact mechanism(s) for the elevated concentrations cannot be confirmed at this time but future work should include estimates of flux rates from soils measured before, during and after the onset the rainy season. Techniques required have been outlined in Schroeder et al. (2005) . Either or both micrometeorological techniques and dynamic flux chambers could be used to determine flux rates with meteorological data as flux rates from soil and water are known to be related to temperature, wind velocity, humidity and Hg substrate level. While the contribution of volcanoes to the global budget is significant, the concentration of Hg in the air near the volcano may contribute to elevated levels of Hg in the food chain. The deposited oxidized Hg is potentially available for methylation. It is methyl Hg that is biomagnified in food chains leading to man and it is the form that crosses the blood brain and placental membranes. Methyl Hg is likely to be the most important form leading to toxicity in humans ( Clarkson, 2002 ). Acknowledgements We are grateful to M.Sc. Eliecer Duarte, Lic. Eric Fernadez, Lic. Sebastian Miranda from Observatorio Vulcanológico y Sismológico de Costa Rica (OVSICORI-UNA) for the support provided during sampling campaigns. We also thank M.Sc. Alfonso Liao for his help with meteorological data analysis. Organization of American States International Graduate Fellowship to Paleah Black, support from UNA and research grants to DL are acknowledged. References Barquero and Fernandez, 1990 J. Barquero E. Fernandez Volcanic eruptions and their consequences at Poa Volcano, Costa Rica Bol. Vokanol. 21 1990 13 18 (in Spanish) Boszke et al., 2003 L. Boszke A. Kowalski G. Glosinska R. Szarek J. Siepak Environmental factors affecting speciation of mercury in the bottom sediments Polish J. Environ. Stud. 12 2003 5 13 Clarkson, 2002 T. Clarkson Review: the three modern faces of mercury Environ. Health Perspect. 110 2002 11 23 Ferrara et al., 2000 R. Ferrara B. Mazzolai E. Lanzillotta E. Nucaro N. Pirrone Volcanoes as emission sources of atmospheric mercury in the Mediterranean basin Sci. Total Environ. 259 2000 115 121 Han et al., 2004 Y. Han M. Thomas L. Soon-Onn P. Hopke S. MukYi L. Wei J. Pagano L. Falanga M. Milligan C. Andolina Atmospheric gaseous mercury concentrations in New York State: relationships with meteorological data and other pollutants Atmos. Environ. 38 2004 6431 6446 Krug and Winstanley, 2004 E. Krug D. Winstanley Comparison of mercury in atmospheric deposition and in Illinois and USA soils Hydrol. Earth Syst. Sci. 8 2004 98 102 Li et al., 2008 C. Li L. Chunsheng J. Cornetta S. Williec J. Lamc Mercury in Arctic air: the long-term trend Sci. Total Environ. 407 2008 2756 2759 Lin and Pehkonen, 1997 C. Lin J. Pehkonen Aqueous free radical chemistry of mercury in the presence of iron oxides and ambient aerosol Atmos. Environ. 31 1997 4125 4137 Lindqvist et al., 1991 O. Lindqvist K. Johansson L. Bringmark M. Aastrup B. Timm A. Anderson Mercury in the Swedish environment recent research on causes, consequences and corrective methods Water Air Soil Pollut. 55 1991 1 261 Martínez et al., 2000 M. Martínez E. Fernández J. Valdés V. Barboza R. Van der Laat E. Duarte E. Malavassi L. Sandoval J. Barquero T. Marino Chemical evolution and volcanic activity of the active cráter lake of Poás volcano, Costa Rica 1993–1997 J. Volcanol. Geotherm. Res. 97 2000 127 141 Nriagu, 1989 J. Nriagu A global assessment of natural sources of atmospheric trace metals Nature 338 1989 47 49 Nriagu and Becker, 2003 J. Nriagu C. Becker Vocanic emissions of mercury to the atmosphere: global and regional inventories Sci. Total Environ. 304 2003 3 12 O’Driscoll et al., 2003 N. O’Driscoll S. Beauchamp S. Siciliano A. Rencz D. Lean Continuous analysis of dissolved gaseous mercury (DGM) and mercury flux in two freshwater lakes in Kejimkujik Park, Nova Scotia: examining flux models with quantitative data Environ. Sci. Technol. 37 2003 2226 2235 O’Driscoll et al., 2005 N. O’Driscoll A. Rencz D. Lean The biogeochemistry and fate of mercury in the environment A. Sigel H. Sigel R.K.O. Sigel Biogeochemical Cycles, Metal Ions in Biological Systems vol. 43 2005 Taylor & Frances Boca Raton, FL 231 238 (Chapter 9) Pacyna et al., 2006 E. Pacyna J. Pacyna F. Steenhuisen S. Wilson Global anthropogenic mercury emission inventory for 2000 Atmos. Environ. 40 2006 4048 4063 Pirrone et al., 1996 N. Pirrone G. Keeler J. Nriagu Regional differences in worldwide emissions of mercury to the atmosphere Atmos. Environ. 30 1996 2981 2987 Poisant et al., 2004 L. Poisant M. Pilote P. Constant C. Beauvais H. Zhang X. Xiaohong Mercury gas exchanges over selected bare soil and flooded sites in the bay St. Francois wetlands (Quebec, Canada) Atmos. Environ. 38 2004 4206 4214 Pyle and Matter, 2003 D. Pyle T. Matter The importance of volcanic emissions for the global atmospheric mercury cycle Atmos. Environ. 37 2003 5115 5124 Schroeder, 1996 Schroeder, W.H., 1996. Estimation of atmospheric input and evasion fluxes of mercury to and from the Great Lakes. In: Baeyens W., Ebinghaus, R., Vasiliev, O. (Eds.), Global and Regional Mercury Cycles: Sources, Fluxes and Mass Balances, pp. 109–121. Schroeder and Munthe, 1998 W.H. Schroeder J. Munthe Atmospheric mercury – an overview Atmos. Environ. 32 1998 809 822 Schroeder et al., 2005 W. Schroeder S. Beauchamp G. Edwards L. Poissant P. Rasmussen R. Tordon G. Dias J. Kemp W. Van Heyst C. Banic Gaseous mercury emissions from natural sources in Canadian landscapes J. Geophys. Res. 110 2005 1 13 Song and Van Heyst, 2005 X. Song W. Van Heyst Volatilization of mercury from soils in response to simulated precipitation Atmos. Environ. 39 2005 7494 7505 Steffen et al., 2008 A. Steffen T. Douglas M. Amyot P. Ariya K. Aspmo T. Berg J. Bottenheim S. Brooks F. Cobbett A. Dastoor A. Dommergue R. Ebinghaus C. Ferrari K. Gardfeldt M.E. Goodsite D. Lean A. Poulain C. Scherz H. Skov J. Sommar C. Temme A synthesis of atmospheric mercury depletion event chemistry in the atmosphere and snow Atmos. Chem. Phys. 8 2008 1445 1482 Sunderland and Mason, 2007 E.M. Sunderland R.P. Mason Human impacts on open ocean mercury concentrations Global Biogeochem. Cycles 21 2007 GB4022 10.1029/2006GB002876 Tomiyasu et al., 2006 T. Tomiyasu M. Eguchi H. Sakamoto K. Anazawa R. Imura Seasonal change and vertical movement of atmospheric mercury at Kagoshima city in relation with Sakurajima Volcano, Japan Geochem. J. 40 2006 253 263 UNEP, 2008 UNEP Chemicals Branch, 2008. The Global Atmospheric Mercury Assessment: Sources, Emissions and Transport. UNEP –Chemicals, Geneva. < http://www.chem.unep.ch/mercury/Atmsopheric_Emissions/UNEP >.
更多查看译文
关键词
seasonality,relative humidity,wind velocity,dry season,wet season,wind speed
AI 理解论文
溯源树
样例
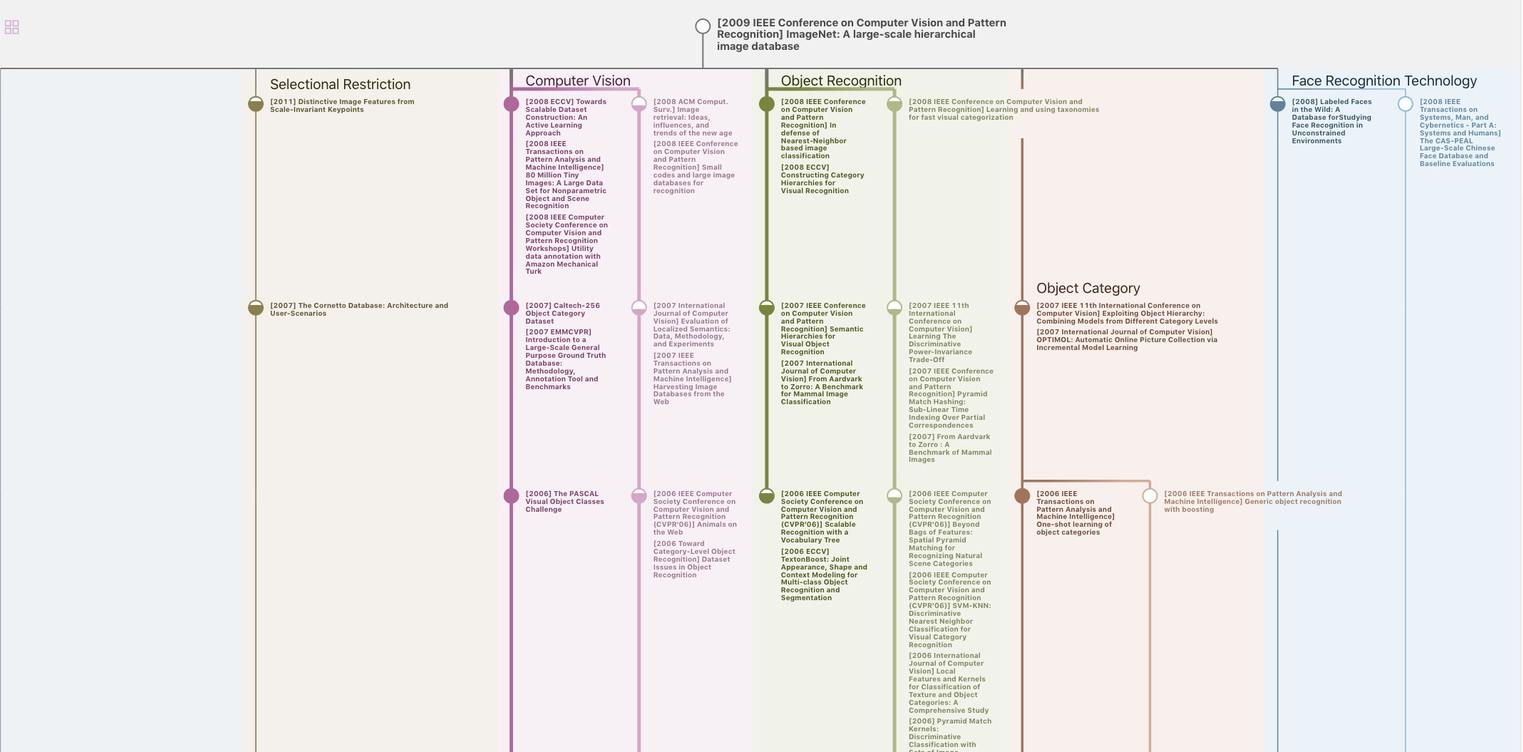
生成溯源树,研究论文发展脉络
Chat Paper
正在生成论文摘要