The relationship between body iron stores and blood and urine cadmium concentrations in US never-smoking, non-pregnant women aged 20–49 years
Environmental Research(2011)
摘要
Methods Serum ferritin, soluble transferrin receptor, urine and blood cadmium values in never-smoking, non-pregnant, non-lactating, non-menopausal women aged 20–49 years ( n= 599) were obtained from the 2003–2008 National Health and Nutrition Examination Surveys. Body iron was calculated from serum ferritin and soluble transferrin receptor, and iron deficiency defined as body iron <0 mg/kg. Robust linear regression was used to evaluate the relationships between body iron and blood and urine cadmium, adjusted for age, race, poverty, body mass index, and parity. Results Per incremental (mg/kg) increase in body iron, urine cadmium decreased by 0.003 μg/g creatinine and blood cadmium decreased by 0.014 μg/L. Iron deficiency was associated with 0.044 μg/g creatinine greater urine cadmium (95% CI=0.020, 0.069) and 0.162 μg/L greater blood cadmium (95% CI=0.132, 0.193). Conclusions Iron deficiency is a risk factor for increased blood and urine cadmium among never-smoking, pre-menopausal, non-pregnant US women, independent of age, race, poverty, body mass index and parity. Expanding programs to detect and correct iron deficiency among non-pregnant women merits consideration as a potential means to reduce the risk of cadmium associated diseases. Highlights ► Body iron was calculated from serum ferritin and soluble transferrin receptor. ► Body iron was inversely associated with blood and urine cadmium in US women. ► Inverse associations with blood cadmium were evident in all race/ethnic subsamples. ► Inverse associations with urine cadmium were evident in women of other/multi-race. ► Black women had lower mean body iron compared to white women. Keywords Cadmium Body iron Iron deficiency Women Never-smoking NHANES 1 Introduction Cadmium is a ubiquitous toxic heavy metal pollutant. Human exposures are primarily from food for the non-smoking general population ( ATSDR, 2008; EFSA, 2009 ). Among women, cadmium exposure is associated with increased risk of osteoporosis ( Akesson et al., 2006; Gallagher et al., 2008 ), breast cancer ( McElroy et al., 2006; Gallagher et al., 2010 ), and heart failure ( Peters et al., 2010 ), important causes of morbidity and mortality among women worldwide ( WHO, 2003, 2008 ). Cadmium uptake from the gastrointestinal tract is more efficient when iron stores are low ( Park et al., 2002; Min et al., 2008 ), and because pre-menopausal women tend to have lower iron stores compared to men, they may be at greater risk for increased cadmium accumulation ( Vahter et al., 2002; Nishijo et al., 2004 ) and susceptibility to cadmium-induced toxicities ( Vahter et al., 2007 ). Comparative interpretations of epidemiologic findings are limited by the use of different biomarkers of cadmium exposure and iron status. For example, Berglund et al. (1994) and Meltzer et al. (2010) showed that serum ferritin was inversely correlated with blood cadmium, and Akesson et al. (2002) found that urine cadmium increased from early gestation to lactation only among women with low iron status, as determined by transferrin receptor-serum ferritin ratio ( Akesson et al., 2002 ). Blood cadmium is considered the most valid biomarker of recent cadmium exposure ( Jarup and Akesson, 2009 ), with a half-life of 3–4 month ( Jarup et al., 1998 ). Urine cadmium is a biomarker of lifetime cadmium exposure ( Dillon and Ho, 1991 ), with a half-life of 30 years ( Satarug and Moore, 2004 ). Lower serum ferritin levels indicate reduced total body iron stores ( WHO, 2001 ), whereas increased soluble transferrin receptor levels indicate reduced tissue iron ( Skikne et al., 1990 ). Because the concentration of serum ferritin is influenced by infection and inflammation, the World Health Organization recommends assessing iron status by measuring both serum ferritin and soluble transferrin receptor, an indicator of red blood cell production and iron demand that is not significantly affected by infection or inflammation ( WHO, 2007 ). Limiting cadmium exposure studies to non-smoking subjects reduces exposure misclassification bias because cigarettes contain significant amounts of cadmium taken up by the tobacco plant and cigarettes also contain many other toxic compounds ( ATSDR, 2008 ). Compared to non-smokers, smokers have 4–5 times greater blood cadmium levels and 2–3 times greater kidney levels of cadmium ( Satarug and Moore, 2004 ); the latter is reflected by urine cadmium levels ( Dillon and Ho, 1991 ). Mijal and Holzman (2010) analyzed data from the 1999–2006 National Health and Nutrition Examination Surveys (NHANES) for never-smoking women and found a positive association between low ferritin (<20 ng/mL) and blood cadmium ≥0.50 μg/L. On the other hand, an analysis of NHANES III data found no significant correlations between urine cadmium and serum ferritin concentration among non-smoking men and women ( Choudhury et al., 2001 ). Contradictory findings from Scandinavian studies ( Olsson et al., 2002; Akesson et al., 2002 ) leave open questions about the relationship between iron status and urine cadmium. The relationships between body iron stores and both blood and urine cadmium have not been evaluated in non-pregnant, non-lactating, never-smoking women, overall or by race/ethnicity, using a measure of both serum ferritin and soluble transferrin receptor, as recommended by the WHO (2007) . Cogswell et al. (2009) applied the method of Cook et al. (2003) for estimating body iron as a derivative of ferritin and soluble transferrin receptor to an NHANES probability sample of non-pregnant women. They found that this estimate of body iron was less influenced by concomitant inflammation, predicted anemia more accurately, and resulted in lower estimates of iron deficiency than did the serum ferritin model based upon measures of serum ferritin, protoporphyrin and transferrin saturation ( Cogswell et al., 2009 ). Our primary objective was to evaluate whether body iron, as measured by Cook et al. (2003) and Cogswell et al. (2009) , is associated with urine and blood cadmium concentrations in non-pregnant, non-lactating, never-smoking, pre-menopausal US women, ages 20–49 years. Secondary objectives were to quantify associations between urine cadmium, blood cadmium and serum ferritin, and between urine cadmium, blood cadmium and body iron among race/ethnicity subgroups. 2 Methods 2.1 Study population Data on serum ferritin, soluble transferrin receptor, urine cadmium, urine creatinine, and blood cadmium were obtained for women aged 20–49 years from the 2003–2004, 2005–2006 and 2007–2008 National Health and Nutrition Examination Surveys (NHANES) ( CDC, 2003–2004, 2005–2006, 2007–2008a ). NHANES uses a complex, multistage, probability sampling design to select participants representative of the civilian, non-institutionalized US population ( CDC, 2009 ). Each sample person is assigned a sample weight that reflects adjustments for complex survey design (including oversampling), survey non-responses, and post-stratification, to ensure that calculated estimates are US population representative ( CDC, 2009 ). NHANES laboratory data on serum ferritin and transferrin receptor were available for women aged 12–49 years. The analytic sample included those women both with measurements of serum ferritin, transferrin receptor, blood cadmium, urine cadmium, urine creatinine, and without missing values for covariates poverty income ratio, body mass index, and parity. Subjects were limited to non-pregnant and non-lactating women ages 20–49, as associations between iron status, using a measure of both serum ferritin and transferrin receptor, and cadmium among pregnant and lactating women were previously reported ( Akesson et al., 2002 ). We further limited subjects to pre-menopausal women because this subpopulation is as at risk for low iron stores ( Vahter et al., 2002; Nishijo et al., 2004 ). Survey participants who reported they had ever smoked more than 100 cigarettes were excluded from the analytic sample in order to minimize exposure misclassification. Excluded from the resultant sample without missing values for the analytes and covariates of interest were 286 current or former smokers with a mean age of 34 years. There were 1536 survey participants excluded secondary to missing laboratory values or missing data for income, body mass index or parity, with a mean age of 32 years. 2.2 Body iron and iron deficiency variables Analyte concentrations were measured by the Division of Laboratory Sciences, National Center for Environmental Health, and Centers for Disease Controls and Prevention laboratory ( CDC, 2003–2004, 2005–2006, 2007–2008b ). Serum ferritin and transferrin receptor were obtained from a single blood sample and measured by immuno-turbidimetry with a Roche/Hitachi 912 clinical analyzer. Transferrin receptor values were converted to their equivalents in the Flowers assay, per Cogswell et al. (2009) : Flowers transferrin receptor =1.5×Roche transferrin receptor +0.35 mg/L. The formula of Cogswell et al. (2009) was used to calculate body iron, as follows: body iron (mg/kg)=−[log 10( transferrin receptor ×1000/ ferritin )−2.8229]/0.1207. This calculation results in a counter-intuitive negative value, i.e., <0 mg/kg for individuals with known iron deficiency; however, negative (and positive) values represent positive values for serum ferritin and transferrin receptor, as documented by repetitive phlebotomy in volunteer healthy adult subjects ( Cook et al., 2003 ). We also created dichotomous variables for low body iron as defined by Cogswell and colleagues (2009) , i.e., body iron <0 mg/kg. Serum ferritin and transferrin receptor values were natural log-transformed based upon an examination of scatterplots of the relationships between serum ferritin and transferrin receptor and blood cadmium and urine cadmium. 2.3 Blood and urine cadmium variables Blood cadmium (μg/L) and urine cadmium concentrations (ng/mL) from spot urine samples were measured by inductively coupled mass spectrometry by the Division of Laboratory Sciences, National Center for Environmental Health, and Centers for Disease Controls and Prevention laboratory ( CDC, 2003–2004, 2005–2006, 2007–2008b ). Levels of blood cadmium and urine cadmium below the detection limit were assigned a value of the detection limit divided by the square root of 2 ( CDC, 2003–2004, 2005–2006, 2007–2008b ). There were 47 urine cadmium and 174 blood cadmium values below the limits of detection 0.60 ng/mL and 0.20 μg/L, respectively. A creatinine-adjusted urine cadmium measure was generated by dividing urine cadmium (ng/mL) by urinary creatinine (mg/dL), also obtained from spot urine sample, and expressed as μg/g creatinine. 2.4 Demographic, anthropometric and reproductive history variables Race/ethnicity subgroups were defined as non-Hispanic white, non-Hispanic black, Mexican American and other (non-Hispanic other, Hispanic other, and multi-race). Based on the scientific literature regarding relevance to iron status, multivariable analysis included a continuous parity measure ( WHO, 2001 ) and a dichotomous measure for poverty ( WHO, 2001 ), particularly the poverty index ratio, i.e., family total income below the poverty threshold, as this variable showed a multi-modal distribution. A continuous variable for body mass index was also used as a non-specific indicator of nutritional status. In consideration of the scientific literature regarding relevance to cadmium accumulation, multivariable analysis also included continuous age in years ( ATSDR, 2008 ). 2.5 Statistical analysis Sample summary statistics represent unweighted data. The Rao–Scott chi-square test was used to evaluate statistical significance for the difference between weighted sample proportions of iron deficient and not iron deficient women by race/ethnicity. Significant differences in mean blood cadmium, urine cadmium and body iron values by race/ethnicity were calculated based upon analysis of weighted complex survey results. Linear associations between urine cadmium and blood cadmium in the unweighted data were evaluated using the Spearman correlation coefficient. Linear model assumptions were checked through visual inspection of scatterplots and residual plots. We used robust regression to address the possible influence of cadmium values below the limits of detection ( Roosli et al., 2008 ). Only unweighted results are presented for models with similar results in weighted analysis; otherwise, weighted and unweighted results are presented. 3 Results 3.1 Summary statistics Mean blood and urine cadmium levels for the overall sample were 0.302 μg/L and 0.242 μg/g creatinine, respectively, and 16% of all women were iron deficient ( Table 1 ). Iron deficiency was present in 23% of non-Hispanic black women, compared to 12% of non-Hispanic white and Mexican American women, and 13% of women of other race/ethnicity ( Table 2 ). Relative to non-Hispanic white women, non-Hispanic black women had significantly lower mean body iron and higher blood cadmium, and women of other race/ethnicity had both higher mean blood cadmium and urine cadmium ( Table 2 ). 3.2 Overall sample regression results for body iron, blood cadmium and urine cadmium Body iron was inversely associated with blood cadmium and urine cadmium ( Table 3 ); for every mg/kg increase in body iron, blood cadmium decreased by 0.0141 μg/L (95% CI=−0.0167, −0.0115) and urine cadmium decreased by 0.0029 μg/g creatinine (95% CI=−0.0050, −0.0007). Iron deficiency was associated with 0.1622 μg/L greater blood cadmium (95 CI=0.1317, 0.1927) and 0.0443 μg/g greater urine cadmium (95% CI=0.0196, 0.0690), adjusting for age, race/ethnicity, poverty, body mass index and parity ( Table 3 ). Age showed a significant positive association with blood cadmium and urine cadmium; however, body mass index and parity did not show significant associations. 3.3 Regression results by race/ethnicity for body iron, blood cadmium and urine cadmium Body iron was inversely associated with blood cadmium among all race/ethnic subsamples, with the largest magnitude of estimated effects among certain minority subsamples. Among non-Hispanic black women, for every mg/kg increase in body iron, blood cadmium decreased by 0.0199 μg/L (95% CI=−0.0261, −0.0137), and among women of other race/ethnicity, blood cadmium decreased by 0.0222 μg/L (95% CI=−0.0307, −0.0136) ( Table 3 ). The association between body iron and urine cadmium was statistically significant among women of other race/ethnicity, only ( Table 3 ). Scatterplot Figs. 1 and 2 (Supplementary Appendix) illustrate the inverse relationships between body iron, blood cadmium and urine cadmium across race/ethnicity subsamples, and highlight the relatively steeper slope for the relationship between body iron and urine cadmium among minority subsamples. 3.4 Regression results for serum ferritin, blood cadmium and urine cadmium The inverse relationship between serum ferritin and blood cadmium, but not urine cadmium, was robust in weighted and unweighted analyses, adjusted for the same covariates as the body iron model, plus log-transformed soluble transferrin receptor (Models 1 and 2, Table 4 ). Serum ferritin and transferrin receptor are inversely correlated in weighted ( r =−0.53) and unweighted ( r =−0.52) robust regression. Exclusion of the transferrin receptor covariate strengthened the effect estimates for blood cadmium in Models 3 and 4 and, although the effect estimates for the association between ferritin and urine cadmium remained similar to the models with transferrin receptor, results without transferrin receptor were statistically significant. Therefore, transferrin receptor may have statistically masked the influence of serum ferritin. Urine cadmium and blood cadmium were positively correlated, with Spearman rho=0.49. 4 Discussion 4.1 Relationships between body iron, serum ferritin, blood and urine cadmium We present novel findings of an inverse relationship between body iron and urine cadmium, and a positive association between body iron deficiency and urine cadmium, among never-smoking, non-pregnant, non-lactating US women aged 20–49 years. Consistent with previous findings using the NHANES III dataset ( Choudhury et al., 2001 ), we found no statistically significant evidence of an association between serum ferritin and urine cadmium in the model with soluble transferrin receptor; however, we did find an inverse association in the model without soluble transferrin receptor, and interpret this discrepancy as a result of statistical masking in the current study. The model that used the body iron measure, however, showed evidence of robustness to this masking effect, and may also reflect robustness to threats of biological validity and misclassification bias attributable to inflammation. Our findings of inverse relationships between body iron and blood cadmium, and between serum ferritin and blood cadmium, as well as a positive association between iron deficiency and blood cadmium, are consistent with findings of Mijal and Holzman (2010) . The absence of an association between parity and blood cadmium is another consistent finding. Also consistent with previous research is our finding of a positive correlation between blood and urine cadmium, although the magnitude of the correlation was somewhat lower than the coefficient of 0.56 reported by Akesson et al. (2006) ; the latter result may have been influenced by older age and inclusion of smokers. Findings of a positive correlation are also biologically supported, as a smaller fraction of blood cadmium can also reflect accumulated cadmium exposure, with a half-life of 10 years ( Jarup et al., 1998 ), and urine cadmium can also be influenced by short-term exposure ( ATSDR, 2008 ). 4.2 Disparities by race/ethnicity Variations by race/ethnicity in the levels of blood cadmium ( Mijal and Holzman, 2010 ) and serum ferritin ( Zacharski et al., 2000 ) were reported in US women; however, the current study findings regarding relationships between cadmium exposures and iron status among race/ethnic subgroups are novel. The inverse relationship between body iron and blood cadmium was evident across all race/ethnicity subgroups, with the greatest magnitude of effect estimates among black women, who also showed the highest percentage with iron deficiency, and among women of other race/ethnicity; however, only the latter subgroup showed a statistically significant inverse relationship between body iron and urine cadmium, most likely attributable to their higher urine cadmium levels. Determinants of iron deficiency among race/ethnic subgroups of women, however, are unclear ( Killip et al., 2007 ), and merit further research with larger sample sizes. 4.3 Study limitations and strengths The cross-sectional design of NHANES precludes determinations of the temporal relationships between body iron, blood cadmium and urine cadmium, as well as interpretations of causality. Iron deficiency has been shown, however, to upregulate divalent metal transporter 1 and increase cadmium absorption from the gastrointestinal tract with subsequent distribution to the liver and kidney in rats ( Park et al., 2002 ) and in mice ( Min et al., 2008 ). A potential study limitation is the lack of information regarding zinc deficiency, which may increase the risk for cadmium-related disease ( Reeves and Chaney, 2008 ). Because elevated serum ferritin ( WHO, 2007 ), cadmium ( Lin et al., 2009 ), and zinc deficiency ( Prasad, 2008; Bao et al., 2010 ) each may be associated with inflammation, lack of inflammatory biomarker data may confound our analysis of the relationships among these parameters. A strength of the current study is the use of a measure of iron deficiency that incorporates both serum ferritin and soluble transferrin receptor, minimizing confounding effects of inflammation and providing greater specificity for iron status. In addition, among females aged 12–49 years Cogswell et al. (2009) found good agreement between iron deficiency estimates based upon the body iron measure used in this study and estimates calculated based upon the ferritin model that defines iron deficiency as an abnormal value for at least 2 of 3 indicators (serum ferritin, erythrocyte protoporphyrin, and transferrin saturation). As mentioned in the study by Cogswell et al. (2009) , additional limitations of the general use of the calculation of body iron include the assumption that soluble transferrin receptor and serum ferritin assays remain stable over time, the lack of information on laboratory and clinical findings used to confirm iron deficiency, and the fact that the estimate of body iron developed by Cook et al. (2003) was derived from a study of 14 healthy adult white subjects ( Skikne et al., 1990 ). Further, a possible source of exposure misclassification is the use of creatinine-adjusted spot urine samples ( Barr et al., 2005; Gunier et al., 2010 ); however, we also conducted analysis using multivariable adjustment for urine creatinine, and results were similar (not shown). 4.4 Conclusion This study’s finding that body iron is inversely related to blood and urine cadmium among never-smoking US women, and the finding that body iron deficiency is associated with both increased blood and urine cadmium suggests that iron deficiency may be a risk factor for increased cadmium accumulation among never-smoking, non-pregnant women. Consequently, iron deficiency may be a risk factor for diseases associated with elevated cadmium levels, such as osteoporosis ( Akesson et al., 2006; Gallagher et al., 2008 ), renal tubular dysfunction ( Akesson et al., 2005 ), cardiovascular disease ( Peters et al., 2010 ), endometrial cancer ( Akesson et al., 2008 ), and breast cancer ( McElroy et al., 2006; Gallagher et al., 2010 ). Our finding that this relationship is independent of parity leads us to hypothesize that correction of iron deficiency among non-pregnant women would be expected to reduce cadmium body burden and, therefore, reduce their risk of cadmium-associated diseases. Appendix A Supplementary materials Supplementary data associated with this article can be found in the online version at doi:10.1016/j.envres.2011.03.007 . Appendix A Supplementary materials Fig. S1 Scatterplot of blood cadmium (μg/L) by body iton (mg/kg) by race/ethnicity. Fig. S2 Scatterplot of urine cadmium (μg/g creatinine) by body iton (mg/kg) by race/ethnicity. References Akesson et al., 2002 A. Akesson M. Berglund A. Schutz P. Bjellerup K. Bremme M. Vahter Cadmium exposure in pregnancy and lactation in relation to iron status Am. J. Public Health 92 2002 284 287 Akesson et al., 2005 A. Akesson T. Lundh M. Vahter P. Bjellerup J. Lidfeldt C. Nerbrand G. Samsioe U. Strombert S. Skerfving Tubular and glomerular kidney effects in Swedish women with low environmental cadmium exposure Environ. Health Perspect. 113 2005 1627 1631 Akesson et al., 2006 A. Akesson P. Bjellerup T. Lundh J. Lidfeldt C. Nerbrand G. Samsioe S. Skerfving M. Vahter Cadmium-induced effects on bone in a population-based study of women Environ. Health Perspect. 114 2006 830 834 Akesson et al., 2008 A. Akesson B. Julin A. Wolk Long-term dietary cadmium intake and postmenopausal endometrial cancer incidence: a population-based prospective cohort study Cancer Res. 68 2008 6435 6441 ATSDR, 2008 ATSDR (Agency for Toxic Substances & Disease Registry), 2008. Toxicological Profile for Cadmium, September 2008. Available online at: 〈 http://www.atsdr.cdc.gov/ToxProfiles/TP.asp?id=48&tid=15 〉 (accessed 11.17.10). Bao et al., 2010 S. Bao M.J. Liu B. Lee B. Besecker J.P. Lai D.C. Guttridge D.L. Knoell Zinc modulates the innate immune response in vivo to polymicrobial sepsis through regulation of NF-kappaB Am. J. Physiol. Lung Cell Mol. Physiol. 298 2010 L744 754 Barr et al., 2005 D.B. Barr L.C. Wilder S.P. Caudill A.J. Gonzalez L.L. Needham J.L. Pirkel Urinary creatinine concentrations in the U.S. population: implications for urinary biologic monitoring measurements Environ. Health Perspect. 113 2005 192 200 Berglund et al., 1994 M. Berglund A. Akesson B. Nermell M. Vahter Intestinal absorption of dietary cadmium in women depends on body iron stores and fiber intake Environ. Health Perspect. 102 1994 1058 1066 CDC, 2003–2004, 2005–2006, and 2007–2008a CDC (Centers for Disease Control and Prevention), 2003–2004, 2005–2006, and 2007–2008a. National Center for Health Statistics (NCHS). National Health and Nutrition Examination Survey Data, US Department of Health and Human Services, Centers for Disease Control and Prevention, Hyattsville, MD, 〈 http://www.cdc.gov/nchs/nhanes/nhanes_questionnaires.htm 〉 (accessed 6.06.10). CDC, 2003–2004, 2005–2006, and 2007–2008b CDC (Centers for Disease Control and Prevention), 2003–2004, 2005–2006, and 2007–2008b. National Center for Health Statistics (NCHS). National Health and Nutrition Examination Survey Laboratory Protocol, US Department of Health and Human Services, Centers for Disease Control and Prevention. Hyattsville, MD, 〈 http://www.cdc.gov/nchs/nhanes/nhanes_questionnaires.htm 〉 (accessed 6.05.10). CDC, 2009 CDC (Centers for Disease Control and Prevention), 2009. National Center for Health Statistics (NCHS). Continuous NHANES Web Tutorial, US Department of Health and Human Services, Centers for Disease Control and Prevention. Hyattsville, MD, 〈 http://www.cdc.gov/nchs/tutorials/index.htm 〉 (accessed 11.17.10). Choudhury et al., 2001 H. Choudhury T. Harvey W.C. Thayer T.F. Lockwood W.M. Stiteler P.E. Goodrum J.M. Hassett G.L. Diamond Urinary cadmium elimination as a biomarker of exposure for evaluating a cadmium dietary exposure—biokinetics model J. Toxicol. Environ. Health 63 2001 321 350 Cogswell et al., 2009 M.E. Cogswell A.C. Looker C.M. Pfeiffer J.D. Cook D.A. Lacher J.L. Beard S.R. Lynch L.M. Grummer-Strawn Assessment of iron deficiency in US preschool children and non-pregnant females of childbearing age: National Health and Nutrition Examination Survey 2003–2006 Am. J. Clin. Nutr. 89 2009 1334 1342 Cook et al., 2003 J.D. Cook C.H. Flowers B.S. Skikne The quantitative assessment of body iron Blood 101 2003 3359 3364 Dillon and Ho, 1991 H.K. Dillon M.H. Ho Biological Monitoring of Exposure to Chemicals: Metals 1991 John Wiley & Sons New York/Chichester/Brisbane EFSA, 2009 EFSA (European Food Safety Authority) 2009. Scientific Opinion, Cadmium in food. The EFSA Journal 980, 1–139. 〈 http://www.efsa.europa.eu/en/scdocs/scdoc/980.htm 〉 (accessed 11.17.10). Gallagher et al., 2008 C.M. Gallagher J.S. Kovach J.R. Meliker Urinary cadmium and osteoporosis in U.S. women >or=50 years of age: NHANES 1988–1994 and 1999–2004 Environ. Health Perspect. 116 2008 1338 1343 Gallagher et al., 2010 C.M. Gallagher J.J. Chen J.S. Kovach Environmental cadmium and breast cancer risk Aging 2 2010 1 11 Gunier et al., 2010 Gunier, R., Rull, R., Hertz, A., Canchola, A., Horn-Ross, P., Renolds, P. 2010. Urinary cadmium concentrations among female teachers from Northern California. [Abstract] No. 0824. Poster Presented at the 2010 Joint ISES-ISEE Conference, Seoul, Korea, August 28–September 1, 2010. Jarup et al., 1998 L. Jarup M. Berglund C.G. Elinder G.I. Nordberg M. Vahter Health effects of cadmium exposure—a review of the literature and a risk estimate Scand. J. Work Environ. Health 24 1998 1 51 Jarup and Akesson, 2009 L. Jarup A. Akesson Current status of cadmium as an environmental health problem Toxicol. Appl. Pharmacol. 238 2009 201 208 Killip et al., 2007 S. Killip J.M. Bennett M.D. Chambers Iron deficiency anemia Am. Fam. Physician 75 2007 671 678 Lin et al., 2009 Y.S. Lin D. Rathod W.C. Ho J.J. Caffrey Cadmium exposure is associated with elevated blood C-reactive protein and fibrinogen in the U.S. population: the third national health and nutrition examination survey (NHANES III, 1988–2994) Ann. Epidemiol. 19 2009 592 596 McElroy et al., 2006 J.A. McElroy M.M. Shafer A. Trentham-Dietz J.M. Hampton P.A. Newcomb Cadmium exposure and breast cancer risk J. Natl. Cancer Inst. 98 2006 869 873 Meltzer et al., 2010 H.M. Meltzer A.L. Brantsaeter B. Borch-Johnsen D.G. Ellingsen J. Alexander Y. Thomassen H. Stigum T.A. Ydersbond Low iron stores are related to higher blood concentrations of manganese, cobalt and cadmium in non-smoking, Norwegian women in the HUNT 2 study Environ. Res. 110 2010 497 504 Mijal and Holzman, 2010 R.S. Mijal C.B. Holzman Blood cadmium levels in women of childbearing age vary by race/ethnicity Environ. Res. 110 2010 505 512 Min et al., 2008 K.S. Min N. Iwata N. Tetsutikawahara S. Onosaka K. Tanaka Effect of hemolytic and iron-deficiency anemia on intestinal absorption and tissue accumulation of cadmium Toxicol. Lett. 179 2008 48 52 Nishijo et al., 2004 M. Nishijo S. Satarug R. Honda I. Tsuritani K. Aoshima The gender differences in health effects of environmental cadmium exposure and potential mechanisms Mol. Cell. Biochem. 255 2004 87 92 Olsson et al., 2002 I.M. Olsson I. Bensryd T. Lundh H. Ottosson S. Skerfving A. Oskarsson Cadmium in blood and urine—impact of sex, age, dietary intake, iron status, and former smoking—association of renal effects Environ. Health Perspect. 110 2002 1185 1190 Park et al., 2002 J.D. Park N.J. Cherrington C.D. Klaassen Intestinal absorption of cadmium is associated with divalent metal transporter 1 in rats Toxicol. Sci. 68 2002 288 294 Peters et al., 2010 J.L. Peters T.S. Perlstein M.J. Perry E. McNeely J. Weuve Cadmium exposure in association with history of stroke and heart failure Environ. Res. 110 2010 199 206 Prasad, 2008 A.S. Prasad Zinc in human health: effect of zinc on immune cells Mol. Med. 14 2008 353 357 Reeves and Chaney, 2008 P.G. Reeves R.L. Chaney Bioavailability as an issue in risk assessment and management of food cadmium: a review Sci .Total Environ. 398 2008 13 19 Roosli et al., 2008 M. Roosli P. Frei E. Mohler C. Braun-Fahrlander A. Burgi J. Frohlich Statistical analysis of personal radiofrequency electromagnetic field measurements with nondetects Bioelectromagnetics 29 2008 471 478 Satarug and Moore, 2004 S. Satarug M.R. Moore Adverse health effects of chronic exposure to low-level cadmium in foodstuffs and cigarette smoke Environ. Health Perspect. 112 2004 1099 1103 Skikne et al., 1990 B.S. Skikne C.H. Flowers J.D. Cook Serum transferrin receptor: a quantitative measure of tissue iron deficiency Blood 75 1990 1870 1876 Vahter et al., 2002 M. Vahter M. Berglund A. Akesson C. Liden Metals and women’s health Environ. Res. 88 2002 145 155 Vahter et al., 2007 M. Vahter A. Akesson C. Liden S. Ceccatelli M. Berglund Gender differences in the disposition and toxicity of metals Environ. Res. 104 2007 85 95 World Health Organization (WHO), 2001 World Health Organization (WHO), 2001. Iron Deficiency Anaemia, Assessment, Prevention and Control, a Guide for Programme Managers. WHO/NHD/01.3. 〈 http://www.who.int/nutrition/publications/micronutrients/anaemia_iron_deficiency/WHO_NHD_01.3/en/index.html 〉 (accessed 11.17.10). World Health Organization (WHO), 2003 World Health Organization (WHO), 2003. Prevention and Management of Osteoporosis: A Report of a WHO Scientific Group. WHO Technical Report Series, 921. 〈 http://whqllibdoc.who.int/trs/who_trs_921.pdf 〉 (accessed 11.17.10). World Health Organization (WHO), 2007 World Health Organization (WHO), 2007. Assessing the iron status of populations. Second edition, Including literature reviews. Joint World Health Organization/ Centers for Disease Control and Prevention Technical Consultation on the Assessment of Iron Status at the Population Level, 2004, Geneva, Switzerland. 〈 http://www.who.int/nutrition/publications/micronutrients/anaemia_iron_deficiency/9789241596107/en/index.html (accessed 11.17.10). World Health Organization (WHO), 2008 World Health Organization (WHO), 2008. The Global Burden of Disease: 2004 update. 〈 http://www.who.int/healthinfo/global_burden_disease/2004_report_update/en/index.html 〉 (accessed 11.17.10). Zacharski et al., 2000 L.R. Zacharski D.L. Ornstein S. Woloshin L.M. Schwartz Association of age, sex, and race with body iron stores in adults: analysis of NHANES III data Am. Heart J. 140 2000 98 104
更多查看译文
关键词
Cadmium,Body iron,Iron deficiency,Women,Never-smoking,NHANES
AI 理解论文
溯源树
样例
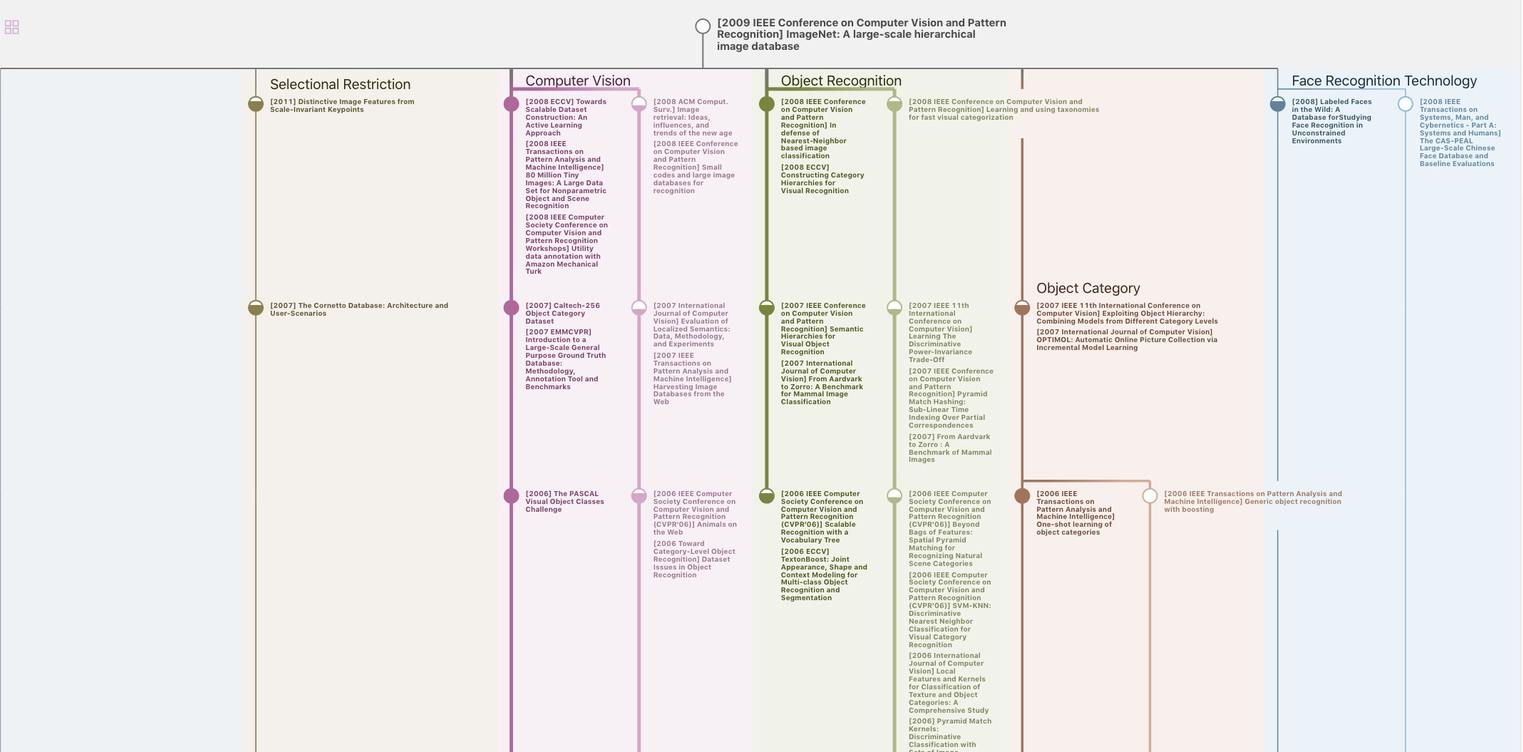
生成溯源树,研究论文发展脉络
Chat Paper
正在生成论文摘要