Carbon isotopes to constrain the origin and circulation pattern of groundwater in the north-western part of the Bohemian Cretaceous Basin (Czech Republic)
Applied Geochemistry(2010)
摘要
The Bohemian Cretaceous Basin represents a complex hydrogeological system composed of several aquifers with very favourable hydrogeological properties. These aquifers have been exploited for many years. The sustainability of such resources might be guaranteed by well organised water management, which requires a detailed knowledge about the functioning of the hydrogeological system. Although many efforts have previously been made to evaluate groundwater residence time, the many intricate geochemical processes complicate groundwater dating. The current study clarifies the functioning of this complex hydrogeological basin using hydrogeochemical and isotopic investigations. Chemical data and a combination of 13 C and 14 C isotopes within the Cenomanian and the Turonian layers indicate groundwater interactions with deep-seated CO 2 , rock matrix, surface waters and fossil organic matter. Very depleted δ 13 C values (average δ 13 C ∼ −13.4‰) suggest interactions with fossil organic matter, whereas enriched values account for the interaction with deep CO 2 gas ascending from the upper mantle via the numerous faults and fractures, and also, to a lesser extent, from calcite dissolution. Geochemical processes that take place in the system cause a clear depletion in 14 C that greatly complicates groundwater residence time evaluation. Different dilution correction models have been applied considering the different C origins. The stable isotope content, mainly 18 O values, indicates both the contribution of modern precipitation and the partial infiltration of palaeowaters during colder climatic conditions from the end of the Pleistocene. The apparent 14 C groundwater ages range from modern to 11.1 ka BP, which suggests some post glacial infiltration from melting ice sheets. Finally, all the acquired information was used to propose a conceptual model of C origin within the basin. 1 Introduction The Bohemian Cretaceous Basin (BCB) is the largest sedimentary complex of the Czech Republic. Because of its favourable hydrogeological conditions, it has been exploited for more than a century, most often for water supply and geothermal purposes. Nowadays, with the increasing exploitation of drinking and thermal waters, a new challenge appears, to protect the BCB groundwater. Groundwater in deep sedimentary formations represents an important strategic resource that often replaces conventional water supply from shallow reservoirs and/or from crystalline rocks following pollution or even catastrophic events in order to prevent disease epidemics and/or to guaranty the access of populations to safe drinking water supply. This was particularly true during the huge floods of 2002 in the whole of Central Europe ( Šilar, 2007 ). Deep aquifers with long residence time waters are also well protected from all negative climatic events leading to the decrease of infiltration (lower amount of precipitation, increase in temperature or/and evapotranspiration rate modification, etc.). Thus groundwater resources from deep sedimentary formations can guarantee the sustainable development of water supply ( Raoult et al., 1997, 1998; Edmunds et al., 2002; Jost, 2005; Douez, 2007; Celle-Jeanton et al., 2009 ); but, in many places, they should be better constrained in order to sustain them. Environmental isotope techniques have been applied in many regional and local investigations in order to understand the origin of water, the flow paths, the residence time and the geochemical processes within aquifers ( Marques et al., 1996; Edmunds and Smedley, 2000; Huneau and Blavoux, 2000; Birkle et al., 2001; Huneau et al., 2001; Edmunds et al., 2003; Edmunds, 2005; Carreira et al., 2008; Edmunds and Shand, 2008; Huneau and Travi, 2008; Coetsiers and Walraevens, 2009; Celle-Jeanton et al., 2009; Jiráková et al., 2009 ). Isotopic hydrogeology, with various approaches for groundwater dating notably by using C isotopes, has already been used in the Czech Republic ( Šilar, 1976, 1989; Bates et al., 2007; Pačes et al., 2008 ). The results of these studies showed that the majority of groundwater in the platform sediments of the whole Bohemian Massif are within the limits of radiocarbon dating and that some waters reveal both 3 H content and very low radiocarbon activity, indicating that complex interaction processes could be involved and could have lead to a modification of the original isotopic signature. This paper presents a global re-evaluation and synthesis of the data obtained from different studies with special emphasis on the origin of C isotopes in groundwater. At present, the simplest approach to radiocarbon dating of groundwater assumes that 14 C moves with the water molecules along the flow path and that the main mechanism enabling the change of the 14 C content is radioactive decay. In most situations this is not the case, because the signature may be diluted, particularly by 14 C-free sources ( Carreira et al., 2008 ). The determination of the main geochemical processes within the aquifers allowed introducing several dating techniques to estimate the apparent radiocarbon groundwater age. Knowledge of water origin is then essential to understand the geochemistry and the dynamics of the groundwater system which is fundamental to manage the sustainability of the exploitation of groundwater resources. 2 Study area 2.1 General setting The BCB is the most extensive continuous sedimentary basin of the platform cover of the Bohemian Massif. It extends over an area of about 14,600 km 2 , of which 12,490 km 2 lie within the territory of the Czech Republic ( Fig. 1 ). On the west, the basin crosses the border with Germany and extends towards the Dresden area. The study area covers approximately 6000 km 2 . The west and the north borders are formed by mountain ridges. The climate is continental humid with mean annual temperatures from 7 °C to 9 °C (data from 1961 to 1990; Czech Hydrometeorological Institute, 2009 ). The temperatures are controlled mainly by altitude differences which range between 100 and 700 m a.s.l. for the majority of study area. The annual precipitation has a zonal character ranging from 500 mm in the south up to 1000 mm in the north (data from 1961 to 1990; Czech Hydrometeorological Institute, 2009 ). The recharge of the Cenomanian and Turonian aquifers occurs mainly in the north-eastern part of the study area which corresponds to a mountainous area. The infiltrated water follows the hydraulic gradient and flows towards the Labe River which represents the main drainage axis of the basin. 2.2 Geological setting and tectonics The BCB is an intra-continental basin formed as a seaway between the North Sea Basin and the Tethys Ocean. A simplified geology with major structural characteristics is displayed in Figs. 1 and 2 . The basement is formed by partially metamorphosed Proterozoic and Palaeozoic rocks, locally pierced by intrusive rocks. The occurrence of volcanic rocks is very scattered throughout the north-western area. The basement is in some places covered with Permo-Carboniferous beds of both sediments (claystones, siltstones, greywackes, arkoses, conglomerates) and volcanic rocks ( Klein, 1979 ). This geological succession ( Fig. 3 ) extends mainly over the south with a few occurrences in the north ( Krásný, 1973; Fediuk, 1996 ). Some of the Permo-Carboniferous sediments may contain fossil organic matter, particularly black coal deposits identified mainly in the south-eastern part of the study zone, with a maximum thickness of several hundreds of meters SW from Mladá Boleslav ( Jetel, 1982 ). Permo-Carboniferous sediments do not outcrop in the study zone, they are only found to the NE of the study area. During the Variscan, major folding and metamorphic events occurred. The BCB was formed by the reactivation of a fault system in the Variscan basement of the Bohemian Massif during the mid-Cretaceous ( Uličný, 1997, 2001 ). The sediments accumulated from the Early Cenomanian or even from the Late Albian to the Santonian. The Upper Cretaceous sediments in this region show a particular dominance of sandstones, locally very rich in quartz (up to 99%) over other rock types. Six lithostratigraphic units can be distinguished, from the oldest to the youngest respectively: Late Albian, Cenomanian (marine deposits), Lower Turonian, Middle Turonian, Upper Turonian, Coniacian and Santonian. Čech et al. (2005) observed abundant plant debris in the marine Cenomanian beds and Kolářová and Krásný (1972) confirmed the occasional occurrence of carbonized wood and plant detritus within the marine Cenomanian sediments. Similarly, on the basis of well-logging measurements, Datel et al. (2009) identified some sandstone beds within the Cenomanian aquifer containing fossil organic material. According to Pačes et al. (2008) , siltstones and clays in the Cenomanian locally contain up to 1% fossil organic C. The thickness of the sedimentary formation in the BCB typically ranges between 200 and 400 m, but in the vicinity of the city of Děčín reaches up to 1200 m. Tertiary sediments and frequent small occurrences of intrusive rocks forming volcanic hills and mountains markedly affect the surface. The sedimentary succession in this area is considerably affected by fault structures of local and regional importance. The Saxonic block-fault tectonics has substantially influenced the development of the Bohemian Massif after the end of the Variscan orogenesis, when the Bohemian Massif became a rising consolidated block ( Malkovský, 1976 ). Numerous faults are still important. The principle directions of displacement zones are SW–NE (Erzgebirge) and NW–SE (Sudetic). The Ohře (Eger) rift, active from the Oligocene to the Pleistocene ( Kopecký, 1978 ), is the most dominant structure in western Bohemia and strikes the study area only with its marginal faults (Krušné Hory and Litoměřice fault). Many distinct volcanic features are defined within the Ohře (Eger) rift, but the volcanic activity in the Labe (Elbe) zone is less obvious. The intersection of the zone with the Ohře (Eger) rift coincides with the Pliocene neovolcanic event made up of an extrusion of nephelinite basanite to olivine basalt. The maximum tectonic vertical movement took place along the Krušné Hory fault. The total throw is estimated to be about 800–1500 m. Ductile deformation of the Cretaceous sediments (folds) is present across the whole basin with fold structures with a W–E direction formed in the initial phases of the Saxonian tectogenesis and that are not continuous over the whole area. The folds were sometimes displaced by faulting during subsequent deformational phases ( Malkovský, 1976; Herčík et al., 1999 ). 2.3 Hydrogeological setting The BCB has been extensively exploited for water supply since the 1930s as a consequence of the favourable hydraulic parameters and high quality of groundwater. Groundwater has already been investigated by various researchers ( Hynie, 1949, 1961; Hercog, 1965; Kliner and Kněžek, 1974; Jetel and Krásný, 1976; Herčík et al., 1999; Bates et al., 2007 ) confirming that this part of the BCB is one of the most complex hydrogeological structures of the entire basin. Its complexity lies in the laterally and vertically unstable lithofacies evolution of the Cretaceous cover. The whole structure was slightly folded and broken into numerous blocks displaced hundreds of meters inducing a very complicated hydrogeological regime. The most significant groundwater resources belong to the central structure which is formed by the lowered block. The organisation of aquifers is mainly subhorizontal and differs according to their position within the study area. Fig. 2 shows the aquifer organisation in a selected cross-section. Within the BCB the following succession of aquifer layers can be found: (1) Basal Aquifer A in the Cenomanian (occasionally connected to the Lower Turonian forming AB aquifer). (2) Aquifer B in the Lower Turonian. (3) Aquifer C in the Middle Turonian. (4) Aquifer D in the Upper Turonian–Coniacian–Santonian. The basal Aquifer A is formed by the Cenomanian sandstones and is about 50 m thick, with sporadic claystone layers. It is generally confined and present throughout the whole area. Transmissivity, as a function of the intergranular porosity and numerous fractures ( Jetel and Rybářová, 1991 ) is about 64 m 2 /day; mean porosity has been determined to be about 20% ( Herčík et al., 1999 ). Aquifer A forms an extended flux field asymmetrically divided by the Labe (Elbe) River into two sections. The dynamics of groundwater circulation in the Cenomanian aquifer are controlled by the piezometric level of the recharge area in the north and the height above sea level of the discharge areas along the drainage of local rivers – Labe (Elbe), Kamenice and Ohře (Eger). The average effective velocity of groundwater flow varies between 0.06 and 0.6 m/a ( Jetel and Rybářová, 1991 ). The Cenomanian aquifer is generally well separated from the overlying aquifer by the marly layer of the Lower Turonian. This impermeable layer is absent only in the south-western part. There, the Cenomanian and the Turonian aquifer form a continuous formation (AB). The Lower–Middle Turonian aquifers (C, BC) with an average thickness of about 150 m are developed mostly of siltstones and sandstones in an area on the right bank of the Labe (Elbe) River, where they host the largest resources of groundwater in the whole Cretaceous Basin. Groundwater of the Turonian aquifer is unconfined over almost the whole western part of the BCB. Confined levels are only found in the area where the Turonian sandstones are overlain by the Upper Turonian aquitard. The permeability is both of intergranular and fissured types and generally decreases towards the SE due to the lithofacies development of the entire Cretaceous complex. The highest average transmissivity coefficient in the aquifer has been documented on the northern margin with a value of 762 m 2 /day, while the lowest values (about 48 m 2 /day) were encountered in the southern part ( Herčík et al., 1999 ). On the east, the flow direction is influenced by the drainage effect of the Jizera River. In general, the groundwater of aquifer C (or BC) is drained by the surface watercourses. From the water management point of view, the Turonian aquifer plays the major role with its significant thickness and favourable hydrogeological parameters. The general flow direction in the study area is globally similar for Cenomanian and Turonian aquifer as shown by the piezometric data collected between 1985 and 1987 ( Herčík et al., 1999 ). Although the tectonics has some consequences on the piezometric level, the effect of faults on the regional groundwater flow direction is insignificant ( Šilar, 1976; Herčík et al., 1999 ). The general flow direction is schematized in Fig. 2 . The uppermost aquifer in Coniacian–Santonian (D) is composed of sandstones which discontinuously crop out in the central part of the area of interest. The permeability is of combined intergranular and fissured types. Aquifer D is not generally of high hydrogeological significance. It is relevant to mention, that some spas are located in the study area, or in its environs. Teplice, on the west, is among the most famous and the discovery of its warm springs goes back to 762 AD. The coal mining activities in the region have affected the hydraulic properties of the spring and consequently its flow rate has decreased considerably ( Čadek, 1968 ). The water of Teplice originates from a Palaeozoic porphyry and is hydraulically connected with the surface water bodies of the Tertiary aquifers. 3 Database setup and methodology This paper provides a review of studies carried out in the north-western part of the BCB ( Šilar, 1976 , personal communication; Pačes et al., 2008 ); the dataset of 22 samples is introduced in Table 1 . The most extensive isotopic database for the Czech massif has been provided by Šilar (1976) , nevertheless only two samples are located within the research area. For these, the Total Dissolved Inorganic C (TDIC) was collected in the field in the form of a precipitate of BaCO 3 . The samples were analysed in the laboratory of the International Atomic Energy Agency, Vienna, by β counting. Most of the analyses in Table 1 come from an unpublished dataset acquired by the same author during the years 1972–2002 (Silar, personal communication). The majority of the samples belong to the Turonian and the Cenomanian aquifers. Eight more measurements from the eastern part of the study area come from the study of Pačes et al. (2008) ; including three Turonian and five Cenomanian samples. Besides the isotopic signature ( 13 C, 14 C, 3 H, 18 O, if available), Table 1 gives an information on coordinates (S-JTSK / Křovák coordinate system, adapted for the territory of former Czechoslovakia), depth and reached aquifer. Oxygen-18 content of the water samples are reported in ‰ vs. Standard Mean of Ocean Water (SMOW), radiocarbon as percent of modern C (pmC) and δ 13 C is expressed with respect to Pee Dee Belemnite (PDB). The spatial distribution of the investigated 22 wells is displayed in Fig. 3 . The isotopic dataset obtained rarely contains complete information on groundwater geochemistry and this led the authors to set up a new dataset on chemistry. Some chemical analyses have been collected from the studies of Šilar (1976) and Pačes et al. (2008) which accompany the isotopic information described above. To add more information on the chemistry of the different aquifers, analyses were gathered from the archive of the Czech Geological Survey ČGS-Geofond in Prague including local and regional research studies ( Žitný, 1970; Čapek, 1979; Brožek, 1987; Skořepa, 1993 ). In total 47 chemical analyses were considered ( Table 2 ) to construct a Piper diagram displaying the different water types. The main objective of the study is to understand the geochemical processes occurring within the aquifers during groundwater flow leading to modifications of the chemical and isotopic composition. First, the information on chemistry is considered, to determine or identify the processes occurring between rocks and groundwater. Then, the isotopic signature of waters is considered in order to determine the recharge conditions and to establish groundwater origin. For this purpose, C isotopes are used in particular giving evidences of the different geochemical processes taking place within the aquifers. The second goal of this work is to propose a conceptual model of the hydrogeology of the BCB. Carbon isotopes will be mainly considered as tracers to identify C origin in the groundwater system. In addition, an attempt to date groundwater will be made for most samples. 4 Results 4.1 Chemistry The chemistry of groundwater is an indicator of the hydrodynamic and geochemical processes taking place within the aquifers. It reflects the flow conditions of groundwater, the interconnection of individual aquifers and often expresses the hydrodynamic role of tectonics and the dominance of certain geochemical processes in the evolution of the chemistry of fresh and mineral waters ( Kolářová and Krásný, 1972 ). Generally pH values lie in the range between 6.5 and 7.9 indicating that most of the TDIC occurs in the form of HCO 3 . The maximum temperature in the Cretaceous formation reaches 33.5 °C in a 511 m deep well (No. 13). The Teplice waters from the Palaeozoic range between 36 °C and 41.5 °C. The mineralisation of these thermal waters slightly exceeds 1000 mg/L. The mean Total Dissolved Solids (TDS) of the Turonian aquifer (371 mg/L) is lower than for Cenomanian samples which are around 500 mg/L ( Pačes, 1974 ). Elevated TDS values in the Turonian aquifer were explained by Kolářová and Krásný (1972) as being the result of significant ion-exchange processes particularly in clayey, poorly permeable Turonian rocks. The dataset contains two Palaeozoic samples, which correspond to the thermal Teplice water, and 24 Cenomanian and 21 Turonian waters. The analyses are displayed in a Piper diagram ( Fig. 4 ). On the whole, the diagram highlights three waters types: Na–HCO 3 , Ca–HCO 3 and Ca–SO 4 . However water composition in the aquifers is often in a transient state resulting from various processes operating at different timescales ( Andersen et al., 2005 ). Two Palaeozoic samples representing thermal waters in Teplice ( T ∼ 40 °C) reveal a Na–HCO 3 type of water. Considering the crystalline geological context of this area, the significant content of Na + and HCO 3 - ions may result from the weathering and dissolution of silicates such as feldspar from granitic rocks ( Appelo and Postma, 2005 ). The majority of the Turonian and Cenomanian samples are of Ca–HCO 3 type probably as a consequence of calcite dissolution. They were generally sampled on the right bank of the Labe (Elbe) River. As mentioned, the aquifers do not generally contain carbonate rocks ( Herčík et al., 1999 ), but Ca 2+ and HCO 3 - ions might be produced by the hydrolysis of the calcite cement of the sandstone ( Pačes et al., 2008 ) or in a very limited way by feldspar dissolution in basement rocks. Some waters tend to be slightly enriched in SO 4 2 - and Cl − . The Cl − and SO 4 2 - content of the Cenomanian waters reaches up to 77 mg/L and 230 mg/L, respectively ( Table 2 ). This may be due to the influence of a local accumulation of connate waters in the basement of the Permo-Carboniferous sediments underlying the Cenomanian aquifer. This is confirmed by the correlations between Cl − and Na + ( Jetel, 1970 ). As a consequence, the Ca–HCO 3 water type gradually transforms towards a Na–Ca–HCO 3 water type or Na–Ca–HCO 3 –Cl–SO 4 water type. This tendency might be explained by the aquifer freshening theory as already observed by several authors in such a sedimentary context ( Appelo and Postma, 2005; Andersen et al., 2005 ). During the aquifer freshening, the mineralised waters are progressively flushed away from aquifers and replaced by freshly infiltrated waters that, therefore, modify the original chemical signature of the aquifer waters. In some places, the elevated SO 4 2 - concentrations (up to 472 mg/L) could be caused by gypsum dissolution as proposed by Pačes et al. (2008) . Cenomanian waters sampled on the left bank of the Labe (Elbe) River, in the proximity of Děčín, Ústí nad Labem and Teplice, plot in the Na–HCO 3 water type zone, with temperatures between 25 °C and 34 °C and a composition similar to the Teplice thermal waters. Here, the fractures of the Palaeozoic and Proterozoic metamorphic rocks, constituting the aquifer basement and outcropping in Teplice, probably enable groundwater interconnection with the Cretaceous sandstone aquifer ( Pačes, 1974 ). Such a link may explain the comparable chemical type of the Cenomanian and the pre-Cretaceous waters; with a chemical composition mainly influenced by the processes occurring within the aquifer basement. A gradual substitution of Na + by Ca 2+ ions occurs along the flow trajectory and may also be caused by aquifer freshening or by isomorphic ion substitution. This exchange could take place by reaction with clay minerals in the matrix of sandstones. Čadek (1968) and Pačes (1974) also suggested that dissolved CO 2 from deep sources hydrates and dissociates to HCO 3 - ions which are exchanged for Na + in feldspars. As also observed by Bates et al. (2007) , the Turonian aquifer has lower amounts of Na + and SO 4 2 - than the Cenomanian aquifer. The investigation of the chemical composition of the BCB groundwater confirms an evolution during the groundwater flow. Several geochemical processes might be observed as displayed in Fig. 4 . Cenomanian samples reveal two different water types, which are clearly separated by the Labe (Elbe) River. On its left bank, the water is enriched in Na + ions, while on its right bank (majority of samples) the chemical composition is rather of Ca–HCO 3 water type. This phenomenon is probably related to the presence of a deep tectonic structure following the Labe (Elbe) River course. Turonian samples do not reveal important differences according to their geographical location. A few samples seem to be influenced by gypsum dissolution, but most of them are of Ca–HCO 3 water type. 4.2 Modern water indicators The identification of a modern water contribution to the aquifer is essential to give a reasonable age to the groundwater ( Jiráková et al., 2009 ). This phenomenon is reliably traced by NO 3 - and 3 H concentrations which are the products of modern anthropogenic activities. Nitrates are usually found in the agricultural areas and only in shallow layers, because they easily undergo reduction during their transfer to depth. Nitrate concentration rarely exceeds 5 mg/L. Nevertheless, according Table 2 , maximum NO 3 - concentrations of 31 mg/L and 22.2 mg/L were measured in the Cenomanian and the Turonian aquifer, respectively, which is doubtless due to the strongly developed agricultural activities along the Jizera River Valley. In deep aquifers, 3 H appears to be a reliable tracer of modern waters. Most of this radioactive H isotope reached the groundwater system during the period of thermonuclear bomb testing ( Clark and Fritz, 1999 ). Presently, only a small quantity of 3 H is observed in precipitation. According to IAEA/WMO (2006) , the values recently measured in Vienna range between 5 and 10 TU in modern local precipitation. A certain amount of 3 H was observed in the aquifers of the BCB. As the analyses considered in this study were not carried out within the same time span for all the samples, the 3 H contents provided are recalculated for the year 2009 ( Table 1 ). Table 1 shows that in some places groundwater in the BCB still contains 3 H at the present time. The Quaternary spring of Ústí (No. 3) contains 6.8 TU that confirms the modern origin of water. The recalculated 3 H content in most of the Turonian samples for the year 2009 ranges from 3.2 to 5.9 TU. Cenomanian waters generally contain very low quantities of 3 H, close to 0 TU. Only a few Cenomanian samples have a content around 6 TU (No. 11, No. 13 and No. 17). These values support the hypothesis that groundwater from the BCB may be partially of modern origin, but only to a very limited proportion. Tritium in deep aquifers may be caused by the dense tectonics in the region that may facilitate the diffusion of modern water components towards the deep reservoirs. Additionally, contamination by modern water may be caused by leakage and short circuiting along the well casing and this is particularly likely for old boreholes ( Appelo and Postma, 2005 ). The presence of 3 H in waters of presumed deep/confined origin was extensively discussed in Carreira et al. (2008) who described this phenomenon in Portuguese CO 2 -rich mineral waters. In particular rock environments, small amounts of 3 H may be produced naturally, however, the natural production results in measurable but generally very low values which do not exceed 0.1 TU ( Clark and Fritz, 1999 ). The 3 H values measured in the BCB aquifers generally exceed this limit. So, the majority of the Turonian and few Cenomanian samples reveal an influence of modern water infiltration. However, the concentration of 3 H for many samples is inconsistent with the low radiocarbon activities measured, suggesting on the contrary a long residence time of groundwater. This inconsistency will be emphasised and discussed later. Nevertheless, the 3 H results suggest the mixing of modern water with an older groundwater component and therefore have to be considered during the groundwater dating. 4.3 Stable isotopes of water Stable isotopes of water are commonly used to identify groundwater origin, the recharge areas and the underground flow paths. It was established that groundwater from the sedimentary rocks of the Czech Massif is generally of meteoric origin and that they have not been subjected to surface evaporation ( Pačes et al., 2001; Pačes and Šmejkal, 2004; Frape et al., 2007 ). In the study area, nine determinations of δ 18 O have been made which reveal values varying from −11.2‰ to −8.6‰ ( Table 1 ). Two of them (No. 1 and No. 2) originate from the Teplice thermal waters in the Palaeozoic metamorphic rocks and have values of −9.4‰ and −9.8‰, respectively. Although there is no meteorological station in the Czech Republic regularly measuring the isotopic signature of precipitation, the data of the Leipzig station ( IAEA/WMO, 2006 ) has been used, which is close to the study area and has very similar climatic and altitude conditions. The weighted mean annual isotopic content of Leipzig rainwater is −8.63‰ δ 18 O. This value is consistent with the study of Noseck et al. (2009) carried out in a region very close to the Ruprechtov site. The δ 18 O values of groundwaters are depleted compared to meteoric waters. Cenomanian samples No. 11, No. 13 and No. 17 are very depleted in 18 O (from −10.1‰ to −10.7‰). Samples No. 4 and No. 5, that belong to the Turonian aquifer, are the most depleted of the dataset (−11.2‰ and −10.9‰) but it should be noted that the stratigraphical identification is very ambiguous in this area. In fact, the Cenomanian and Turonian aquifers evidently communicate mainly due to the very active and large Děčín fault system which enables vertical groundwater flows. Therefore samples No. 4 and No. 5 could possibly represent the signature of Cenomanian waters. Only the Turonian samples No. 6 and No. 7 differ significantly from all the Cenomanian samples and reveal values (−8.6‰ and −8.9‰, respectively) close to modern infiltration. The significant depletion in stable isotopes observed for Cenomanian samples may be explained by several phenomena. First, the altitude effect could be responsible for depleted values considering that the recharge area of both aquifers is located close to a mountainous system. Secondly, the Pleistocene continental glaciation could be significant ( Šilar, 2007 ) as the glacial limit of the Fennoscandian Ice Sheet reached the north borders of Czech territory ( Ehlers and Gibbard, 2004; Toucanne et al., 2009 ). Precipitation during the glacial ages was characterised by depleted isotopic values as the result of the low temperature effect ( Clark and Fritz, 1999 ). The depleted values in stable isotopes observed in Cenomanian samples therefore suggest recharge during colder climatic conditions. Nevertheless, several authors have shown that during cold periods, precipitation did not reach the groundwater system ( Darling, 2004; Douez, 2007 ). It is supposed that the periglacial climate and the permafrost conditions were adverse for groundwater recharge and a recharge gap during the Last Glacial Maximum (LGM) has been proposed. As the ice sheet represents an archive of the palaeoglacial climate, their thaw at the end of the Pleistocene period might have led to the infiltration of depleted waters. Indeed, after the LGM, the water from the melted north European glacier was preferentially drained by the existing large basins such as the Wesser, the Ems and the Labe (Elbe) ( Toucanne et al., 2009 ) that were, for that reason, charged by waters with a depleted isotopic signature. 4.4 Carbon isotopes in the context of the BCB As demonstrated by Fontes (1985), Edmunds and Smedley (2000), Carreira et al. (2008), Jiráková et al. (2009) , many problems have to be solved for a reliable interpretation of C geochemistry. Numerous difficulties can be met within aquifers exposed to several interactions leading to variations in the C isotopic signature. In the studied aquifers, account has been taken of different potential sources of C. It is, therefore, essential to define the isotopic signature of all potential end members in order to establish their degrees of interaction with the groundwater. 4.4.1 Geogenic carbon First, the isotopic signature of rock calcite should be defined. The δ 13 C values of carbonates from Upper Cenomanian and the Turonian formations have been measured in the very similar geological environment of the western BCB in the Dresden area ( Voigt and Hilbrecht, 1997 ). Calcareous sandstones from the Upper Cenomanian show a minimum value of δ 13 C ∼ 1.7‰ which gradually increases and reaches a maximum in the uppermost Cenomanian layer (δ 13 C ∼ 4.6‰) reflecting an enrichment in δ 13 C at the Cenomanian–Turonian boundary event ( Voigt and Hilbrecht, 1997 ). In the Lower Turonian, the values steadily decrease. Middle and Upper Turonian show δ 13 C values in the range of 1–2.1‰. Upper Turonian values are documented also in Weise et al. (2004) who observed values of the same order. Carbon from Cretaceous carbonate rocks is 14 C free ( Clark and Fritz, 1999 ). The second end member with low radiocarbon activities and enriched 13 C values is the CO 2 (gas) of upper mantle origin. Numerous isotopic investigations of gases carried out in the western part of Ohře (Eger) rift have identified CO 2 gas from deep-seated sources with δ 13 C values ranging between −1.8‰ and −3.2‰ ( Pačes, 1974, 1987; Weinlich et al., 1998, 1999, 2003; Pačes et al., 2001; Geissler et al., 2005 ). The radiocarbon activity for geogenic CO 2 (g) is usually around 0 pmC. Near the study area Šilar (1976) measured a value of about 2.4 pmC in the Cheb Basin. 4.4.2 Atmospheric carbon Another input to the system is defined by modern atmospheric CO 2 , which is generally about 100 pmC for 14 C ( Clark and Fritz, 1999 ). The quantity of 13 C in the atmosphere is now decreasing as the result of the industrial revolution and the use of fossil C with depleted 13 C values ( Clark and Fritz, 1999 ). Studies carried out between 1977 and 1992 ( Levin et al., 1995 ), indicated an atmospheric CO 2 signal between −7.8‰ and −8.22‰ as a consequence of atmospheric C dilution, although studies performed by other authors give a mean δ 13 C atmospheric value of −7.5‰ ( Mook, 2000 ). 4.4.3 Soil and vegetation carbon Soil atmosphere and vegetation are characterised by a value of 100 pmC for 14 C ( Clark and Fritz, 1999 ). Since the Czech Republic has C3 cycle characteristics in term of vegetation type. The δ 13 C content in plants ranges between −24‰ and −30‰ with an average of about −27‰ ( Vogel, 1993 ). This is in agreement with the finding of Novák et al. (2003) who carried out soil measurements at several European sites and, in the western part of the Czech Republic at Načetín site, recorded a value of δ 13 C around −27‰ for the soil organic matter. The δ 13 C of soil CO 2 in most C3 ecosystems is generally enriched (δ 13 C ∼ −23‰, Deines et al., 1974 ). As already mentioned, there is evidence of the presence of fossil organic matter in the Cretaceous sediments of the region. Thus, it is relevant to define the fossil organic C isotopic composition since CO 2 can also be produced from the decay of organic matter. The δ 13 C of fossil organic matter is usually between −30‰ and −20‰ ( Truesdell and Hulston, 1980; Weinlich et al., 1999; Bergfeld et al., 2001 ). Martínek et al. (2006) carried out a palaeoenvironmental study in the mountainous region of northern Bohemia and most often values obtained were in the range of −25‰ to −27‰ for δ 13 C in fossil organic matter from the lower Permian layers. Several measurements have been carried out on coal in Central Europe with δ 13 C values being about −23.5‰ for Carboniferous coals from the Polish Silesian Basin, −24.4‰ for the Saar coals and −23.9‰ for the Aachen coals ( Rice and Kotarba, 1993 ). Fossil organic matter is also 14 C free ( Clark and Fritz, 1999 ). 5 Discussion 5.1 Identification of carbon origins within the BCB The study of C isotopes ( 13 C and 14 C) not only provides information about residence time but also information about the C origin. As a consequence, radiocarbon can even be considered in some contexts as a pertinent tracer of groundwater flow and evolution ( Rose and Davisson, 1996; Rose et al., 1996 ). The investigated water samples are reported in a graph showing 13 C and 14 C evolution ( Fig. 5 ). It appears, that the points are scattered among the different end members described previously. The δ 13 C analyses carried out on the TDIC range from −16.3‰ to −4.5‰ clearly suggesting a mixture of C from different sources. The radiocarbon activities cover the entire interval between radiocarbon free sources and sources of modern origin. To identify the mixing processes within the aquifers, consideration needs to be given not only to the C isotopic signature but also to the overall geochemistry of the aquifers and the geographical position of sampling sites, notably the relationship to the tectonics. Water No. 3 sampled near Ústí nad Labem corresponds to a Quaternary spring (δ 13 C ∼ −14.1‰, 14 C ∼ 89.89 pmC) and gives a reasonable idea of the pristine isotopic composition of newly infiltrated waters. The enriched δ 13 C signature of most waters tends to indicate that the majority of samples are variably influenced by CO 2 from the upper mantle characterised by a δ 13 C signature of about −2.7‰ and deprived of any 14 C. This phenomenon was also noticed along the Ohře (Eger) Rift SW from the study area in the vicinity of the Czech spa region ( Weinlich et al., 1998; Geissler et al., 2005 ). The origin of the CO 2 in many of the CO 2 -rich mineral waters from western Bohemia has been investigated by numerous authors; the CO 2 originates from magmatic sources, but the water molecule isotopes always reveal a meteoric origin for the water ( Šilar, 1976; Hladíková et al., 1987; Pačes et al., 2001; Pačes and Šmejkal, 2004 ). Other studies have been carried out in the Poděbrady spa region ( Kolářová and Krásný, 1972; Jetel and Rybářová, 1991 ) located in the eastern proximity of the study area. These studies result in the identification of a CO 2 -rich mineral water accumulation and suppose that the gas ascends through the deep fault structures. In the dataset, samples No. 4, No. 5, Nos. 11–18, No. 21 and No. 22 have undergone isotopic exchanges with C from mantle gases. In addition, this is supported by the position of these samples in Fig. 5 , which are distinguished by low 14 C activities and 13 C values close to the deep CO 2 (g) end member. Samples No. 4 and No. 5 ( 14 C ∼ 10.93 pmC and 5.35 pmC) come from the Turonian layer and interaction with deep CO 2 is not very common for this shallow aquifers. Both these samples were taken from the Děčín area where tectonics markedly affects the surface. Moreover, it has already been mentioned, that the stratigraphy is very ambiguous in the west of the BCB as the Turonian layer can only be distinguished with difficulty and is even often missing. Then, the isotopic signature of No. 4 and No. 5 may possibly represent the Cenomanian waters as confirmed by the δ 18 O‰ depleted value. Waters No. 11 to No. 16 ( 14 C in the range from 4.75 pmC to 12.16 pmC) come from the vicinity of Ústí nad Labem, which is in the north part of the BCB, corresponding to a tectonically rich area so explaining their low radiocarbon content. Cenomanian waters No. 17, No. 18, No. 21 and No. 22 ( 14 C ranges from 14.2 pmC to 24.57 pmC) were sampled in the proximity of the fault on the left bank of the Jizera River and near the Lužice fault, respectively. Turonian samples No. 6 and No. 7 are located on the fault zone of Litoměřice, but the C isotope record ( 14 C ∼ 33.94 pmC and 38.95 pmC) does not suggest any evident interaction of Turonian waters with deep CO 2 . These samples tend to reveal another phenomenon observed from the δ 13 C– 14 C scatter in Fig. 5 and accounting for groundwater–rock interactions. In the BCB, aquifers generally have low contents of carbonates, although there are some insignificant calcareous sandstone, siltstone, marlstone and claystone layers within the Turonian strata ( Kolářová and Krásný, 1972; Datel et al., 2009 ), but it should be noted that calcite cement is present in most Cretaceous sandstones in Bohemia. Fig. 6 shows an increase of HCO 3 - values accompanied by an enrichment in δ 13 C content, suggesting that some calcite dissolution processes must take place in the aquifers, which is probably due to the hydrolysis of calcite cement ( Coetsiers and Walraevens, 2009 ). However, this relationship is ambiguous, as increasing content in HCO 3 - may be the result of more than one process (e.g. reaction with the organic matter). Additionally, a third interaction involving mainly samples No. 16, No. 17, No. 21 and No. 22 can be noted in Fig. 5 . Having depleted 14 C activities (from 9.62 pmC to 24.57 pmC), these samples are characterised also by very depleted 13 C values (from −16.3‰ to −10.2‰) which can not be explained only by a mantle CO 2 contribution or calcite dissolution. Such a tendency is in agreement with reactions with C from fossil organic origin with a δ 13 C value comparable to the one of organic C ( Van Der Kemp et al., 2000 ) but with 0 pmC content of radiocarbon. Negative values between −17.1‰ and −25.5‰ were also measured by Pačes et al. (2001) in some groundwater samples in the mountainous region SW from the study area and they were attributed to CO 2 generated by the decomposition of organic matter. As discussed earlier, fossil organic matter occurs within the region mainly in the Permo-Carboniferous layers but to a lesser extent can be also found in the Cenomanian strata ( Kolářová and Krásný, 1972; Jetel, 1982; Pačes et al., 2001; Martínek et al., 2006; Datel et al., 2009 ). The waters most depleted in δ 13 C are No. 17, No. 21 and No. 22 sampled on the left bank of the Jizera River and No. 16 originating from the northern area near the Kamenice River. These samples come from the area were the occurrence of Permo-Carboniferous layers is well documented ( Krásný, 1973; Malkovský, 1976; Fediuk, 1996 ). Moreover, the vicinity of the fault zone can facilitate the distribution of groundwater, which had been in contact with the Permo-Carboniferous. It is also relevant to note that the southernmost part of the study area is famous for its very thick Permo-Carboniferous layers rich in coal deposits ( Jetel, 1982 ). Similar results associating depleted δ 13 C and low radiocarbon content have already been observed in the nearby region of eastern Bavaria by Weinlich et al. (1999) and Geissler et al. (2005) . The radiocarbon content observed in the Shönbrunn mine was around 5.9 pmC associated with very depleted CO 2 (g) (δ 13 C ∼ −17.4‰). However, no observed clear and direct interaction between fossil organic matter and surface waters is observed. As shown in Fig. 5 , samples which are markedly influenced by reaction with fossil organic matter in the BCB (No. 16, No. 17, No. 21 and No. 22) seem to be in a transitional state. The more the samples are enriched in 14 C, the more they are depleted in δ 13 C values. This phenomenon is difficult to explain as several processes are involved. 5.2 Approach towards groundwater dating Several attempts have previously been made to date groundwater in the investigated area using C isotopes. Šilar (1976) dated mainly groundwaters that were not affected by the CO 2 of deep origin. The author considered solely the Vogel approach assuming the initial 14 C activity equal to 85% of the 0.95 activity of the NBS oxalic acid standard ( Vogel, 1970 ). This model is a simplified approach that does not take into account the different mixing processes and considers only the radioactive decay of 14 C. Some dating results were obtained by Pačes et al. (2008) who considered two different approaches, the Pearson correction model ( Ingerson and Pearson, 1964 ) for the Turonian samples and the geochemical reaction model NETPATH ( Plummer et al., 1991 ) for the Cenomanian samples. As described earlier, the study area is exposed to numerous geochemical processes that lead to an overestimation of groundwater ages. The majority of the commonly used dating models do not consider dilution by 14 C-free CO 2 gas and interaction with organic C. It is proposed to use a δ 13 C mixing model based on the dilution factors modified from Clark and Fritz (1999) . The dilution correction model attempts to account for the dilution of 14 C by less active C and for isotopic exchange reaction, which may take place between the different C-bearing chemical species. The results that will be presented for the apparent radiocarbon age of the BCB groundwater are obtained using Eq. (1) ( Clark and Fritz, 1999 ): (1) t = - 8267 · ln a 14 C DIC / q · a 0 14 C where a 14 C DIC is the measured activity of 14 C, a 0 14 C corresponds to the 14 C activity of modern recharge waters (100 pmC), q is the dilution factor. When correcting 14 C for discrete geochemical processes, a process-specific dilution factor q is determined. The previous discussion on geochemical processes taking place in the aquifer led to proposing three dilution factors for the dilution of recharge waters: (i) by deep CO 2 ( q CO2( q ) ); (ii) by calcite dissolution ( q calcite ) and (iii) by the processes occurring between the groundwater and the organic matter. While the first two calculations can be applied (Eqs. (2) and (3) ), the latter for the organic matter becomes complicated. It is indeed very difficult to trace this process as it is not possible to clearly identify the end members of this interaction and therefore an analogous equation cannot be applied for the dilution factor. Only four samples reveal considerably depleted values in 13 C (No. 16, No. 17, No. 21 and No. 22), however, it is assumed that the very dominant process within the aquifers is interaction with deep CO 2 (g) rather than with organic matter. So the phenomenon of organic matter decomposition was ignored for the four above mentioned samples in the calculation and only dilution by deep CO 2 (g) was considered. The following equations for dilution factors are given here ( Clark and Fritz, 1999 ): (2) q CO 2 ( q ) = δ 13 C DICmeasured - δ 13 C CO 2 deep δ 13 C recharge - δ 13 C CO 2 deep (3) q calcite = δ 13 C DICmeasured - δ 13 C calcite δ 13 C recharge - δ 13 C calcite where δ 13 C DICmeasured represents the 13 C content measured in groundwater samples; δ 13 C recharge represents the 13 C content in recharged waters, which for the observed range of pH values corresponds to the 13 C content in soil (δ 13 C recharge ∼ δ 13 C soil ∼ −23‰; Clark and Fritz, 1999 ), δ 13 C CO2( q ) , δ 13 C calcite are the average values determined for CO 2 (g) of mantle origin (−2.7‰) and calcite (1.5‰), respectively. Dilution factors represent the degree of mixing which may range between 0 and 1. The dilutions factors are then provided in Table 3 . It is theoretically possible to consider both dilutions, i.e. with calcite and with CO 2 (g), and to calculate the total dilution factor according Eq. (4) . (4) q = q CO 2 deep · q calcite The total dilution factors q obtained from this calculation provide erroneous values ( q > 1) that are inadmissible, as mentioned above. For this reason, it was decided to calculate only individual dilution factors from Eqs. (2) and (3) regarding the geochemical context of each water sample established earlier. The key tool to identify processes and to decide which dilution factor should be considered for each sample is constituted by the 13 C– 14 C evolution graph ( Fig. 5 ). It must be stressed, that these simple models may improve the reliability of age estimates, but must be used with caution, and with an understanding of their sensitivity to the input parameters ( Clark and Fritz, 1999 ). Each sample of the dataset has been considered individually, and the dilution models – considering the dilution by CO 2 (g) and by C from calcite dissolution – have been chosen for each situation. The dilution factors, q , of Turonian waters No. 6, No. 7 were determined considering only calcite dissolution ( q = q calcite ). A large dilution by magmatic CO 2 (g) within the Turonian aquifer is not considered, because the majority of faults mainly affects the deep formations. Also, for Turonian samples No. 8, No. 9, No. 10 ( q = q calcite ) only dissolution of calcite was considered. These samples were collected in a tectonically relatively calm region; CO 2 flux from the upper mantle is then probably not involved. Their radiocarbon activities ( 14 C ∼ 63.8–78.8 pmC) indicate modern waters. It has previously been shown, that calcite dissolution affects the isotopic composition of two Cenomanian samples No. 19 and No. 20 and, therefore, for these waters the q calcite (Eq. (3) ) was also used. The rest of the samples (No. 4, No. 5, Nos. 11–18, No. 21 and No. 22) indicate a dominant interaction with magmatic CO 2 (g), therefore, Eq. (2) was used to calculate the dilution factor. Teplice samples No. 1 and No. 2 do not originate from sedimentary formations. The absence of calcite in Palaeozoic basement predetermines that the depletion in 13 C is not the result of calcite dissolution but rather of the interaction with magmatic CO 2 . This concept leads to negative ages. As expected, the dilution model is well adapted for sedimentary formations, but it failed while applied to the deep crystalline reservoirs. 5.3 Groundwater ages in the BCB According to the dating approach introduced in Section 5.2 , groundwater ages were calculated for most of the samples ( Table 3 ). The age calculation shows that the dilution model provides reliable results only for some samples. Palaeozoic waters (No. 1, No. 2) have to be excluded from the interpretation as the dilution model is not well adapted for crystalline aquifers. Sample No. 3 comes from the Quaternary, thus characterising modern waters. Similarly, Turonian samples No. 6, No. 7, No. 8, No. 9 and No. 10 also reveal modern origin. This result is in agreement with the available 18 O data which are similar to the modern isotopic content of precipitation. For No. 10, it may be additionally confirmed by the presence of 3 H. With the exception of No. 8 and No. 9, Turonian samples generally contain 3 H indicating the admixture of waters recharged in the second half of the 20th century ( Clark and Fritz, 1999 ). While some processes (e.g. 14 C-free CO 2 gas, organic C, carbonate rocks) artificially “age” groundwater, the contribution of modern water has the opposite effect. The existence of both tendencies may explain the apparent inconsistency frequently encountered between low radiocarbon activity and the presence of 3 H at the same time. The Cenomanian waters reveal two groups of groundwater ages. The group of “old” waters is represented by the majority of samples with infiltration from 2.1 to 11.1 ka BP. Waters No. 4 and No. 5 located on the Děčín fault zone reveal ages around 10 ka BP. As mentioned earlier, the stable isotopic content of Cenomanian samples and Turonian samples No. 4 and No. 5 reveal depleted values of 18 O between −10.1‰ and −11.2‰ vs. SMOW that corroborate the hypothesis of the infiltration of water partially influenced by different climatic conditions. But it should be kept in mind that even limited mixing with modern water (shown by 3 H content) can strongly modify the original palaeo-signature of groundwater. As a consequence, the ages, which were calculated using the dilution model, represent only the apparent age of groundwater. Strict radiocarbon ages might be considered for the seven samples without 3 H, i.e. No. 8, No. 9, Nos. 18–22. Samples No. 21 and No. 22 are located on the left bank of the Jizera River in close proximity to the fault zone, they were recharged 7 ka and 10.5 ka BP. The samples No. 18, No. 19 and No. 20 come from the NE part of the study area. In spite of their close geographical location, waters No. 19 and No. 20 have high 14 C activities and indicate an infiltration 200 and 600 a ago, they represent the group of “recent” Cenomanian waters. Sample No. 18, with an age of 4.5 ka BP, lies on one of the major faults of the study area, the Lužice fault, which might facilitate interactions between aquifers. 5.4 Conceptual model of the origin of carbon within the BCB It emerged that detailed investigation of the origin of C isotopes can help to determine the several geochemical processes occurring within the aquifers. Although the different chemical reactions affecting the C isotopic signature of TDIC may be adverse for further dating efforts, C isotopes are excellent tracers that help to determine the C origin within the system. Based on the results of several stable and radioactive isotope determinations, different C origins were determined in the BCB allowing the development of a model including all potential C source contributions. The simplified concept is schematized in Fig. 7 , which introduces the very complex geological system and which summarizes the geochemical processes discussed (Section 5.1 ). Special attention has been given to the identification of C origin in the BCB waters. Groundwater is generally drained by rivers, represented in Fig. 7 by the Labe (Elbe) River. Meteoric water with a typical atmospheric stable and radioactive isotopic composition (δ 13 C ∼ −8‰ and elevated 14 C activity) infiltrated into the reservoir. It is necessary to note, that once the meteoric water reaches the surface, interaction with soil and biogenic C lowers the δ 13 C values to approximately −14‰. The biogenic C with values of about δ 13 C ∼ −23‰ can be flushed directly to the reservoirs and may partially influence the shallow horizons. On the other hand, the groundwater reveals low a 14 C, which is accounted for by three phenomena. First, very low radiocarbon activities reveal the ascent of CO 2 (g) from the upper mantle having itself a very low radiocarbon activity. In addition, the isotopic signature together with the geochemical indicators suggests strong water–rock interactions, which are mainly achieved with the calcite cement in the sandstone layers. The calcite generally has very enriched 13 C values, for the Cenomanian and Turonian samples in a range from 1‰ to 2.1‰. Therefore, calcite dissolution is partially responsible for the enriched δ 13 C values measured in groundwater. Fig. 5 and the evolution of Ca 2+ and HCO 3 - concentrations suggest that the Turonian samples reveal more intensive water–rock interactions than those of the Cenomanian. This may be due to the fact that the Cenomanian aquifer is considerably influenced by magmatic C, which dominates over the C coming from the calcite dissolution. The last 14 C-free component interfering in this hydrogeological system is the C from fossil organic matter. Organic matter (δ 13 C ∼ −20‰ to −27‰) is distributed irregularly within the BCB. The water can interact directly either with coal-bearing Permo-Carboniferous layers or with fossil organic matter issued from the Cenomanian deposits. The distribution of all these components within the hydrogeological system is closely linked to the tectonic features of the region. A great part of the area (especially the NW) has been considerably affected by tectonic events which has resulted in numerous faults and fractures. This part was subsequently affected by numerous magmatic intrusions as schematically shown in Fig. 7 . From the dating results introduced in Table 3 and from the location of sampling wells ( Fig. 3 ), it follows that the major fault systems (e.g. Lužice fault, Děčín fault system; Fig. 1 ) have considerable influence on the groundwater pattern. It was confirmed that the distribution and exchange of isotopes within aquifers happens preferentially in the fault structures as the interaction with deep CO 2 (g) appears to be a dominant process in the aquifers of the western part of the BCB. The geometry of the fault structure is the principle feature determining magmatic CO 2 distribution. It was shown, that the majority of the Turonian waters are not affected by deep CO 2 (g) interactions. However, from the isotopic signature of two samples from the Děčín fault system (No. 4, No. 5) it can be concluded that the faults often intersect both aquifers. Deep faults doubtless represent the main pathway for gas ascent, but in the shallower horizons, the gas might also be distributed by shallower fractures. As observed by Jetel and Rybářová (1991) , fracture porosity dominates over the intergranular in the sandstone aquifers, which facilitates gas distribution also within the aquifer itself. Although the faults and fractures do not always affect the direction of groundwater flow ( Šilar, 1976; Herčík et al., 1999 ), they can facilitate interactions between atmosphere, aquifers and upper mantle chemical components. 6 Conclusions Isotopic research, carried out in the very complex aquifer system of the north-western part of the Czech Republic has demonstrated that groundwater evolution is dominated by many kinds of interactions: (i) groundwater–CO 2 gas from the upper mantle, (ii) groundwater–rock, (iii) groundwater–fossil organic matter and (iv) groundwater–surface waters. Carbon isotopes ( 13 C, 14 C) in such an intricate context behave as powerful tracers of groundwater flow and origin. From the detailed study of the aquifer lithology and tectonic conditions it was possible to present for the first time a simplified conceptual scheme of the basin. Considering the complex flow conditions, a method has been developed to evaluate the apparent residence time of groundwater within the system. The apparent groundwater ages range from modern to 11.1 ka BP. The stable isotopic content, mainly 18 O values, indicates both the contribution of modern precipitation and the partial infiltration of palaeowaters from the end of the Pleistocene. Due to this study, it is now possible to focus the investigation on strategic areas such as fault zones, where exchanges between different aquifers along deep discontinuities still need to be clarified and quantified. As a future step, these considerations will help in water management of the area to sustainably develop exploitation of the resource, taking into account its dynamics and renewability. Acknowledgements Financial support for this work was provided by the Grant Agency of the Czech Republic (GACR 205/07/0691). This work was also supported by the French Ministry of Foreign Affairs and the Ministry of Education, Youth and Sports of the Czech Republic. In particular, we thank the French Embassy in Prague for help throughout the project. The authors would like to thank Pr. Benjamin van Wyk de Vries for English language revision. References Andersen et al., 2005 M.S. Andersen V.N.R. Jakobsen D. Postma Geochemical processes and solute transport at the seawater/freshwater interface of a sandy aquifer Geochim. Cosmochim. Acta 69 2005 3979 3994 Appelo and Postma, 2005 C.A.J. Appelo D. Postma Geochemistry, Groundwater and Pollution 2005 A.A. Balkema Publishers Leiden Bates et al., 2007 Bates, B., June, N., Harper, K., Mayo, A.L., Tingey, D., Vilhelm, Z., 2007. Preliminary chemical and isotopic analyses of groundwater systems in the northern Bohemian Cretaceous Basin, Czech Republic. Rocky Mountain Section – 59th Ann. Meeting. Geol. Soc. Am. Abstracts with Programs, vol. 39(5), p. 38. Bergfeld et al., 2001 D. Bergfeld F. Goff C.J. Janik Carbon isotope systematics and CO 2 sources in the Geysers–Clear Lake region, northern California, USA Geothermics 30 2001 303 331 Birkle et al., 2001 P. Birkle B. Merkel E. Portugal I.S. Torres-Alvarado The origin of reservoir fluids in the geothermal field of Los Azufres, Mexiko – isotopical and hydrological indications Appl. Geochem. 16 2001 1595 1610 Brožek, 1987 Brožek, P., 1987. Bukovno-CHMU. Hydrogeological Survey. Report of Stavební Geologie, Prague. GF P059058 (Geofond archive) (in Czech). Čadek, 1968 Čadek, J., 1968. Hydrogeology of the Teplice and Ústí Thermal Waters. Sborník geologických věd HIG, vol. 6. Ústřední ústav geologický, Prague, pp. 7–207 (in Czech). Čapek, 1979 Čapek, A., 1979. Mladá Boleslav-Bělá pod Bezdězem: Evaluation of Wells. Report of Vodní Zdroje, Prague. GF P029955 (Geofond archive) (in Czech). Carreira et al., 2008 P.M. Carreira J.M. Marques R.C. Graça L. Aires-Barros Radiocarbon application in dating complex hot and cold CO 2 -rich mineral water systems: a review of case studies ascribed to the northern Portugal Appl. Geochem. 23 2008 2817 2828 Čech et al., 2005 S. Čech L. Hradecká M. Svobodová L. Švábenická Cenomanian and Cenomanian–Turonian boundary in the southern part of the Bohemian Cretaceous Basin, Czech Republic Bull. Geosci. 80 4 2005 321 354 Celle-Jeanton et al., 2009 H. Celle-Jeanton F. Huneau Y. Travi W.M. Edmunds Twenty years of groundwater evolution in the Triassic sandstone aquifer of Lorraine: impacts on baseline quality Appl. Geochem. 24 2009 1198 1213 Clark and Fritz, 1999 I.D. Clark P. Fritz Environmental Isotopes in Hydrogeology 1999 Lewis Publishers Boca Raton Coetsiers and Walraevens, 2009 M. Coetsiers K. Walraevens A new correction model for 14 C ages in aquifers with complex geochemistry – application to the Neogene Aquifer Belgium Appl. Geochem. 24 2009 768 776 Czech Hydrometeorological Institute, 2009 Czech Hydrometeorological Institute, 2009. < http://www.chmi.cz/meteo/ok/infklim.html >. Darling, 2004 W.G. Darling Hydrological factors in the interpretation of stable isotopic proxy data present and past: a European perspective Quatern. Sci. Rev. 23 2004 743 770 Datel et al., 2009 J.V. Datel M. Kobr M. Procházka Well logging methods in groundwater surveys of complicated aquifer systems: Bohemian Cretaceous Basin Environ. Geol. 57 2009 1021 1034 Deines et al., 1974 P. Deines D. Lagmuir R.S. Harmon Stable isotope ratios and existence of gas phase in the evolution of carbonate groundwaters Geochim. Cosmochim. Acta 38 1974 1147 1164 Douez, 2007 Douez, O., 2007. Réponse d’un système multicouche aux variations paléoclimatiques et aux solicitations anthropiques: Approche par modélisation couplée hydrodynamique, thermique et géochimique. Doct. Thesis. Univ. Bordeaux, p. 3. Edmunds, 2005 W.M. Edmunds Groundwater as an archive of climatic and environmental change P.K. Aggarwal J. Gat K. Froehlich Isotopes in the Water Cycle. Past, Present and Future of a Developing Science 2005 Springer 341 352 Edmunds and Shand, 2008 W.M. Edmunds P. Shand Natural Groundwater Quality 2008 Blackwell Publishing Oxford Edmunds and Smedley, 2000 W.M. Edmunds P.L. Smedley Residence time indicators in groundwater: the East Midlands Triassic sandstone aquifer Appl. Geochem. 15 2000 737 752 Edmunds et al., 2002 W.M. Edmunds J.J. Carillo-Rivera A. Cardona Geochemical evolution of groundwater beneath Mexico City J. Hydrol. 258 1–4 2002 1 24 Edmunds et al., 2003 W.M. Edmunds A.H. Guendouz A. Mamou A. Moulla P. Shand K. Zouari Groundwater evolution in the Contitnental Intercalaire aquifer of southern Algeria and Tunisia: trace element and isotopic indicators Appl. Geochem. 18 2003 805 822 Ehlers and Gibbard, 2004 J. Ehlers P.L. Gibbard Quaternary Glaciations – Extent and Chronology Part I: Europe 2004 Elsevier Amsterdam Fediuk, 1996 F. Fediuk Permian ignimbritic rhyolite in Tachov Hill near Doksy, N-Bohemia Veštník Českého Geologického Ústavu 71 2 1996 145 150 Fontes, 1985 Fontes, J.C., 1985. Some considerations on ground water dating using environmental isotopes. Hydrogeology in the Service of Man. Memoires of the 18th Congress of the International Association of Hydrogeologists, Cambridge, pp. 118–151. Frape et al., 2007 S.K. Frape O. Shouakar-Stash T. Pačes R. Stotler Geochemical and isotopical characteristics of the waters from crystalline and sedimentary structures of the Bohemian Massif T.D. Bullen Yanxin. Wang Proc. Water–Rock Interaction vol. 12 2007 Taylor & Francis Group Balkema, London 727 733 Geissler et al., 2005 W.H. Geissler H. Kämpf R. Kind K. Bräuer K. Klinge T. Plenefish J. Horálek J. Zedník V. Nehybka Seismic structure and location of a CO 2 source in the upper mantle of the western Eger (Ohře) Rift, central Europe Tectonics 24 2005 TC5001 10.1029/2004TC001672 Herčík et al., 1999 Herčík, F., Hermann, Z., Valečka, J., 1999. Hydrogeology of the Bohemian Cretaceous Basin Czech Geological Survey, Prague (in Czech). Hercog, 1965 Hercog, F., 1965. The Calculation of the Groundwater Reserves in the “Podlažice Depression” Sbor. ref. celostát. Aktivu Využitelné množství podzemních vod, Prague, pp. 47–67 (in Czech). Hladíková et al., 1987 Hladíková, J., Pačes, T., Šmejkal, V., 1987. The stable isotope study of dissolved components in some mineral and thermal waters in Czechoslovakia. International Symposium on the Use of Isotope Techniques in Water Resource Development. Extended Synopsis, IAEA-SM-299, IAEA, pp. 54–55. Huneau and Blavoux, 2000 Huneau, F., Blavoux, B., 2000. Isotopic Hydrogeology within the Miocene Basin of Carpentras-Valreas (southeastern France), vol. 262. IAHS-AISH Publication, pp. 433–438. Huneau and Travi, 2008 F. Huneau Y. Travi The Miocene aquifer of Valréas, France W.M. Edmunds P. Shand Natural Groundwater Quality 2008 Blackwell Publishing London 87 305 Huneau et al., 2001 F. Huneau B. Blavoux Y. Bellion Differences between hydraulic and radiometric velocities of groundwaters in a deep aquifer: example of the Valréas Miocene aquifer (southeastern France) Compt. Rend. Acad. Sci. – Serie IIa: Sciences de la Terre et des Planetes 333 3 2001 163 170 Hynie, 1949 Hynie, O., 1949. The Bohemian Groundwater Reservoirs in the Water Management. Geotechnika, vol. 8. Ústřední ústav geologický, Prague (in Czech). Hynie, 1961 Hynie, O., 1961. Hydrogelogy of Czechoslovakia I: Fresh Waters. Academia, Prague (in Czech). IAEA/WMO, 2006 IAEA/WMO, 2006. Global Network of Isotopes in Precipitation. The GNIP Database. < http://isohis.iaea.org >. Ingerson and Pearson, 1964 E. Ingerson F.J. Pearson Jr. Estimation of age and rate of motion of ground-water by the 14 C method Y. Miyake T. Koyama Recent Researches in the Fields of Hydrosphere, Atmosphere and Nuclear Geochemistry 1964 Maruzen Tokyo 263 283 Jetel, 1970 Jetel, J., 1970. Hydrogeology of the Permo-Carboniferous and Cretaceous in the Profile Melnik – Jested. Sborník geologických věd HIG, 77-42. Ústřední ústav geologický, Prague (in Czech). Jetel, 1982 Jetel, J. 1982. Hydrogeology of the Black-Coal Deposits in the Mšeno Basin. Sborník geologických věd HIG, vol. 16. Ústřední ústav geologický, Prague, pp. 33–39 (in Czech). Jetel and Krásný, 1976 Jetel, J., Krásný, J., 1976. Approximative Aquifer Character in Regional Hydrogeologic Study, vol. 6. Věstník Ústředního ústavu geologického, pp. 459–461. Jetel and Rybářová, 1991 Jetel, J., Rybářová, L., 1991. Hydrogeology of the Cenomanian aquifer in the Labe region of the Bohemian Cretaceous Basin. Sborník geologických věd HIG, vol. 19. Ústřední ústav geologický, Prague, pp. 9–63 (in Czech). Jiráková et al., 2009 H. Jiráková F. Huneau H. Celle-Jeanton Z. Hrkal P. Le Coustumer Palaeorecharge conditions of the deep aquifers of the Northern Aquitaine region (France) J. Hydrol. 368 2009 1 16 Jost, 2005 Jost, A., 2005. Caractérisation des forçages climatiques et géomorphologiques des cinq derniers millions d’années et modélisation de leurs conséquences sur un système aquifère complexe: le bassin de Paris. Doct. Thesis, vol. VI. Univ. Pierre et Marie Curie, Paris. Klein, 1979 Klein, V., 1979. The Basement Composition and Morphology of the Cretaceous Basin on the West from Hradce Králové, vol. 53. Věstník Ústředního ústavu geologického, Prague, pp. 357–366. Kliner and Kněžek, 1974 Kliner, K., Kněžek, M., 1974. Method of Groundwater Flux Separation Using the Water Level Observation, vol. 22(5). Vodohospodářský časopis, Bratislava, pp. 457–466 (in Czech). Kolářová and Krásný, 1972 Kolářová, M., Krásný, J., 1972. Hydrogeology of the Poděbrady spa Region. Sborník geologických věd HIG, vol. 9. Ústřední ústav geologický, Prague (in Czech). Kopecký, 1978 Kopecký, L., 1978. Neoidic Taphrogenic Evolution and Young Alkaline Volcanism of the Bohemian Massif. Czechoslovakia. Sborník geologických věd GEO, vol. 31. Ústřední ústav geologický, Prague, pp. 91–107 (in Czech). Krásný, 1973 Krásný, J., 1973. Static Analyses of Hydrogeological Data from the Permo-Carboniferous Basin in the Piedmont of the Krokonoše Mountains. Sborník geologických věd HIG, vol. 13. Ústřední ústav geologický, Prague, pp. 113–152 (in Czech). Levin et al., 1995 I. Levin R. Graul N.B.A. Trivett Long-term observations of atmospheric CO 2 and carbon isotopes at continental sites in Germany Tellus 47B 1995 23 34 Malkovský, 1976 M. Malkovský Saxonic tectonics of the Bohemian Massif Int. J. Earth Sci. 65 1976 127 143 (in German) Marques et al., 1996 J.M. Marques L. Aires-Barros R.C. Graça The origin and relation among hot and cold CO 2 -rich mineral waters in Vilarelho da Raia–Pedras Salgadas region, northern Portugal: a geochemical approach Geotherm. Resour. Council Trans. 20 1996 363 369 Martínek et al., 2006 K. Martínek M. Blecha V. Daněk J. Franců J. Hladíková R. Johnová D. Uličný Record of palaeoenvironmental changes in a Lower Permian organic-rich lacustrine succession: integrated sedimentological and geochemical study of the Rudník member, Krkonoše Piedmont Basin, Czech Republic Palaeogeogr. Palaeoclimatol. Palaeoecol. 230 2006 85 128 Mook, 2000 Mook, W.G., 2000. Environmental Isotopes in the Hydrological Cycle – Principles and Applications. IAEA, UNESCO, Vienna. Noseck et al., 2009 U. Noseck K. Rozanski M. Dulinski V. Havlová O. Sracek T. Brasser M. Hercik G. Buckau Carbon chemistry and groundwater dynamics at natural analogue site Ruprechtov, Czech Republic: insights from environmental isotopes Appl. Geochem. 24 2009 1765 1776 Novák et al., 2003 M. Novák F. Buzek A.F. Harisson E. Přechová I. Jačková D. Fottová Similarity between C, N and S stable isotope profiles in European spruce forest soils: implications for the use of δ 34 S as a tracer Appl. Geochem. 18 2003 765 779 Pačes, 1974 T. Pačes Springs of Carbon-dioxide Waters in Northwestern Bohemia. Field trip guide 1974 Czech Geological Survey Prague Pačes, 1987 Pačes, T., 1987. Hydrochemical evolution of saline waters from crystalline rocks of the Bohemian Massif (Czechoslovakia). In: Fritz, P., Frape, S.K. (Eds.), Saline Water and Gases in Crystalline Rocks. Geol. Assoc. Can. Spec. Paper 33, pp. 145–156. Pačes and Šmejkal, 2004 T. Pačes V. Šmejkal Magmatic and fossil components of thermal and mineral waters in the Eger River continental rift (Bohemian Massif, central Europe) R.B. Wanty R.R. Seal II Water–Rock Interaction vol. 11 2004 Balkema Publishers 161 172 Pačes et al., 2001 T. Pačes P. Möler A. Fuganti G. Morteani J. Pěček Sparkling mineral water at western rim of the Doupovské hory Mountains (Czech Republic): genesis by water–rock interaction and deep-seated CO 2 Bull. Czech Geol. Surv. 76 4 2001 189 202 Pačes et al., 2008 T. Pačes J.A. Corcho Alvarado Z. Herrmann V. Kodeš J. Mužák J. Novák R. Purtschert D. Remenárová J. Valečka The Cenomanian and Turonian Aquifers of the Bohemian Cretaceous Basin, Czech Republic W.M. Edmunds P. Shand Natural Groundwater Quality 2008 Blackwell Publishing Ltd. 72 390 (Chapter 17) Plummer et al., 1991 Plummer, L.N., Prestemon, E.C., Parkhurst, D.L., 1991. An Interactive Code (NETHPATH) for Modeling Net Geochemical Reactions Along a Flow Path. US Geol. Surv., Water Resour. Invest. Rep. 91-4078. Raoult et al., 1997 Y. Raoult J. Boulègue J. Lauverjat P. Olive Contribution de la géochimie à la compréhension de l’hydrodynamisme de l’aquifère de l’Albien dans le Bassin de Paris Compt. Rend. Academie Sci. – Série Iia Sciences de la Terre et des Planetes 325 6 1997 419 425 Raoult et al., 1998 Y. Raoult J. Lauverjat J. Boulègue P. Olive T. Bariac Étude hydrogéologique d’une ligne d’écoulement de l‘aquifère de l’Albien dans le bassin de Paris entre Gien-Auxerre et Paris Bull. Soc. Géol. France 169 1998 453 457 Rice and Kotarba, 1993 Rice, D.D., Kotarba, M., 1993. Origin of Upper Carboniferous coal bed gases, Lower and Upper Silesian coal beds, Poland. In: Proc. 1993 Int. Coalbed Methane Symp., pp. 649–658. Rose and Davisson, 1996 T.P. Rose M.L. Davisson Radiocarbon in hydrologic systems containing dissolved magmatic carbon dioxide Science 273 1996 1367 1370 Rose et al., 1996 T.P. Rose M.L. Davisson R.E. Criss Isotope hydrology of voluminous cold springs in fractured rock from an active volcanic region, northeastern California J. Hydrol. 179 1996 207 236 Šilar, 1976 Šilar, J., 1976. Radiocarbon Ground-Water Dating in Czechoslovakia – First Results, vol. 51. Věstník Ústředního ústavu geologického, Prague, pp. 209–220. Šilar, 1989 J. Šilar Radiocarbon dating of ground water in Czechoslovakia and paleoclimatic problems of its origin in central Europe. Zeszyty Naukowe Politechniki Slaskiej, Ser. Mat.-Fiz. 61 Geochronometria 6 1989 133 141 Šilar, 2007 Šilar, J., 2007. Floods and groundwater resources in emergency situations. In: Vrba, J., Salamat, A.R. (Eds.), Groundwater for Emergency Situations. Proc. Int. Workshop, Tehran, 29–31 October 2006, pp. 15–34. Skořepa, 1993 Skořepa, J., 1993. Roudnice – Hostěraz, Dump of Solid Waste. Report of Aquatest a.s., Prague. GF P079538 (Geofond archive) (in Czech). Toucanne et al., 2009 S. Toucanne S. Zaragosi J.F. Bourillet M. Cremer F. Eynaud B. Van Vliet-Lanoe A. Penaud C. Fontanier J.L. Turon E. Cortijo P.L. Gibbard Timing of massive ‘Fleuve Manche’ discharges over the last 350 kyr: insights into the European ice-sheet oscillations and the European drainage network from MIS 10 to 2 Quatern. Sci. Rev. 28 2009 1238 1256 Truesdell and Hulston, 1980 A.H. Truesdell J.R. Hulston Isotopic evidence on environments of geothermal systems P. Fritz J.C. Fontes Handbook of Environmental Isotope Geochemistry, The Terrestrial Environment vol. 1 1980 Elsevier 179 226 Uličný, 1997 Uličný, D., 1997. Sedimentation in a Reactivated, Intra-Continental Strike-Slip Fault Zone: The Bohemian Cretaceous Basin, Central Europe. Gaea Heidelbergensis, 3, Abstracts, 18th IAS Regional European Meeting, Heidelberg, p. 347. Uličný, 2001 D. Uličný Depositional systems and sequence stratigraphy of coarse-grained deltas in a shallow-marine, strike-slip setting: the Bohemian Cretaceous Basin, Czech Republic Sedimentology 48 2001 599 628 Van Der Kemp et al., 2000 W.J.M. Van Der Kemp C.A.J. Appelo K. Walraevens Inverse chemical modelling and radiocarbon dating of palaeogroundwaters: the tertiary Ledo-Paniselian aquifer in Flanders, Belgium Water Resour. Res. 34 2000 1277 1287 Vogel, 1970 Vogel, J.C., 1970. Carbon-14 Dating of Groundwater. Isotope Hydrology. IAEA. Vienna, pp. 225–239. Vogel, 1993 J.C. Vogel Variability of carbon isotope fractionation during photosynthesis J.R. Ehleringer A.E. Hall G.D. Farquhar Stable Isotopes and Plant Carbon–Water Relations 1993 Academic Press San Diego, CA 29 38 Voigt and Hilbrecht, 1997 S. Voigt H. Hilbrecht Late Cretaceous carbon isotope stratigraphy in Europe: correlation and relations with sea level and sediment stability Palaeogeogr. Palaeoclimatol. Palaeoecol. 134 1997 39 59 Weinlich et al., 1998 F.H. Weinlich J. Tesař S.M. Weise K. Bräuer H. Kämpf Gas flux distribution in mineral springs and tectonic structure in the western Eger Rift J. Czech Geol. Soc. 43 1–2 1998 91 110 Weinlich et al., 1999 F.H. Weinlich K. Bräuer H. Kämpf G. Strauch J. Tesař S.M. Weise An active subcontinental mantle volatile system in the western Eger Rift, central Europe: gas flux, isotopic (He, C, and N) and compositional fingerprints Geochim. Cosmochim. Acta 63 1999 3653 3671 Weinlich et al., 2003 F.H. Weinlich K. Bräuer H. Kämpf G. Strauch J. Tesař S.M. Weise Gas flux and tectonic structure in the western Eger Rift, Karlovy Vary – Oberpfalz and Oberfranken, Bavaria Geolines 15 2003 181 187 Weise et al., 2004 F. Weise S. Čech B. Ekrt M. Košťák M. Mazuch S. Voigt The Upper Turonian of the Bohemian Cretaceous Basin (Czech Republic) exemplified by the Úpohlavy working quarry: integrated stratigraphy and palaeoceanography of a gateway to the Tethys Cretaceous Res. 25 2004 329 352 Žitný, 1970 Žitný, L., 1970. Hydrogeological Evaluation. Dolánky in the Český Dub vicinity. Report of Vodní Zdroje, Prague. GF P022249 (Geofond archive) (in Czech).
更多查看译文
关键词
aquifers,upper mantle,geochemistry,surface water,carbon,stable isotope,ice sheets,stable isotopes,conceptual model,groundwater,residence time,organic matter,carbon isotope
AI 理解论文
溯源树
样例
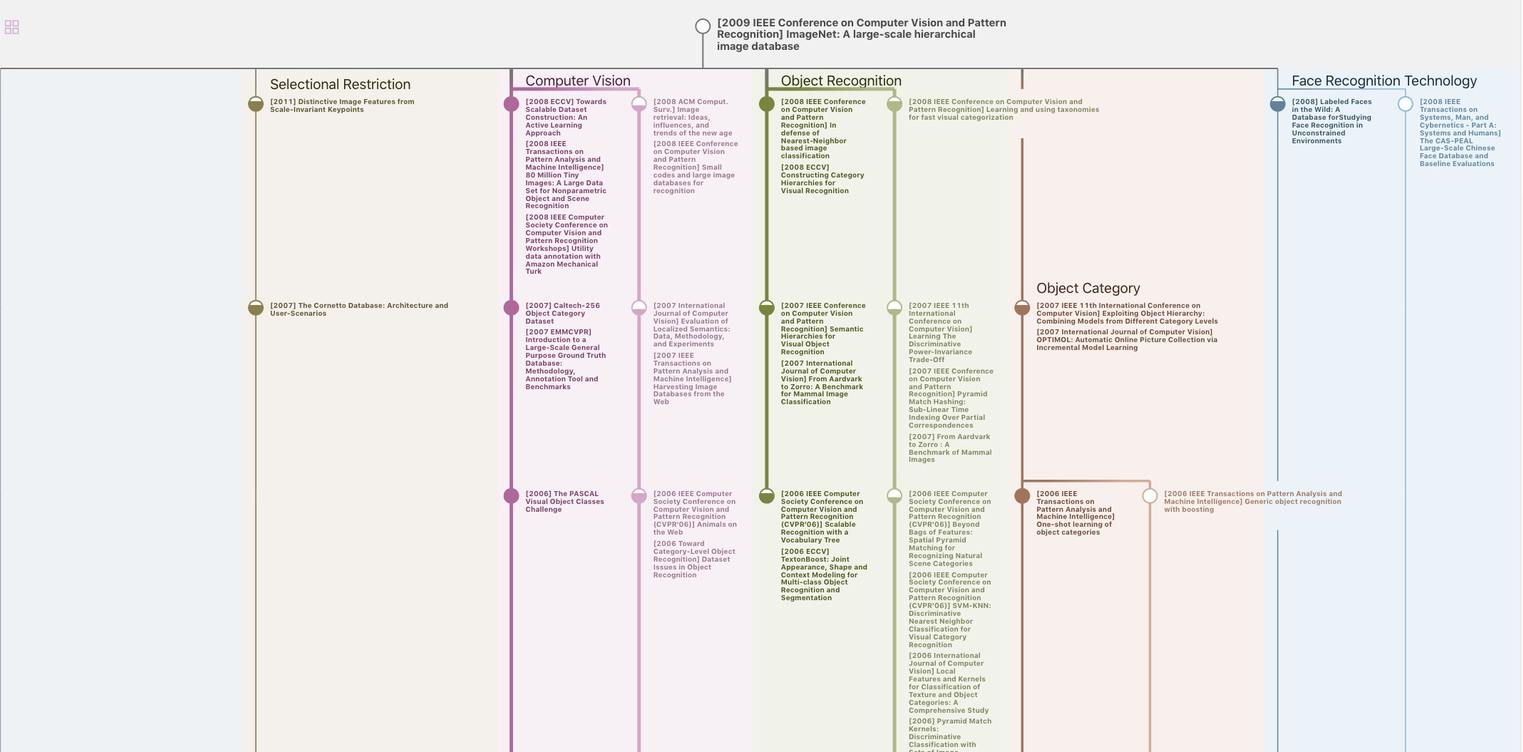
生成溯源树,研究论文发展脉络
Chat Paper
正在生成论文摘要