Biogenic structures of two ant species Formica sanguinea and Lasius flavus altered soil C, N and P distribution in a meadow wetland of the Sanjiang Plain, China
Applied Soil Ecology(2010)
摘要
Biogenic structures produced by soil ecosystem engineers influence the soil architecture and mediate soil functions and ecosystem services. Ant mounds in meadow wetlands are important biogenic structures with the potential of altering carbon storage and nutrient cycling in these ecosystems. In this study, we examined the soil nutrient concentrations of ant mounds and their effects on the wetland nutrient storage functions in meadow wetlands of the Sanjiang Plain, in northeastern China. The aims of this study were to investigate C, N and P variation in active ant mounds produced by Formica sanguinea Latreille and Lasius flavus Fabricius to estimate the C, N and P pools of ant mounds in comparison with control soil. The average total N (TN), total P (TP) and available P (AP) concentrations in the ant mounds of both species were higher than in the control soil. Organic carbon (C org ), DOC, NH 4 + and NO 3 − in F. sanguinea mounds were higher than in the control soil, but not for L. flavus mounds. Average concentrations of all the five types of nutrient were higher in F. sanguinea mounds than in L. flavus mounds. The variations in C org , DOC, TN and TP concentrations in ant mounds were not significant at depths from 0 to 25 cm. NH 4 + and NO 3 − concentrations differed by soil layers for F. sanguinea mounds but not for L. flavus m ounds. The C/N ratios were generally lower in the mounds than in the control soil (at 5–25 cm), but no significant differences were found for C/P ratios (except at 10–15 cm). Carbon and DOC pools were smaller, TN and AP pools were larger in ant mounds compared with the control soil, but there was no significant difference for TP pools. NH 4 + and NO 3 − pools were substantially larger in F. sanguinea mounds, but smaller in L. flavus mounds, than those in the control soil. All of the five types of nutrient pools were larger in F. sanguinea than in L. flavus mounds. Ant mounds increased the spatial variability of soil nutrient pools in the wetland. Keywords Ant mounds Soil fauna Nutrient dynamic Heterogeneity Wetland 1 Introduction Soil nutrient dynamics and functions are significantly influenced by the biological activities of soil fauna. In soil, the relative importance of regulation imposed by ecosystem engineers ( sensu Jones et al., 1994 ) is likely to be greater than regulation by foodweb complexity (trophic relationships) ( Lavelle, 2002 ). Ecosystem engineers are organisms that move through the soil and build organo-mineral structures with specific physical, chemical and microbiological properties, directly or indirectly modulating the availability of resources to other species ( Jones et al., 1994, 1997 ). The ability to build biogenic structures is the heart of the soil engineering concept ( Lavelle, 1997 ). Biogenic structures such as mounds, nests, sheathings or casts, galleries, chambers and the specific environment associated with them are defined as the “functional domain” of a given ecosystem engineer, with the specific communities of organisms and the soil processes operating at certain spatial and temporal scales ( Lavelle, 2002; Jiménez et al., 2008 ). Jouquet et al. (2006) suggested that ‘extended phenotype engineers’ such as termites and ants whose activities may be concentrated at a few points have a greater effect on the maintenance of ecosystem heterogeneity, than ‘accidental engineers’ such as geophagous earthworms whose activities may often extend over a wider area. Ants are an important component of ecosystems because they can constitute a significant part of the animal biomass and also act as ecosystem engineers ( Jones et al., 1994; Folgarait, 1998 ). Ants affect soil physical structure ( Folgarait, 1998 ), nutrient dynamics ( Petal and Kusinska, 1994; Wagner et al., 2004; Holec and Frouz, 2006; Jiménez and Decaëns, 2006; Kilpeläinen et al., 2007; Jiménez et al., 2008 ) and biological properties ( Dauber and Wolters, 2000; Boulton and Amberman, 2006; Amador and Görres, 2007 ) of the soil environment by building the mounds or nests which they live in. However, most studies on the biogenic structures of ants have been conducted in boreal forests ( Kilpeläinen et al., 2007 ), neotropical savannas ( Jiménez and Decaëns, 2006 ), arid grassland ( Wagner et al., 2004 ) and agricultural field ( Amador and Görres, 2007 ), with a few studies in temperate meadow wetland ecosystems ( Petal, 1980; Petal and Kusinska, 1994 ). Wetland ecosystems comprise one of the most important carbon pools globally, and the effects of biogenic structures on the soil nutrient dynamics will directly influence the wetland functions but these aspects have been neglected to a great extent. Furthermore, nutrient concentration studies have generally focused on the biogenic structure as a whole ( Verchot et al., 2003; Wagner et al., 2004; Holec and Frouz, 2006; Kilpeläinen et al., 2007 ) rather than analyzing the different components ( Frouz et al., 2003; Jiménez and Decaëns, 2006; Jiménez et al., 2008 ). The Sanjiang Plain is one of the largest and most entire freshwater wetlands in China. In the past 50 years, it has been extensively drained or reclaimed and thus has been significantly degraded ( Liu and Ma, 2002 ). A number of ant mounds are present in the meadow wetlands and sometimes may cover a large proportion of the soil surface. While studies on wetland structure, ecological processes, functions and their changes have been widely conducted ( Liu and Ma, 2002; Wu et al., 2007; Lu, 2009 ), there is a lack of studies describing and characterizing the structures produced by ants despite their tremendous potential for changing the ecosystem properties of the wetlands. The aim of this study was to determine if the variation in C, N and P contents of active mounds of two ant species Formica sanguinea and Lasius flavus , could influence soil properties in wetlands. We tested the hypotheses that there were no differences between control and ant mound affected soils at various depths in regard to C, N, P concentrations, C/N and C/P ratios and C, N and P pools. 2 Materials and methods 2.1 Study site and organisms The Sanjiang Plain is a low floodplain, located in Heilongjiang Province of Northeast China, which was formed by the Heilong, the Songhua and the Wusuli rivers. The study was carried out in August 2008, at the Sanjiang Mire Wetland Experimental Station, Chinese Academy of Sciences (47°13″50′N, 133°13″10′E) located in the Sanjiang Plain. This region experiences a temperate moist monsoon climate with a mean annual temperature of 1.9 °C and a mean annual precipitation of 600 mm. The average monthly temperature is −21 °C in January and 22 °C in July. More than 60% of the annual precipitation falls between July and September. The average altitude of the study area is 55 m ( Song et al., 2006 ). Calamagrostis augustifolia meadow wetland is the representative wetland type in this plain where C. augustifolia is the dominant grass species and the accompanying species consist of Lythrum salicaria , Sanguisorba parviflora , Vicia cracca , Filipendula palmate , Trifolium lupinaster and Pedicularis sceptrum-corolinum . The main soil types are meadow soil and albic soil with different hydrological regimes of perennial moist or temporary flooded ( Chen, 1996 ). Mounds of F. sanguinea and L. flavus are common in C. augustifolia meadow wetland of the Sanjiang Plain. The mounds are often constructed from soil excavated from deeper soil layers and plant materials such as leaf litter and twigs. The mounds can be easily identified in the meadow landscape by the pronounced above-ground part typically with distinct edges and with few or no grass plants growing on the surface of the mound. The aboveground portions of the mounds of both species are often orange or yellowish domes, but the physical characteristics and material compositions are significant different as indicated in Table 1 . 2.2 Soil sampling and chemical analysis The study was conducted in August 2008, mid summer at the site. Mounds were grouped by the dominant ant species, and all mounds were in C. augustifolia meadow wetlands. We collected six samples from mounds produced by each ant species. The sampled mounds were selected randomly and were at least 50 m apart to ensure their complete independence. In order to investigate spatial variation in the mounds, the soil was vertically sampled to a depth of 25 cm, from the surface to the base of the mound, using a stainless steel cylinder (14 cm diameter) and the samples were subdivided at 5 cm intervals. Control soil samples were obtained by stratified sampling to a 25 cm depth at sites with no mounds within 3 m. At the same time as the sampling, three replicate samples were taken from every soil layer using the ring sampler method to measure soil bulk density ( Lu, 1999 ). Soil samples for chemical analysis taken from the different layers were placed separately in identifiable plastic bags, stored immediately in an ice chest to preserve further mineralization processes ( Holec and Frouz, 2006; Jiménez et al., 2008 ), and refrigerated at 4 °C until analysis. Subsamples, in which ants, stones and other impurity were removed, were dried to constant weight at 40 °C, milled, and sieved through 2 mm sieve for elemental analysis. The chemical analyses were performed at the Key Laboratory of Wetland Ecology and Environment, Chinese Academy of Sciences. Total organic carbon (C org ) and nitrogen (TN) in soil were measured with a Carlo Erba FLASHEA 1112 CHN-S analyzer. Total Phosphorus (TP) concentration was determined by plasma emission spectrometer after H 2 SO 4 + HClO 4 wet-digestion. Inorganic N concentrations in NO 3 − and NH 4 + form were measured using a colorimetric method, and available phosphorus (AP) was determined by HCl–H 2 SO 4 digestion ( Lu, 1999 ). 2.3 Statistical analysis Means and standard errors of contents of C org and nutrient concentrations were calculated using the SPSS 14.0 software package. We calculated soil nutrient storage by multiplying soil nutrient concentrations by sample depth and bulk density. For example, TC storage was calculated using the following formula: T c = ∑ i = j n d v i × C i × h i 10 T c (kg m −2 ) is the carbon storage from layers j to n ; dv i (g cm −3 ) is the soil bulk density; C i (%) is the C concentration; and h i (cm) is the soil depth. TN and TP storage were calculated in the same way as TC. Data were ln( x + 1) transformed before analysis to improve normality and reduce heteroscedasticity. Analysis of variance (ANOVA) was performed with habitat types (mounds of F. sanguinea , mounds of L. Flavus and control sites) and soil depth as the main fixed factors. LSD was used to compare treatment means. Comparison of the C, N and P pools m −2 in ant mounds and control soil were also performed with one-way ANOVA .The results were considered statistically significant at α < 0.05. All figures were drawn using the OriginPro 7.5 software. 3 Results 3.1 Nutrient contents in the mounds and control soil The C org and DOC concentrations in the control soil decreased with depth and the maximum values were found in the 0–5 cm layer, but the maximum values for L. flavus mounds were found in the10–15 cm layer and that of DOC in F. sanguinea mounds at 5–10 cm. Concentrations of C org (6.61–15.56%) and DOC (51.43–187.8 mg/kg) in F. sanguinea mounds were significantly greater than those in L. flavus mounds and the control soil ( F = 714.16, df = 2, p < 0.001 and F = 570.20, df = 2, p < 0.001), but no significant differences were observed between L. flavus mounds and the control soil ( Fig. 1 ). TN concentrations differed between ant mounds and the control soil ( F = 163.46, df = 2, p < 0.001), and TN concentrations in surface mound soil were lower than those in control soil, while higher in the subsurface soil. TN concentration values at 10-25 cm in F. sanguinea and L. flavus mounds were 5.6–7.9 and 4.3–4. 9 times higher than those in control soil, respectively; however, in the 0–5 cm soil layer, they were 0.7 and 0.4 times those in the control soil. For all soil layers (0–25 cm), the TN concentrations in F. sanguinea mounds were higher in comparison with the L. flavus mounds ( F = 163.46, df = 1, p < 0.001). TN concentrations in different layers only differed significantly in the control soil ( F = 58.85, df = 4, p < 0.001) ( Fig. 2 ). The two types of mounds and the control soil differed considerably in regard to NH 4 + ( F = 307.28, df = 2, p < 0.001) and NO 3 − ( F = 254.48, df = 2, p < 0.001) concentration profiles. The differences among both NH 4 + and NO 3 values in different soil layers were significant for F. sanguinea ( F = 1082.57, df = 4, p < 0.001; and F = 2028.12, df = 4, p < 0.001, respectively) while no significant differences between soil layers were found for L. flavus mounds and control soil. The higher values of NH 4 + and NO 3 − were obtained respectively at 5–20 cm and 0–15 cm depths in F. sanguinea mounds, and ranged 36.25–40.04 mg/kg and 2.17–4.36 mg/kg. The values of NH 4 + and NO 3 − throughout the L. flavus mounds were slightly lower than those of the control soil, although the differences were not statistically significant ( Fig. 2 ). On the whole, there were significant differences in TP and AP contents among ant mound and control soils ( F = 25.88, df = 2, p < 0.05; and F = 543.27, df = 2, p < 0.001, respectively). In the 0–10 cm layer, the order of TP concentrations was F. sanguinea > Control soil > L. flavus and at 10–25 cm the order was F. sanguinea > L. flavus > Control soil. The ant mounds contained more AP compared with the control soil, especially in the 5–25 cm layers. A significant depth effect was only observed forTP in the control soil profile ( F = 73.24, df = 4, p < 0.05) ( Fig. 3 ). The interactions between ant species and soil layer were all significant for TC ( F = 226.75, p < 0.001), DOC ( F = 143.82, p < 0.001), TN ( F = 7.67, p < 0.01), NH 4 + ( F = 307.39, p < 0.001), NO 3 − ( F = 335.77, p < 0.001) and AP ( F = 12.95, p < 0.01) concentrations but not for TP ( F = 2.37, p > 0.05). 3.2 C/N, C/P ratios in the mounds and control soil As shown in Table 2 , the C/N ratios in F. sanguinea mounds showed a tendency to decrease with increasing depth from 0 to 25 cm, while in L. flavus mounds and control soil it increased initially, reached a maximum at 10–15 cm, and then decreased. For the same soil layer, values of C/N ratios were lower in the mounds than in the control soil and there were no significant differences between F. sanguinea and L. flavus except in the 0–5 cm layer ( Table 2 ). The C/P ratios of F. sanguinea and L. flavus mounds showed the tendency of firstly increasing and then decreasing with soil depth and reached maximum values of 149.32 at 5–10 cm and 96.74 at 10–15 cm, respectively. However, the ratios dramatically decreased with depth for the control soil ( Table 2 ). In the same soil layers between 5 and 25 cm, values of the C/P ratio were higher in F. sanguinea mounds than in the control soil, while for the 0–5 cm layer the control soil had a higher C/P ratio than the mounds ( Table 2 ). 3.3 Nutrient pools in the mounds and control soil The soil bulk density values for ant mounds were lower than those for the control soil, except at 0–5 cm in L. flavus mounds ( Table 3 ). Carbon and DOC pools in L. flavus mounds were 8.09 kg m −2 and 9.09 g m −2 , respectively, significantly lower than in F. sanguinea mounds and the control soil ( F = 12.25, df = 2, p < 0.05) ( Fig. 4 ). However, no significant differences were observed for Carbon and DOC pools between F. sanguinea mounds and the control soil. As shown in Fig. 4 , TN (0.81 kg m −2 ) and NH 4 + (2.94 g m −2 ) values were considerably higher in F. sanguinea mounds than in the control soil ( F = 17.14, df = 1, p < 0.05; and F = 19.25, df = 1, p < 0.01, respectively), but the difference for NO 3 − was insignificant. The TN pool in L. flavus mounds was only slightly larger than in the control soil ( Fig. 4 ). The NH 4 + (0.64 g m −2 ) and NO 3 − (0.10 g m −2 ) values were much lower in L. flavus mounds compared with those of the control and F. sanguinea mound soils ( F = 64.58, df = 2, p < 0.01; and F = 15.74, df = 2, p < 0.05, respectively), which were 1.86 g m −2 and 0.23 g m −2 , respectively. Higher values were obtained for the TN, NH 4 + and NO 3 − pools in the F. sanguinea than in the L. flavus mounds. Regarding TP pools, there were no significant differences between ant mounds and control soil, but AP storage appeared considerably higher in mounds than in the control soil ( F = 39.82, df = 2, p < 0.01) ( Fig. 4 ). Higher values of AP occurred in F. sanguinea mounds compared with L. flavus mounds ( F = 26.25, df = 1, p < 0.05). 4 Discussion Data from this study confirm the effects of ant mounds on soil nutrients and their variability in wetland environments in Northeast China, in agreement with a few similar reports from other meadow wetland ecosystems ( Petal, 1980; Petal and Kusinska, 1994 ). Hence, attention should be paid to these structures when characterizing the nutrient properties of these meadow wetlands. As indicated by other authors ( Lobry de Bruyn and Conacher, 1990; Whitford and DiMarco, 1995; Holec and Frouz, 2006; Jiménez and Decaëns, 2006; Jiménez et al., 2008 ), our study showed that the effects of ant mounds on soil nutrient concentrations differed with ant species. Compared with other research on Formica and Lasius ant mounds ( Table 4 ), the C org values for F. sanguinea mounds in our site were lower while those for L. flavus mounds were significant higher. Previous studies on ant mounds of different species carried out in other ecosystems have also reported both lower and higher concentrations of C org and plant nutrients compared with control soils ( Table 4 ). In our study, the average concentrations of TN, TP and AP were higher in ant mounds than in control soil. These results are consistent with some previous reports on TN concentrations in Formica rufa mounds ( Risch et al., 2005 ), TN and TP contents in Pogonomyrmex barbatus nests ( Wagner et al., 2004 ), TP concentrations in Pogonomyrmex occidentalis and Pogonomyrmex rugosus nests ( Snyder et al., 2002 ), and AP contents in Lasisus niger nests ( Frouz et al., 2003; Holec and Frouz, 2006 ). Our results in regard to lower NH 4 + concentrations in L. flavus mounds than in control soil agree with those of Jiménez et al. (2008) . However, Lenoir et al. (2001) found that the TN concentration was lower in ant-nest material than in the surrounding soil. Beattie and Culver (1983) found no significant differences between NH 4 + and NO 3 − concentrations in nest material taken from the centre of F. canadensis nests and the surrounding soil. In addition, our results indicating that the vertical pattern of nutrient concentrations in mounds differs according to ant species is in agreement with previous studies ( Jiménez and Decaëns, 2006; Jiménez et al., 2008; Ginzburg et al., 2008; Hudson et al., 2009 ). The differences between ant mounds and the surrounding soil observed in our study are due, at least in part, to several factors depending on the species and how the mounds were built (e.g. difference in ant behavior, including feeding strategy and constructing activities), as other research has indicated ( Wagner et al., 2004; Kilpeläinen et al., 2007 ). In our study, relative higher C org and nutrient concentrations were found in F. sanguinea mounds than in L. flavus mounds and the surrounding soil ( Figs. 1–3 ). This was probably due to different feeding strategies. We observed that L. flavus mainly fed on honeydew from plant root aphids and live underground, while F. sanguinea are omnivorus invertebrates eating almost any plant litter and insects they can find in meadows. In our study site, the mound materials were selectively collected by F. sanguinea ants, and C. augustifolia litter was common mound-building material. More organic matter is concentrated in the top soil layers of F. sanguinea mounds than those of L. flavus mounds ( Table 1 ), which may lead to greater nutrient concentration in F. sanguinea mounds. In addition, inputs of other types of organic matter (e.g. high-protein plant tissues, seeds, feces and urine) to ant mounds as a direct results of ant foraging behavior often lead to higher C org and nutrient concentrations (e.g. TN, TP) ( Wagner et al., 2004 ). Our results show that ant mounds considerably increased the spatial variability of the chemical parameters C org , DOC, TN and TP, with the higher values in the subsurface soil, while nutrient content decreased with depth and the maximum values were observed in the surface 0–5 cm for the control soil. In L. flavus mounds the nutrient contents (e.g. C org , DOC and TP) were relatively lower than in the control soil. In agreement with previous studies ( Levan and Stone, 1983; Holec and Frouz, 2006 ), we postulate that soil transport from deep horizons and the mixing of upper organic and deeper mineral soil layers were responsible for these changes in the nutrient profile which were especially significant in L. flavus mounds. In meadow wetlands, organic matter, the principal source of C, N and P, is generally concentrated in the top soil layers and transport of this material to the subsurface would lead to the reduction of nutrient content in the surface layers and an increase in subsurface soil. In addition, ant mixing activities and soil translocations decreased the differences of TN and TP concentrations between soil layers for mound profiles, comparing with the control soil profiles. Mound building activities of ants also have other significant impacts on the physical and biological properties of soil, which may further lead to soil chemical variability. Chambers, soil aggregates, and maculae constructed in the soil may directly alter pore and particle size distribution with associated effects on moisture content, soil bulk density and aeration. We also found that the activities of ants when building mounds led to a tendency towards an increase in soil fine silt and clay particles and a decrease in soil moisture ( Wu et al., 2010 ) as Folgarait (1998) emphasized. Microbial activities in ant mounds are also strongly affected by soil moisture ( Holec and Frouz, 2006 ). In this study, the pH of ant mounds may have been shifted towards neutral values from the acidic state of the meadow wetland soil (pH 6.4–6.7) with saturated water conditions. The shift in pH to the neutral values observed in ant nests can increase nutrient (e.g. TP) availability ( Frouz et al., 2003 ). Previous studies ( Frouz et al., 1997; Laakso and Setälä, 1998; Dauber and Wolters, 2000; Ginzburg et al., 2008 ) have revealed that components of the microbial community were activated in different types of mounds compared with the surrounding soil. The present of ants also increased potential enzyme activities and altered the kinetic properties of the enzymes, and further significantly increased DOC, NH 4 + and NO 3 − in litter solutions compared to the control treatment in a greenhouse experiment ( Stadler et al., 2006 ). In addition, many arthropods (myrmecophiles) live in ant mounds as specialized inhabitants of refuse piles, brood or queen chambers, storage areas, or the bodies of ants ( Folgarait, 1998 ). Laakso and Setälä (1998) also found larger soil animal biomass in boreal wood ant ( Formica aquilonia ) mounds compared with surrounding soil. Thus the physical changes brought about by constructing activities of ants can affect soil biology and microbial activities by changing soil micro-habitats ( Folgarait, 1998; Stadler et al., 2006 ) and these may have an impact on soil chemical processes as discussed later. It is well recognized that mound-building ants affect vegetation heterogeneity and succession ( Ofer et al., 1982; Whitford and DiMarco, 1995; Farji-Brener and Silva, 1996; Farji-Brener and Medina, 2000; Moutinho et al., 2003 ). It is worth noting that there are often no wetland plants on F. sanguinea mounds in this study meadow, because the wet environment disappeared with the micro-topographical changes, especially in height, caused by ant mounds ( Table 1 ). The higher levels of N and P in the F. sanguinea mounds than in L. flavus mounds and control soil also might be due to the absence of plants on the mounds which is in agreement with Lenoir et al. (2001) . In addition, some previous research showed that ants can contribute to higher overland flow rates by modifying soil properties and reducing vegetation cover ( Cerdà and Jurgensen, 2008; Cerdà et al., 2009 ), or by providing preferential pathways for water into the wet subsoil ( Shakesby et al., 2007 ). In this study, we consider that the lack of vegetation cover, development of soil macropores and the accumulation of material highly susceptible to erosion could all accelerate rates of soil erosion and runoff from mounds. As discussed earlier, the C and nutrient concentrations were probably also influenced by changes in soil processes such as respiration, decomposition, mineralization and denitrification. Ants could have an accelerating effect on litter decomposition through direct physical effect and indirect trophic effects via aphids and micro-organisms ( Domisch et al., 2008 ). In this study, the relatively higher concentrations of N and P compared with C org in ant mounds led to lower values of C/N and C/P ratios. Low C/nutrient ratios usually indicate a fast decomposition rate ( Berg and McClaugherty, 2003 ). The data indicate that organic matter decomposition rates in both ant mounds were higher than those in the control soil, although in the F. sanguinea mounds C org concentrations showed an increase compared with the control soil, which might be due to the inputs of organic matter into mounds exceeding their decomposition. Previous research has also shown that carbon can be lost at higher rates relative to background soils from ant mounds through litter decomposition, C mineralization and CO 2 emissions ( Dauber and Wolters, 2000; Domisch et al., 2006; Risch et al., 2005 ). In this study, higher NH 4 + and NO 3 − contents indicate a high rate of N mineralization and nitrifying microorganisms seem to have been activated in F. sanguinea mounds because there was enough organic matter and suitable moisture conditions. However, L. flavus responds to taller vegetation by building larger mounds with soil excavated from deeper soil layers ( Dlussky, 1981; Blomqvist et al., 2000 ). There are fewer microorganisms in deeper soil horizons ( Alexander, 2005 ), and these may have different metabolic capacities from those at the soil surface which could have caused mineralization processes to be inhibited ( Amador and Görres, 2007 ). This could explain the lower concentrations of NH 4 + and NO 3 − in the L. flavus mounds. However, Verchot et al. (2003) found elevated NO 3 − concentrations deep in the soil profile of Atta sexdens nests, while neither net mineralization nor net nitrification were significantly greater at depth in the mineral soil of the nests. They suggested that the elevated NO 3 − may be caused by the surrounding N-rich organic matter buried by the ants in chambers within the deep soil. Further studies are required to assess the reasons for nutrient variation in ant mounds and associated structures, because these can be due to many factors. For example, higher levels of inorganic N in F. sanguinea mounds may result from enhanced resource availability and improved conditions for microorganisms involved in N mineralization, as well as a low rate of N uptake by plants. A detailed study on which microbial populations are enhanced or inhibited in these biogenic structures merits further investigation. In the present study, available P content in both types of ant mounds was significant higher than in surrounding soil ( Fig. 3 ), in agreement with previous studies ( Frouz et al., 2003; Holec and Frouz, 2006 ). We assume that, in addition to the possibility of higher mineralization of P in ant mounds, the amount of available P retained by the mounds was affected by the supply of available P in excreta and food residues as well as the sorption capacity of the nest substrate (due to a higher fine silt and clay particle contents than control soils), as similarly found by Frouz et al. (1997, 2003) . Soil nutrient pools are affected not only by nutrient concentrations but also by soil bulk density. Our results indicate that the effects of ant mounds on nutrient storage varied with ant species. In our study, C org and DOC pools in L. flavus mounds were lower than those in the control soil, while there were no significant differences between F. sanguinea mounds and control soil. The smaller C org and DOC pools in F. sanguinea mounds were due to the lower bulk densities compared with control soil ( Wu et al., 2010 ), while due to both lower concentrations and bulk densities for the L. flavus mounds. Risch et al. (2005) also found that red wood ant mound C pools were significantly lower than in the forest floor. Available P pools of ant mounds significantly higher than control soils were mainly due to the considerably higher P concentrations. Higher N pools in both types of ant mounds and higher NH 4 + and NO 3 − pools in F. sanguinea mounds agree with the findings of Kilpeläinen et al. (2007) . Wagner et al. (2004) also reported higher pools of TN, NH 4 + and NO 3 − in P. barbatus nests than those in control soil. In conclusion, the ant mounds considerably increased the spatial variability of chemical parameters and affected C/nutrient ratios and nutrient pools at the soil microscale. Our data also indicate that the direction and degree of these variations were related to ant species identity. The accumulation of ant biogenic structures may be an important potential driver of change for some wetland ecosystem processes and services at lager scales. In view of the high density of ant mounds in temperate meadow wetlands, the role of ant mounds thus deserves much more attention. Acknowledgements We thank Professor Zhenghui Xu for identification of the ant species. We also thank Dr. Beth Middleton for language corrections and some useful suggestions. This work was supported by the programs of National Natural Science Foundation of China (Nos. 40901036 and 40830535 ), Knowledge Innovation Programs of Chinese Academy of Sciences (Nos. KZCX2-YW-BR-16 and KZCX3-SW-NA09-01 ) and State Key Development Program for Basic Research of China (No. 2009CB421103 ). References Alexander, 2005 D.B. Alexander Bacteria and archaea D.M. Sylvia J.J. Fuhrmann P.G. Hartel D.A. Zuberer Principles and Applications of Soil Microbiology second ed. 2005 Pearson–Prentice-Hall Upper Saddle River, NJ 101 139 Amador and Görres, 2007 J.A. Amador J.H. Görres Microbiological characterization of the structures built by earthworms and ants in an agricultural field Soil Biol. Biochem. 39 2007 2070 2077 Beattie and Culver, 1983 A.J. Beattie D.C. Culver The nest chemistry of two seed-dispersing ant species Oecologia 56 1983 99 103 Berg and McClaugherty, 2003 B. Berg C. McClaugherty Plant Litter. Decomposition, Humus Formation, Carbon Sequestration 2003 Springer Verlag Berlin 286pp. Blomqvist et al., 2000 M.M. Blomqvist H. Olff M.B. Blaauw T. Bongers W.H. van der Putten Interactions between above- and belowground biota: importance for small-scale vegetation mosaics in a grassland ecosystem Oikos 90 2000 582 598 Boulton and Amberman, 2006 A.M. Boulton K.D. Amberman How ant nests increase soil biota richness and abundance: a field experiment Biodivers. Conserv. 15 2006 69 82 Cerdà and Jurgensen, 2008 A. Cerdà M.F. Jurgensen The influence of ants on soil and water losses from an orange orchard in eastern Spain J. Appl. Entomol. 132 2008 306 314 Cerdà et al., 2009 A. Cerdà M.F. Jurgensen M.B. Bodí Effects of ants on water and soil losses from organically-managed citrus orchards in eastern Spain Biologia 3 2009 527 531 Chen, 1996 G.Q. Chen Research on the wetlands in Sanjiang Plain 1996 Science Press Beijing pp. 27–30 Dauber and Wolters, 2000 J. Dauber V. Wolters Microbial activity and functional diversity in the mounds of three different ant species Soil Biol. Biochem. 32 2000 93 99 Dlussky, 1981 G.M. Dlussky Nester von Lasius flavus (Hymenoptera, Formicidae) Pedobiologia 21 1981 81 99 Domisch et al., 2006 T. Domisch L. Finér M. Ohashi A.C. Risch L. Sundström P. Niemelä M.F. Jurgensen Contribution of red wood ant mounds to forest floor CO 2 efflux in boreal coniferous forests Soil Biol. Biochem. 38 2006 2425 2433 Domisch et al., 2008 T. Domisch M. Ohashi L. Finér A.C. Risch L. Sundström J. Kilpeläinen P. Niemelä Decomposition of organic matter and nutrient mineralization in wood ant ( Formica rufa group) mounds in boreal coniferous forests of different age Biol. Fertil. Soils 44 2008 539 545 Farji-Brener and Medina, 2000 A.G. Farji-Brener C.A. Medina The importance of where to dump the refuse: seed banks and fine roots in nests of the leaf-cutting ants Atta cephalotes and A. colombica Biotropica 32 1 2000 120 126 Farji-Brener and Silva, 1996 A.G. Farji-Brener J.F. Silva Leaf-cutting ant Atta laevigata aid to the establishment success of Tapirira velutinifolia (Anacardiaceae) seedlings in a parkland savanna J. Trop. Ecol. 12 1996 163 168 Folgarait, 1998 P.J. Folgarait Ant biodiversity and its relationship to ecosystem functioning: a review Biodivers. Conserv. 7 1998 1221 1244 Frouz et al., 2003 J. Frouz M. Holec J. Kalcík The effect of Lasisus niger (Hymenoptera Formicidae) ant nest on selected soil chemical properties Pedobiologia 47 2003 205 212 Frouz and Kalčík, 1996 Frouz, J., Kalčík, J., 1996. The role of ants for availability of phosphorus in spruce plantation. In: Kalčík, J., Macháček, V. (Eds.), Proceeding of National Conference About Study of Phosphorus and Other Elements, Institute of Soil Biology, České Budějovice, pp. 75–80. Frouz et al., 1997 J. Frouz H. Šantrůčková J. Kalčík The effect of wood ants ( Formica polyctena Foerst.) on the transformation of phosphorous in a spruce plantation Pedobiologia 41 1997 437 447 Ginzburg et al., 2008 O. Ginzburg W.G. Whitford Y. Steinberger Effects of harvests ant ( Messor spp.) activity on soil properties and microbial communities in a Negev Desert ecosystem Biol. Fertil. Soils 45 2008 165 173 Hedde et al., 2005 M. Hedde P. Lavelle R. Joffre J.J. Jiménez Specific functional signature in soil macro-invertebrate biostructures Funct. Ecol. 19 2005 785 793 Holec and Frouz, 2006 M. Holec J. Frouz The effect of two ant species Lasius niger and Lasiu flavus on soil properties in two contrasting habitats Eur. J. Soil Biol. 42 2006 213 217 Hudson et al., 2009 T.M. Hudson B.L. Turner H. Herz J.S. Robinson Temporal patterns of nutrient availability around nests of leaf-cutting ants ( Atta colombica ) in secondary moist tropical forest Soil Biol. Biochem. 41 2009 1088 1093 Jiménez and Decaëns, 2006 J.J. Jiménez T. Decaëns Chemical variations in the biostructures produced by soil ecosystem engineers. Examples from the neotropical savannas Eur. J. Soil Biol. 42 2006 92 102 Jiménez et al., 2008 J.J. Jiménez T. Decaëns P. Lavelle C and N concentrations in biogenic structures of a soil-feeding termite and a fungus-growing ant in the Colombian savannas Appl. Soil Ecol. 40 2008 120 128 Jones et al., 1997 C.G. Jones J.H. Lawton M. Shachak Positive and negative effects of organisms as physical ecosystem engineers Ecology 78 1997 1946 1957 Jones et al., 1994 C.G. Jones J.H. Lawton M. Shachak Organisms as ecosystem engineers Oikos 69 1994 373 386 Jouquet et al., 2006 P. Jouquet J. Dauber J. Lagerlöf P. Lavelle M. Lepage Soil invertebrates as ecosystem engineers: intend and accidental effects on soil and feedback loops Appl. Soil Ecol. 32 2006 153 164 Kilpeläinen et al., 2007 J. Kilpeläinen L. Finér P. Niemelä T. Domisch S. Neuvonen M. Ohashi A.C. Risch L. Sundström Carbon, nitrogen and phosphorus dynamics of ant mounds ( Formica rufa group) in managed boreal forests of different successional stages Appl. Soil Ecol. 36 2007 156 163 Laakso and Setälä, 1998 J. Laakso H. Setälä Composition and trophic structure of detrital foodweb in ant nest mounds of Formica aquilonia and in the surrounding forest soil Oikos 81 1998 266 278 Lavelle, 1997 P. Lavelle Faunal activities and soil processes: adaptive strategies that determine ecosystem function Adv. Ecol. Res. 27 1997 93 132 Lavelle, 2002 P. Lavelle Functional domains in soils Ecol. Res. 17 2002 441 450 Lenoir et al., 2001 L. Lenoir T. Persson J. Bengtsson Wood ant nests as potential hot spots for carbon and nitrogen mineralization Biol. Fertil. Soils 34 2001 235 240 Levan and Stone, 1983 M.A. Levan E.L. Stone Soil modification by colonies of Black Meadow ants in a New York old field Soil Sci. Soc. Am. J. 47 1983 1192 1195 Liu and Ma, 2002 X.T. Liu X.H. Ma Natural Environmental Changes and Ecological Protection in the Sanjiang Plain 2002 Science Press Beijing Lobry de Bruyn and Conacher, 1990 L.A. Lobry de Bruyn A.J. Conacher The role of termites and ants in soil modification: a review Aust. J. Soil Res. 28 1990 55 93 Lu, 1999 R.K. Lu Analytical Methods for Soils and Agricultural Chemistry 1999 China Agricultural Science and Technology Press Beijing (in Chinese) Lu, 2009 X.G. Lu Wetland Biodiversity Change and Sustainable Use in the Sanjiang Plain 2009 Science Press Beijing Moutinho et al., 2003 P. Moutinho D.C. Nepstad E.A. Davidson Influence of leaf-cutting ant nests on secondary forest growth and soil properties in Amazonia Ecology 84 5 2003 1265 1276 Ofer et al., 1982 J. Ofer R. Ikan O. Haber Nitrogenous constituents in nest soils of harvester ants ( Messor ebeninus ) and their influence on plant growth Commun. Soil Sci. Plant Anal. 13 1982 737 747 Ohashi et al., 2007 M. Ohashi J. Kilpelä inen L. Finé r A.C. Risch T. Domisch S. Neuvonen P. Niemelä The effect of red wood ant ( Formica rufa group) mounds on root biomass, density, and nutrient concentrations in boreal managed forests J. For. Res. 12 2007 113 119 Petal, 1980 J. Petal Ant populations, their regulation and effect on soil in meadows Ekol. Pol. 28 1980 297 326 Petal and Kusinska, 1994 J Petal A. Kusinska Fraction composition of organic matter in the soil of anthills and of the environment of meadows Pedobiologia 38 1994 493 501 Risch et al., 2005 A.C. Risch M.F. Jurgensen M. Schütz D.S. Page-Dumroese The contribution of red wood ants to soil C and N pools and to CO 2 emissions in subalpine forests Ecology 86 2005 419 430 Shakesby et al., 2007 R.A. Shakesby P.J. Wallbrink S.H. Doerr P.M. English C.J. Chafer G.S. Humphreys W.H. Blake K.M. Tomkins Distinctiveness of wildfire effects on soil erosion in south-east Australian eucalypt forests assessed in a global context For. Ecol. Manage. 238 2007 347 364 Snyder et al., 2002 S.R. Snyder T.O. Crist C.F. Friese Variability in soil chemistry and arbuscular mycorrhizal fungi in harvester ant nests: the influence of topography, grazing and region Biol. Fertil. Soils 35 2002 406 413 Song et al., 2006 C.C. Song Y.S. Wang Y.Y. Wang Z.C. Zhao Emission of CO 2 , CH 4 and N 2 O from freshwater marsh during freeze–thaw period in Northeast of China Atmos. Environ. 40 2006 6879 6885 Stadler et al., 2006 B. Stadler A. Schramm K. Kalbitz Ant-mediated effects on spruce litter decomposition, solution chemistry, and microbial activity Soil Biol. Biochem. 38 2006 561 572 Verchot et al., 2003 L.V. Verchot P.R. Moutinho E.A. Davidson Leaf-cutting ant ( Atta sexdens ) and nutrient cycling: deep soil inorganic nitrogen stocks, mineralization, and nitrification in Eastern Amazonia Soil Biol. Biochem. 35 2003 1219 1222 Wagner et al., 2004 D. Wagner J.B. Jones D.M. Gordon Development of harvester ant colonies alters soil chemistry Soil Biol. Biochem. 36 2004 797 804 Whitford and DiMarco, 1995 W.G. Whitford R. DiMarco Variability in soils and vegetation associated with harvester ant ( Pogonomyrmex rugosus ) nests Biol. Fertil. Soils 20 1995 169 173 Wu et al., 2007 H.T. Wu X.G. Lu Y.Q. Jiang M.S.Z. Tong Early-stage litter decomposition and its influencing factors in the wetland of the Sanjiang Plain, China Acta Ecol. Sin. 27 2007 4027 4035 Wu et al., 2010 H.T. Wu D.H. Wu X.G. Lu X.M. Yin Spatial distribution of ant mounds and effects on soil physical properties in wetlands of the Sanjiang Plain, China Acta Ecol. Sin. 30 2010 270 275 Zacharov et al., 1981 A.A. Zacharov E.F. Ivanickova A.E. Maksimova Accumulation of elements in nests of red wood ants Pedobiologia 21 1981 36 45
更多查看译文
关键词
Ant mounds,Soil fauna,Nutrient dynamic,Heterogeneity,Wetland
AI 理解论文
溯源树
样例
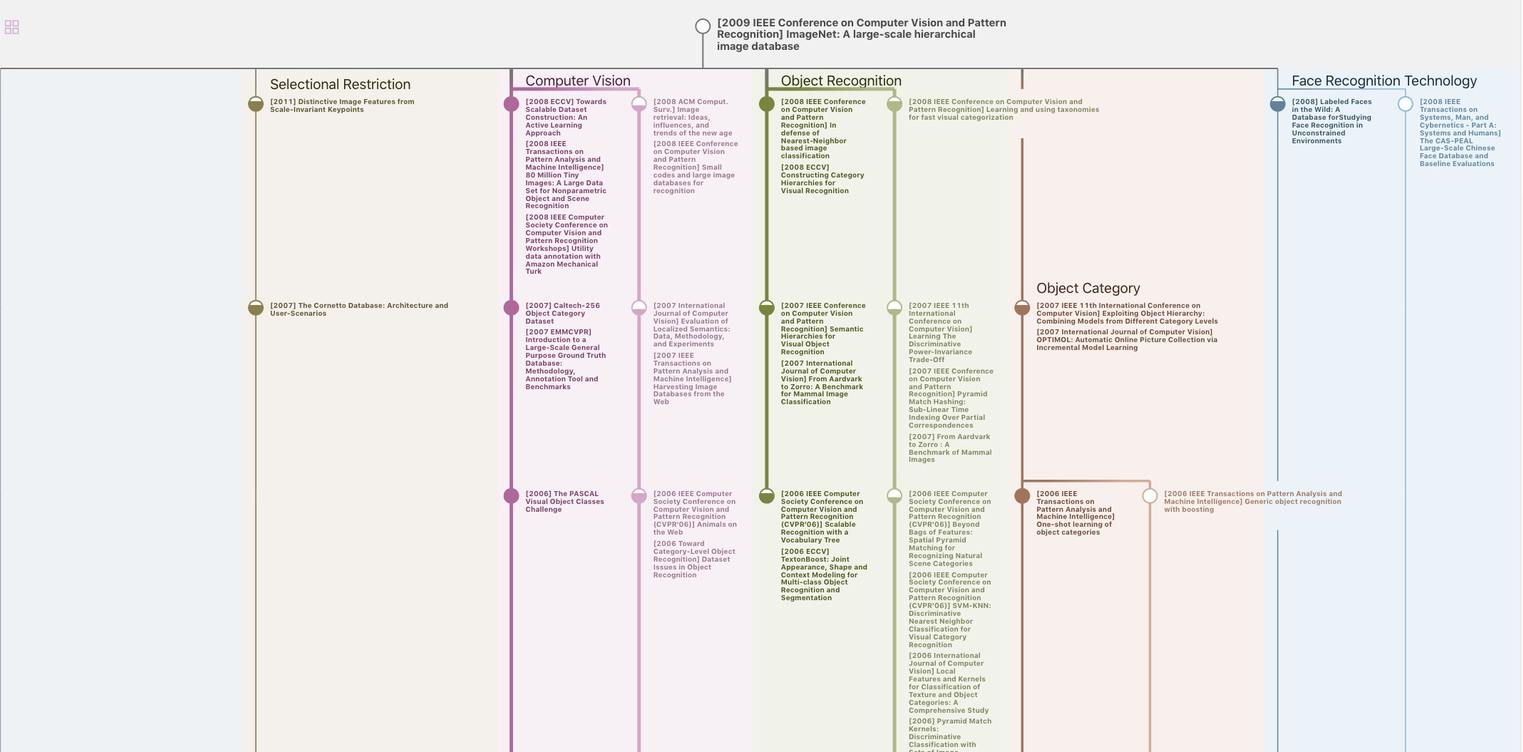
生成溯源树,研究论文发展脉络
Chat Paper
正在生成论文摘要