Ecuador, Peru And Bolivia
QUATERNARY GLACIATIONS - EXTENT AND CHRONOLOGY: A CLOSER LOOK(2011)
摘要
A synthesis of published paleoglacial research and cartographic overview of landscape features in Ecuador, Peru and Bolivia indicate three broadly synchronous glacial advances: two during MIS 2, culminating at 25–20 and ~ 16 ka, and one at 13.0–12.5 ka, possibly associated with the Younger Dryas chron. Keywords Tropical Andes Younger Dryas paleoglaciers cosmogenic radiocarbon 56.1 Introduction The development of an accurate glacial chronology for sites in Ecuador, Peru and Bolivia is important for understanding factors that force glacial change in the Andes as well as the linkages between tropical and global climate systems ( Klein et al . , 1999; Hastenrath, 2009 ). Because tropical mountains tend to experience high precipitation but minimal thermal seasonality, tropical glaciers exhibit different mass-balance behaviour than glaciers at higher latitudes. Their distinctive ablation zone environments make tropical glacier termini highly responsive to climatic variation ( Kaser and Osmaston, 2002; Smith et al . , 2008 ). As such, the glacial geomorphology of tropical mountains can serve as a strong indicator of past climatic fluctuation ( Seltzer, 1990; Heine, 2000; Licciardi et al . , 2009 ). Mark et al . (2004) reviewed the state of palaeoglacial knowledge for Ecuador, Peru and Bolivia in the first edition of this volume. This chapter synthesises information from that review with more recent research and provides a more comprehensive cartographic overview of mapped moraines in this portion of the Andes. The past 5 years have been rich in both new moraine dating studies and studies that revisit previously published age estimates in key localities. Further, two comprehensive overview papers have been published in the past 2 years: Smith et al . (2008) examined palaeoglaciation in Ecuador, Peru and Bolivia from Marine Isotope Stage (MIS) 5e (132–117 ka) through the Younger Dryas chron (12.9–11.7 ka), while Rodbell et al . (2009) focused on Andean glacial chronologies referencing the Late-glacial and Holocene (16.7 ka–present). Readers are referred to these two papers for a more detailed discussion than that which is presented here. All relevant features of palaeoglacial chronologies included on maps within the literature described in this chapter have been digitised and incorporated into an ArcGIS geodatabase. This database includes 1550 individual mapped moraines and 350 sampling locations, as well as the present glacial extent (generally as represented on the original maps) and the location of lakes utilised for sediment coring. Maps produced from this geodatabase accompany this chapter. The original maps were georeferenced using the coordinate grid provided on the map and/or by matching features on Google Earth imagery. Individual features were then hand-digitised and attributed based on information both on the maps and in the text. The shaded relief background utilised on the maps created for this chapter is derived from 30-m resolution digital elevation models (DEMs) produced by the Advanced Spaceborne Thermal Emission and Reflection radiometer (ASTER) optical sensor (ASTER Global DEM is a product of the Japanese Ministry of Economy, Trade and Industry (METI) and NASA). Some ASTER DEMs are prone to ‘pitting’—artificial sinks in the elevation model. Where pitting is pervasive, 90-m resolution DEMs produced by the NASA Shuttle Radar Topography Mission (SRTM) have been used instead. In some instances, moraines digitised from the original maps have been shifted slightly to better match features identifiable on Google Earth imagery or the topographic surfaces generated from the DEMs. While every effort has been made to represent reality as closely as possible, the complexities of georeferencing and the uncertain ground accuracy of the original maps do not allow use of this geodatabase at small mapping scales. The literature reviewed in this chapter primarily utilises radiocarbon or surface exposure dating (SED) methods ( Fig. 56.1 ). In both cases, adjustment of calculated values is necessary in order to estimate the true calendar age of the sample. Because the production rate of 14 C has varied through time, radiocarbon dates must be calibrated using dendrochronologically derived curves while scaling factors must be applied to surface exposure dates calculated from cosmogenic radio-nuclides (CRN) such as 10 Be, 36 Cl and 3 He when samples are obtained at low latitudes and/or high elevations. Because there are multiple scaling methods in use, a range of age interpretations can exist for single feature. At this time, no single universally accepted scaling method has been identified, though the CRONUS-Earth Project initiative is aimed at synthesising existing scaling methods and producing consistent calibration dates ( Balco et al . , 2008 ). All radiocarbon dates reported in this chapter are calibrated years before present (cal. ka BP, with BP as AD 1950) unless otherwise indicated. Rodbell et al . (2009) provided calibrated radiocarbon dates for numerous Andean sites where these dates were not initially reported, and these are used here. In other cases, calibration calculations are made using Calib version 5.0 ( Stuiver and Reimer, 1993 ). Readers are referred to the original literature for the original 14 C dates. CRN dates reported in this chapter are those provided in the original literature, though instances where revised calculations have been made by other authors are noted. Rodbell et al . (2009) have calibrated the results of several tropical Andean SED studies using the CRONUS calculator and include a useful table of correlated values for several of the papers reviewed here. In both this chapter and the accompanying ArcGIS geodatabase, glacial chronologies are defined by their correlative MISs ( Fig. 56.2 ). Based on sea-level lowstands, the global Last Glacial Maximum (LGM) is defined herein as 22–19 ka ( Yokoyama et al . , 2000 ); in the tropical Andes, the LGM has been situated at 21 ± 2 ka ( Mark et al . , 2005; Smith et al . , 2008 ). The transition between MIS 2 and MIS 1 occurs between 11.4 and 12.1 ka ( Martinson et al . , 1987 ), and the base of the Holocene has recently been placed at 11.7 ka using the Greenland NGRIP ice core ( Walker et al., 2009 ). Following the convention of Rodbell et al . , 2009 , the Late-glacial is defined in this chapter as 16.7–11.7 ka. 56.2 Geographical setting The Andes span the entire western flank of the South American continent. Within Ecuador, Peru and Bolivia, the Andes stretch for over 3000 km and contain an estimated 2650 km 2 of glacial ice, ~ 95% of the glaciers presently existent within the tropical latitudes ( Kaser and Osmaston, 2002 ). This portion of the Andes comprises a collection of individual ranges that are generally grouped into two parallel cordilleras—the Cordillera Oriental and the Cordillera Occidental—with a high plateau between the two. In southern Peru and Bolivia, this plateau, known as the Altiplano, is more than 200 km wide. In Ecuador, the two cordilleras generally reach elevations between 4000 and 4400 m but are punctuated by seven stratovolcanoes in excess of 5000 m and an eighth (Volcán Chimborazo) that reaches 6000 m ( Smith et al . , 2008 ). In Peru and Bolivia, extensive sections of each cordillera exceed 5000 m, with numerous individual peaks topping 6000 m, particularly in the ranges of the Cordillera Occidental, while the Altiplano averages 3500–4000 m ( Smith et al . , 2008 ). At present, glacial limits in the tropical Andes are ~ 5000 m, though terminus elevations are somewhat lower in the Cordillera Blanca (4700 m; Mark, 2008 ), Cordillera Huayhuash (4800 m; Hall et al . , 2009 ) and Cordillera Vilcanota (4600 m; Mercer and Palacios, 1977 ) of Peru. The Quelccaya Ice Cap in southern Peru is the only remaining such glacial feature in the Earth's tropical latitudes. 56.3 Tertiary and Early Pleistocene glaciations (> MIS 19; > 790 ka) There is scant documented evidence of tropical Andean glaciers from this Early in geologic history. It is estimated that the Bolivian Andes were no more than 50% of their present altitude 10 Ma and that the Peruvian Andes have attained most of their present elevation in only the past 5–6 Ma ( Smith et al . , 2008 ), suggesting that the range may have been of insufficient altitude to support glaciation at this latitude prior to these dates. Clapperton (1983) described the 1962 research of Earnest Dobrovolny, who identified a pair of glacial tills near La Paz, Bolivia that attest to very old palaeoglaciations. One, a 7-m thick till, is overlain by an ignimbrite formation dated to ~ 3.25 Ma. The second till is highly oxidised, yet buried well beneath two other tills associated with the most recent two glacial cycles. The depth of the oxidation suggests a considerable lag between the deposition of this till and those above it. No specific glacial stadial is hypothesised for this material. 56.4 Middle Pleistocene glaciations (MIS 19–6; 790–132 ka) Heine (1995) and Heine and Heine (1996) describe the sequence of moraines on Rucu Pichincha, Ecuador (0.2°S, 78.5°W; Fig. 56.3 ), and classify these into seven distinct groups, M1–M7, sequentially numbered from oldest to youngest. Groups M1–M3 pre-date the last glacial cycle (MIS 4–2), though precise dating control is lacking. M1, the oldest, is underlain by a lava formation dated to greater than 900 ka. On Rucu Pichincha, no limiting age for these moraines has been determined, however correlative sequences have been identified on the Potrerillos Plateau (0.3°S, 78.2°W; Fig. 56.4 A and B), where a lava flow inside the valley carved by the M1 glaciation is dated to > 150–180 ka provides a minimum-limiting age for the M1 and M2 sequences ( Heine, 1995; Smith et al . , 2008 ). On Rucu Pichincha, M1 moraines occur at 3550–3600 m, with M2 moraines located in close proximity. M3 moraines are only identified in two locations, at elevations as low as 3700 m. On the Chimborazo–Carihuairazo massif (1.4°S, 78.8°W; Fig. 56.5 A and B), there are no moraines interpreted as dating to prior to MIS 2; however, there is other geomorphic evidence for earlier and potentially more extensive glacial events. Clapperton and McEwan (1985) described lateral moraines at 3600 m in the Rio Mocha Valley between the two volcanoes that represent the local MIS 2 maximum extent of glaciation, but note that these moraines appear too small for the deeply eroded glacial valley in which they have been deposited, suggesting an earlier glaciation. The authors also described the presence of oxidised tills outside the limits of MIS 2 moraines. Clapperton (1986) (reported in Smith et al., 2008 ) placed these tills as low as 2750 m and noted that this is the lowest altitude at which glacial deposits have been identified in Ecuador. Clapperton (1990) described the presence of highly weathered glacial deposits inter-bedded with volcanic material on Carihuairazo and suggested that these may date from as early as MIS 8 based on rates of weathering and oxidation relative to a separate till constrained by a minimum-limiting 14 C date of > 43 ka. Lack of dating control makes the age of this till uncertain. Advancing the work of Clapperton (1981), Rodbell (1993a) measured rock weathering and soil development, and used radiocarbon dating from lake/bog sediments to define four pre-Holocene moraine sequences in the Cordillera Blanca (9.3°S, 77.5°W; Fig. 56.6 A–F). The oldest of these four sequences, the Cojup, was assigned one of three potential date ranges based on either extrapolation of rock weathering or Profile Development Index (PDI) of moraine soils, the youngest being 46–29 ka, while the oldest was > 4.3 Ma. Farber et al . (2005) revisited the age classifications of Rodbell, using 10 Be SED to improve the age precision of these features. Based on this work, the authors suggested a date range of 440–120 ka and speculated that the wide range of dates associated with Cojup boulders in their study may actually be indicative of two separate glacial advances. Only small isolated remnants of Cojup moraines are found in the Cordillera Blanca, at elevations as low as 3650 m ( Rodbell, 1993a ). Further evidence of Middle Pleistocene glaciation in the tropical Andes is found in the Lima Conglomerate, a unit deposited in sediment fans along Peru's Pacific Coast littoral. Le Roux et al . (2000) identified distinct cycles of coarse and fine material within the conglomerate and suggested that they related to variations in river discharge resulting from the release of meltwater and glacial debris during low sea-level stands at the beginning of interglacial stades. Specific cycles within the unit were tentatively identified with lowstands during MIS 16, 12 and 6. 56.5 Late Pleistocene glaciations (MIS 5–2; 132–11.7 ka) 56.5.1 MIS 5e–3 (132–35 ka) There is little documented evidence in Ecuador for glacial events during this portion of the Pleistocene. Clapperton et al. (1997) briefly mentioned the existence of moraines at 3000 m on the west flank of the Potrerillos Plateau and 2700 m on the east flank, which he suggested pre-dates the global LGM. These were not linked to any of the M1–M6 moraine sequences that Heine and Heine (1996) describe in this region. On the north flank of Carihuairazo, Clapperton (1990) noted the presence of a glacial till constrained by a minimum-limiting 14 C date of > 43 ka. Clapperton suggested that this till dates either from a glacial advance at MIS 4 (~ 70 ka) or perhaps even MIS 6 (before 132 ka). Among his four pre-Holocene moraine classifications for the Cordillera Blanca, Peru ( Fig. 56.6 A–F), Rodbell (1993a) designated Rurec moraines as pre-global LGM; however, he was uncertain about their estimated age. Based on the method of data analysis (extrapolation of weathering rates, linear or logarithmic extrapolation of morainic soil PDI values) and the assumed age of seemingly related moraine groups elsewhere in Peru, four possible age ranges were suggested: (1) 32–20.5 ka, (2) 46.5–27 ka, (3) 132–75 ka or (4) 591–228 ka. Later SED analysis of the Rurec moraines ( Farber et al . , 2005 ) confirmed that they were distinct from and older than the Laguna Baja moraine sequence that Rodbell (1993a) believed represented the global LGM throughout the Cordillera Oriental in northern Peru (including the Cordillera Blanca), but placed their age at 29–20.5 ka, and thus also within MIS 2. Recent recalculation of the CRN data from Farber et al . (2005) by Bromley et al . (2009) further revise the age of the Rurec moraines to ~ 20.5 ka. Extensive evidence of pre-MIS 2 glaciations exists in the mountains surrounding the Junin Plain in central Peru (11.0°S, 76.2°W; Fig. 56.7 A and B). Wright (1983) classified the older of two distinct glacial sequences as the Rio Blanco phase and determined a minimum-limiting age of ~ 42 ka based on a radiocarbon date obtained from Laguna Coochachuyco. Wright further noted that the topographic contrast between the Rio Blanco phase and the younger Punrun phase was very similar to differences between the Bull Lake and Pinedale glaciations in the Rocky Mountains. More recently, Smith et al . (2005) used SED to date the oldest apparent moraines on the east slope of the Junin Plain, slightly south of the area mapped by Wright. This study indicated that these oldest moraines were at least 67 ka, and possibly much older. The pre-MIS 2 moraines of the Junin Plain are consistent with the findings of Mercer and Palacios (1977) , who mapped moraines in the Cordillera Vilcanota (14.0°S, 71.0°W; Fig. 56.8 ), farther south in Peru's Cordillera Oriental. Here, moraines slightly up-valley from the most distal moraines were constrained by a minimum-limiting radiocarbon date of ~ 41.5 ka, while soil development profiling suggested that these moraines could be 115–70 ka, or even older ( Goodman et al . , 2001 ). The most distal moraines, located at 3600 m and more than 16 km from present-day glacier termini, were undated. At the same time, glaciers on the south side of the Cordillera Vilcanota merged with glaciers emanating from the Quelccaya Ice Cap, covering an extensive area above 4500 m. Pre-MIS 2 moraines are also identified on Nevado Coropuna (15.5°S, 72.6°W; Fig. 56.9 ) by Bromley et al . (2009) . Here, adjacent to an MIS 2 moraine sequence designated C-I, the authors noted but could not date a series of moraines that are markedly more weathered than those of the C-I group. In Quebrada Huayllaura, these pre-C-I moraines are located 3 km farther down-valley (at 4150 m) than the C-I group, indicating that this pre-LGM glaciation was more extensive than anything that occurred subsequently. 56.5.2 MIS 3–2 (35–16.7 ka) The identity and location of LGM moraines in Ecuador has been an issue of contention. Heine (1995) and Heine and Heine (1996) placed the M4 moraine sequence identified on both Rucu Pichincha ( Fig. 56.3 ) and the Potrerillos Plateau ( Fig. 56.4 A and B) at the global LGM. The M4 moraines, which occur at 3800–3900 m, are so dated based on their relative position, weathering and oxidation compared to M5 moraines, which are constrained on Rucu Pichincha by a minimum-limiting age of ~ 15.4 ka. Clapperton et al . (1997) , however, identified analogous moraines at 3850 m on the Potrerillos Plateau as belonging to a Late-glacial event he termed the Sucus Glacier Advance and proposed as being associated with an 800 km 2 ice cap atop the plateau. Clapperton et al . (1997) obtained a minimum-limiting radiocarbon date of ~ 15.5 ka for the Sucus Advance. On the Potrerillos Plateau, M4 moraines could only be constrained by a minimum-limiting radiocarbon age of ~ 14.2 ka ( Heine and Heine, 1996 ). It is difficult to reconcile the results obtained by both research teams. The relatively young minimum-limiting age obtained for M4 moraines does not necessarily preclude them from being associated with the 22–19 ka LGM; however, ~ 15.5 ka minimum-limiting age for the Sucus Advance suggests the possibility that the associated moraines may be older and not of Late-glacial origin. Further, Rodbell et al . (2009) noted the potential for mistaken interpretation in Clapperton et al . (1997) based on the possibility that what they interpret as primary glacial tills are in fact soliflucted tills originating from upslope moraines. Rodbell et al . (2009) also noted that the presence of a Late-glacial ice cap at 4300 m at this latitude is inconsistent with evidence from Las Cajas National Park, located at a similar altitude but 2° further south, showing it to have been ice free by 15.6 ka at the latest. Clapperton and McEwan (1985) provided a relative sequence of Quaternary moraines in the Rio Mocha watershed, located between the cones of the Chimborazo–Carihuairazo massif ( Fig. 56.5 A and B), designating groups 1–3. Group 3 moraines are the oldest and most extensive on the massif and occur as low as 3600 m in the Rio Mocha watershed. Though no locally collected datable material constrains the ages of Group 3 moraines, the authors relate these moraines to a till on the northern flanks of Carihuairazo constrained by a maximum-limiting 14 C age of ~ 35,500. Clapperton (1990) extended the mapping of the three moraine groups to the entire Chimborazo–Carihuairazo massif, estimated the spatial extent of the glaciations associated with each moraine group and differentiated two subgroups within Group 3: an inner set of low moraines (5–10 m high) and outer set of high moraines (100–200 m high). Outer moraines descend to 3400 m on the eastern flanks of Carihuairazo and 3800 m on the western flanks of both cones. Inner moraines generally terminate 200–300 m higher in altitude. The structure of Group 3 moraines between Carihuairazo and Chimborazo suggested to Clapperton (1990) that a continuous icefield existed between the two mountains, fed both by glaciers flowing off the two volcanoes as well as its own accumulation zone if/when the local snowline was depressed below 4400 m. During MIS 2, a 400 km 2 ice cap appears to have existed along the crest of Southern Ecuador's Cordillera Occidental in what is now Las Cajas National Park ( Rodbell et al . , 2002 ) (2.8°S, 79.2°W; Fig. 56.10 ). The authors suggested that this MIS 2 ice cap covered ground above 2800 m; however, Clapperton (1986) limited MIS 2 glaciation in the region to 3200 and 3800 m on the range's southeast and northwest flanks, respectively. Clapperton (1986) (reported in Smith et al . , 2008 ) noted that glaciation appears to have occurred as low as 2800 m in the past but links the tills that provide evidence of this to the same weathered tills that exist beyond the limit of Group 3 moraines on the Chimborazo–Carihuairazo massif, and that have been suggested as originating during either MIS 4 or 6 glaciations. Moraine dates are not tightly constrained in the Las Cajas area; however, radiocarbon data obtained from the Chorreras Valley by Rodbell et al . (2002) indicate that the area was ice free by ~ 14 ka. Rodbell (1993b) provided the northernmost palaeoglacial documentation in Peru's segment of the Cordillera Oriental (7.7°S, 77.5°W; Fig. 56.11 ) and dated all existent moraines in the area to the global LGM, suggesting that the MIS 2 glaciation was at least as extensive as earlier glaciations. These were classified as Laguna Baja moraines based on their location relative to the eponymous lake, and this has become the type-name for moraines their age both here and in the Cordillera Blanca. Because recent research indicates that, in the Cordillera Blanca, the Laguna Baja sequence is in fact not representative of the greatest MIS 2 glacial expansion ( Farber et al . , 2005; Bromley et al . , 2009 ) (discussed below), there is now uncertainty about the age classification (though not the relative relationship) of the moraines here. In the Cordillera Blanca ( Fig. 56.6 A–F, the Rurec moraines that Rodbell (1993a) initially believed to pre-date MIS 2 have been determined to be representative of the global LGM ( Farber et al . , 2005 ) and are dated ~ 20.5 ka ( Bromley et al . , 2009 ). Terminal moraines of the Rurec group are located between 3400 and 3800 m, ~ 5 km down-valley from the oldest Laguna Baja moraines that Rodbell (1993a) believed represented the global LGM. Thus Rurec moraines appear to mark the most extensive MIS 2 glacial advance in the range. Laguna Baja moraines are located between 3550 and 4250 m in the Cordillera Blanca. Rodbell (1993a) considered this sequence to be equivalent to the Group 1 (MIS 2) moraines identified by Clapperton (1981) and tentatively dated them to either 30–25 or 14.8–14 ka based on their likely correlation with MIS 2 moraines of uncertain date in the Cordillera Vilcanota reported by Mercer and Palacios (1977) . As with the Rurec moraines, Farber et al . (2005) reported a revised date of 16.5 ka for the Laguna Baja sequence based on SED investigations. Though Bromley et al . (2009) did not specifically address the age of the Laguna Baja sequence, it should be noted that their additional downward revision of the Rurec dates based upon CRONUS calculator recalibration of the Farber et al . (2005) CRN data suggests the possibility that the Laguna Baja sequence may also be younger, and may be of Late-glacial origin. A recent SED study from the Juellesh and Tuco Valleys at the southern end of the Cordillera Blanca ( Fig. 56.6 F) found no evidence of global LGM moraines ( Glasser et al . , 2009 ). Because the oldest moraines identified in the study were approximately concurrent with the Younger Dryas chron, the authors posited that the local climate was too arid between 25 and 18 ka to support extensive glacial advancement. In the Cordillera Huayhuash (10.3°S, 76.8°W; Fig. 56.12 ), Hall et al . (2009) found very little evidence of early MIS 2 glaciations and ascribe this to the morphology of the range, where steep valley slopes inhibit preservation of moraines from one glacial period to the next. Nonetheless, SED of exposed bedrock at 3800 m, the lowest elevation at which glacial features are found in the Jahuacocha Valley, provides a minimum-limiting age of 26 ka, suggesting that deglaciation from MIS 2 maxima may have occurred no later than this. A second set of MIS 2 moraines, which the authors classify as Stage IV, is dated at ~ 22–20 ka, coeval with the global LGM. These are inferred to represent a local ice advance just prior to the onset of an extended period of retreat. The cordilleras on either side of the Junin Plain ( Fig. 56.7 A and B) exhibit ample evidence of MIS 2 glaciation. Wright (1983) reported that most areas above 4500 m in the western Junin Plain and surrounding cordillera were ice-scoured, while glacier termini on the eastern portion of the plain occurred down to 4200 m. Moraines from MIS 2 were classified as Punrun phase moraines, yet limiting age dates for the Punrun were confounded by somewhat conflicting radiocarbon evidence. A sediment core from Lago Junin, on the floor of the plain, revealed a glacial till constrained by maximum–minimum dates of 24.0 and 12.0 ka, respectively. However, a radiocarbon date obtained from a sediment core at the south end of Laguna Huatacocha, ~ 40 km closer to the glacier's source, indicated that ice had retreated past this point by 13.5 ka. Smith et al . (2005) examined moraines on the east side of the Junin Plain, overlapping the earlier mapping work of Wright (1983) in the Calcalcocha Valley. The authors classified four groups of moraines (A–D), with Group C being representative of the local LGM and thus equivalent to the Punrun sequence. Their SED results indicate that the local glacial maxima occurred as early as 34 ka, with recession commencing no later than 21 ka. Though the 34 ka date was later revised downward to 31 ka ( Smith et al . , 2008 ), the key conclusion remained that the local LGM at the Junin Plain significantly pre-dated the global LGM at 22–19 ka. Zech et al . (2007) recalculated the CRN data of Smith et al . (2005) using a different calibration method, and determined that the local LGM was 25–22 ka, in accord with work from the same study in Bolivia's Cordillera Real. Bromley et al . (2009) also recalculated the Smith et al . (2005) dataset using the CRONUS calculator and agreed that the local LGM dates had been overestimated. Smith et al . (2005) identified another MIS 2 morainic sequence, classified as Group B and dated to 20–16 ka. Bromley et al . (2009) recalibrated these dates using the CRONUS calculator and estimated a mean age of 16.1 ± 1.7 ka. On the map accompanying this chapter ( Fig. 56.7 B), the Group D moraines of Smith et al . (2005) , dated at > 67 ka, appear to correlate with the MIS 2 Punrun phase of Wright (1983) . This is not the interpretation of the authors, however; instead this apparent spatial relationship is due only to the different scales and possible slight spatial inaccuracies of the original maps. On the northwest flank of the Cordillera Vilcanota (14.0°S, 71.0°W; Fig. 56.8 ), a cluster of well-nested moraines between 4000 and 4350 m in the Upismayo and Jalacocha Valleys was determined to be of MIS 2 origin based on maximum-limiting radiocarbon date of ~ 34.4–31.7 ka and a minimum-limiting radiocarbon date of 16.8 ka ( Mercer and Palacios, 1977 ). These moraines have subsequently been classified as the U3 sequence ( Mark et al . , 2004 ). Goodman et al . (2001) combining soil development profiling and radiocarbon dating of lake/bog sediments, calculated a 16.65 ka minimum-limiting age for the U3 sequence and argued that these moraines were the result of a distinct glacial advance that culminated at this time, noting that such a chronology was contemporaneous with other sites in the tropical Andes as well as the Heinrich Event One evidenced by ice-rafted debris in the North Atlantic. Mark et al . (2002) examined the U3 moraines and noted that, while well-nested, the moraines were also cross-cutting and thus suggestive of multiple glacial advances that happened to terminate at the same location. Further, the authors obtained a radiocarbon minimum-limiting date from nearby Laguna Casercocha in a sediment core of ~ 20 ka, which they argued bolstered their hypothesis that the U3 sequence represents two different MIS 2 advances. Mercer and Palacios (1977) mapped moraines in the vicinity of the Quelccaya Ice Cap (14.0°S, 70.9°W; Fig. 56.13 ) and defined three glacial phases: Huancané I, Huancané II and Huancané III. Huancané III moraines are the oldest of these but are considered to be of Late-glacial origin based on a minimum-limiting age of ~ 14 ka. Goodman et al . (2001) identified a series of moraines ~ 14 km southwest of the present ice face and obtained a minimum-limiting radiocarbon date of 25.5 ka. Their age and distance from the ice face indicate that these moraines originated from a different advance than those of the Huancané III group. A distinct group of moraines on Nevado Coropuna, a glacier-capped stratovolcano at the southern end of Peru's Cordillera Occidental ( Fig. 56.9 ) were estimated at 21.4–20.7 ka based on SED by Bromley et al . (2009) , using the CRONUS calculator scaling method. These moraines, classified C-I, occur at 4600 m and 4350 m on the west and north sides of the mountain, respectively, and are interpreted as representative of an LGM advance on the mountain. Based on recalibration of surface exposure data from Smith et al . (2005) and Farber et al . (2005) , the authors argued that the C-I sequence is concurrent with the Group C (Junin Plain) and Rurec (Cordillera Blanca) moraine sequences. Smith et al . (2005) found synchrony between the glacial phases of in Bolivia's Cordillera Real (16.3°S, 68.2°W; Fig. 56.14 A and B) and those of the Junin Plain region of central Peru, and use the same moraine classification (Group C for the MIS 2 local LGM, Group B for Late-glacial). As with the Junin Plain moraines, Zech et al . (2007) recalculated the Group C dates of Smith et al . (2005) and estimated an age range of ~ 25–22 ka here as well. In the Cordillera Real, Smith et al . (2005) studied a pair of valleys on either side of Nevado Huayna Potosi ( Fig. 56.14 B) and found distinctly different glacial behaviour during each sequence. In the west-facing Milluni Valley, the lowest Group B moraines are located about 8–10 km up-valley from the local LGM Group C moraines, while in the steep, narrower, east-facing Zongo Valley, no Group C moraines are identified while Group B moraines appear to have progressed at least 1000 m lower in altitude and are the oldest in the valley. The authors attributed the difference in Group B extent to increased snowfall, debris-cover on the glacier surface, and topographic shading in the Zongo Valley having allowed the maintenance of ice at lower elevations. Approximately 60 km northwest of the Milluni Valley, Zech et al . (2007) used SED to estimate the age of moraines at the mouth of the Valle San Francisco ( Fig. 56.14 A) as ~ 25–22 ka, or coeval with the Group C moraines of Smith et al . (2005) . Zech et al . (2007) also identified a local glacial advance at ~ 15 ka, which could be equivalent to the Group B sequence. Farther south in the Cordillera Cochabamba (17.2°S, 66.5°W; Fig. 56.15 ), Zech et al . (2007) estimated an identical ~ 25–22 ka range for the local LGM. 56.5.3 Late-glacial (16.7–11.7 ka) In addition to their disagreement over the global LGM or Late-glacial provenance of Potrerillos Plateau moraines located at 3800–3900 m, Heine and Heine (1996) and Clapperton et al . (1997) provided differing opinions as to Late-glacial conditions, particularly whether or not there is evidence of cooling that correlates with the Younger Dryas chron in the Ecuadorian glacial record. Heine and Heine described M5 and M6 moraines on both Rucu Pichincha ( Fig. 56.3 ) and the Potrerillos Plateau ( Fig. 56.4 A and B). M5 moraines are constrained by a minimum-limiting age of ~ 15.4 ka (Rucu Pichincha) and ~ 14.8 ka (Potrerillos Plateau), and in both locales occur between 4100 and 4200 m. M6 moraines occur inside M5 moraines at roughly the same elevation in both locales and are age-bracketed by dates of ~ 13 ka (obtained from an underlying peat) and 8.2 ka (obtained from an overlying tephra). Based on the above chronology, Heine and Heine (1996) suggested the following Late-glacial scenario for Rucu Pichincha and the Potrerillos Plateau: after the local LGM, glaciers re-advance by 15.4 ka (Rucu Pichincha) or 14.8 ka (Potrerillos Plateau) to deposit M5 moraines. As M5 glaciers recede, lakes (Rucu Pichincha) and peat bogs (Potrerillos Plateau) form behind M5 moraines, but these desiccate as the climate dries at ~ 13 ka (Rucu Pichincha) and ~ 13.3 (Potrerillos Plateau). Glaciers advance once more to deposit M6 moraines sometime between 12.5 and 9 ka. Clapperton et al . (1997) identified moraines correlating to the M6 sequence as evidence of the ‘Potrerillos Glacier Advance’, which they associate with the redevelopment of a smaller (140 km 2 ) ice cap atop the Potrerillos Plateau. Because this advance is constrained by a maximum-limiting age of 12.9 ka and is evidenced by the burial of paramó vegetation between 12.71 and 12.26 ka BP in one sediment core, the authors argued it was coeval with the onset of the Younger Dryas chron in the Northern Hemisphere. Heine and Heine (1996) argued that the onset of M6 glaciation occurred 400–500 years after the beginning of Younger Dryas cooling in Europe and that, without explanation of this lag, a correlation could not be made. However, the calibrated radiocarbon dates derived from the original Heine and Heine (1996) 14 C dates (~ 12.85 ka, per Rodbell et al . , 2009 ) are in very close proximity to the 12.9 ka date generally accepted as the onset of the Younger Dryas chron ( Rasmussen et al . , 2006 ). As noted earlier, however, the presence of any ice cap at the altitude/latitude combination present at the Potrerillos Plateau is inconsistent with observed glacial behaviour elsewhere in the Ecuadorian Andes ( Rodbell et al . , 2009 ). Both research groups agree that both Rucu Pichincha and the Potrerillos Plateau were deglaciated no later than the Early Holocene (~ 11 ka per Clapperton et al . , 1997 and no later than 8.2 ka per Heine and Heine, 1996 ), and have remained ice free ever since. Late-glacial moraines on the Chimborazo–Carihuairazo massif were classified by Clapperton and McEwan (1985) and Clapperton (1990) as belonging to Group 2 ( Fig. 56.5 A and B). In the Rio Mocha drainage, these moraines occur as low as 3900 m and indicate that glaciers from both Chimborazo and Carihuairazo were confluent during this period as well. Clapperton and McEwan (1985) identified the site of a former glacial lake created by the damming of the Rio Mocha by a Group 2-era lobe of the modern-day Reschreiter Glacier on Chimborazo. Radiocarbon dates obtained from a pair of peat layers exposed at this site were calculated as ~ 13.2 and ~ 12.7 ka, respectively. The authors interpreted this as being the result of multiple lacustrine-peat bog transitions associated with glacial terminus variations and correlate the advance responsible for the lake with a contemporaneous glacial stage identified by Mercer and Palacios (1977) at the Quelccaya Ice Cap—interpreted as coincident with the Younger Dryas chron. Heine and Heine (1996) countered the assumption that the dates obtained from the two peat layers were indicative of the dates of the glacial advance, noting that more than 8 m of lacustrine sediments underlie the two peat layers and that the lake must have been present, based on local sedimentation rates, at least 2.5 ka years prior to the dates calculated by Clapperton and McEwan (1985) . Further, Heine and Heine (1996) posited that a moraine was the lake-forming feature rather than the glacier itself and that the lake would have been present even after the glacier had retreated. Instead, the authors hypothesised that the moraine responsible for the formation of this lake was associated with the M5 glacier advance that they identified at Rucu Pichincha and the Potrerillos Plateau approximately 14.8–15.6 ka. Rodbell and Seltzer (2000) reported visiting the site of this glacial lake in 1993 and stated that they agree with the interpretation of Heine and Heine (1996) that a direct relationship between lake fluctuations and variation in ice margins is questionable. Radiocarbon evidence from the northern Cordillera Oriental in Peru ( Fig. 56.11 ) indicates that glaciers had retreated past Laguna Baja (3600 m) by ~ 14 ka, representing a greater than 50% reduction in areal extent relative to their MIS 2 maxima ( Rodbell, 1993b ). There is evidence of glacial re-expansion above 3800 m between ~ 14.2 and 12.0 ka; however, final deglaciation was rapid, with the area being ice free between 12.0 and 11.1 ka. There is strong geomorphologic evidence for a Late-glacial ice advance in the Cordillera Blanca ( Fig. 56.6 A–F). Rodbell (1993a) defined the Manachaque moraine sequence (equivalent to the Group 2 moraines of Clapperton, 1981 ) and dated these features to ~ 13.5–10.3 ka. Manachaque moraines are located between 3800 and 4400 m and are distributed over a distance of 10 km down-valley from the oldest Holocene moraines. Rodbell and Seltzer (2000) examined a lacustrine sediment sequence from a former glacial lake (Glacial Lake Breque, Fig. 56.6 D) and were able to closely bracket a glacial advance that culminated at 12.9 ka and was immediately followed by rapid ice retreat. Because this retreat occurred despite strong evidence of regional cooling at this time, the authors inferred a sharp decrease in precipitation that starved glaciers of mass even though temperatures were reduced. This evidence of glacial retreat commencing at 12.9 ka, which the authors also found present at the Quelccaya Ice Cap, would appear to contradict other research ( Clapperton and McEwan, 1985; Rodbell, 1993a; Clapperton et al . , 1997 ) finding glacial advances concurrent with the Younger Dryas chron in the northern hemisphere. The authors, however, did not eliminate the possibility that the drying conditions were associated with a global scale event during the Younger Dryas chron. Instead, they noted that the cool and moist conditions that led to a glacial advance prior to 12.9 ka indicate markedly different conditions in the tropical Andes than those that define the proceeding Bølling-Allerød interstadial in the northern hemisphere. Glasser et al . (2009) confirmed the presence of moraines dating from immediately prior to or early in the Younger Dryas chron in the southern Cordillera Blanca ( Fig. 56.6 F). In the Juellesh Valley, they dated a moraine at 12.4 ± 0.6 ka, while in the Tuco Valley, the oldest, most exterior lateral moraine returned a date of 12.5 ± 1.1 ka. The authors remarked upon the potential coincidence of these moraines with the Younger Dryas chron but did not explicitly link them, noting that the separation of temperature from precipitation as a forcing mechanism for tropical glacier change is difficult. A pair of nested moraines located ~ 1 km farther up the Juellesh Valley were dated at ~ 10.8 and ~ 9.7 ka, respectively, suggesting either a somewhat subdued rate of retreat from the ~ 12.4 ka position followed by an extended stillstand or possibly even a minor re-advance. In the Cordillera Huayhuash ( Fig. 56.12 ), at least two, and possibly three, sets of Late-glacial moraines have been identified at elevations as low as 4000 m ( Hall et al . , 2009 ). Using a combination of SED and radiocarbon dating, the authors placed Stage III moraines at 14–13 ka, and related them to Manachaque moraines in the Cordillera Blanca ( Rodbell, 1993a ) and Group A moraines of the Junin Plain region ( Smith et al . , 2005 ). While Stage II moraines, dated at 10–9 ka, were the next prominent set of moraines, the authors also identified a Stage IIa represented by smaller moraines immediately down-valley from the larger Stage II features. Stage IIa moraines were dated at ~ 12–11.5 ka, which the authors suggested potentially represent an advance synchronous with the Younger Dryas chron. They noted, however, that the ages of the more dominant Stage II and Stage III moraines are not synchronous with the Younger Dryas. The existing literature from the Junin Plain ( Fig. 56.7 A and B) describes very little in the way of Late-glacial ice extent and behaviour. Smith et al . (2005) defined a Group A moraine classification and dated these moraines to ~ 15–12 ka; however, they mapped only one such moraine, in the Alcacocha Valley. In the Upismayo Valley of the Cordillera Vilcanota ( Fig. 56.8 ), Mark et al . (2002) described a group of moraines 4 km up-valley from the late-MIS 2 U3 group, which Mark et al . (2004) classified as the U2 sequence. Mark et al . (2002) were unable to define a precise date range for the U2 moraines, determining only maximum/minimum-limiting ages of 12.25 and 5.05 ka, respectively. The authors placed greater weight on the lower end of this range and suggested that U2 moraines were most likely associated with a Middle Holocene advance. Mercer and Palacios (1977) described three distinct terminal moraine sequences in the Rio Huancané Valley, west of the Quelccaya Ice Cap ( Fig. 56.13 ), and placed two of these, Huancané III and Huancané II, within the Late-glacial period. Huancané III is the oldest and is constrained by a minimum-limiting radiocarbon date obtained from a peat sample of 14.2 ka. While this date is only a minimum limit, the authors believed that the ice cap retreated from the Huancané III moraines very near to these limiting dates. Based on additional radiocarbon dates, the authors argued that the Huancané II sequence resulted from a glacial advance that was in progress by 13.3 ka and over by ~ 12.9 ka. Rodbell and Seltzer (2000) agreed with this Quelccaya chronology, and their research in the Cordillera Blanca identified a glacial advance constrained by very similar dates, leading them to conclude that glaciers in both central and southern Peru varied in near ‘lockstep’ and that this was indicative of at least regional climatic forcing at that time. Goodman et al . (2001) studied the Huancané II moraines and calculated minimum-limiting radiocarbon dates of 13.1 and 12.8 ka based on their own coring analysis. They also obtained a minimum-limiting date of 10.1 ka for moraines fronting Laguna Churuyo, ~ 8 km southwest of the present ice face. The location of these moraines, however, is suggestive of the Huancané III sequence. Mark et al . (2002) also examined the Huancané sequences, obtained from a peat sample a minimum-limiting radiocarbon date of 14.3 ka for the Huancané III sequence. The authors also mentioned the 12.8 ka radiocarbon date obtained from Laguna Paco Cocha by Rodbell and Seltzer (2000) and noted that this indicates that the Quelccaya Ice Cap has not been farther than 1 km from its current position anytime in the past 12.8 ka years. On Nevado Coropuna ( Fig. 56.9 ), a group of moraines classified as the C-II sequence are constrained by surface exposure dates of 14.2 and 10.6 ka, with a mean age of 13.0 ± 1.6 ka ( Bromley et al . , 2009 ). In Quebradas Ullullo and Santiago, C-II moraines terminate 3.5 km up-valley from the C-I sequence, and on the north side of the mountain, they terminate at 4700 m, 350 m higher than the C-I group. The authors tentatively suggested that the C-II group was equivalent to the Laguna Baja sequence in the Cordillera Blanca, based on the fact that the Laguna Baja age had been revised downward to 16.5 ka by Farber et al . (2005) and that the revised Rurec sequence dates of Farber et al . (2005) had themselves been overestimated due to the SED scaling method they had applied. A key point made by Bromley et al . (2009) was that while the exact dates of the C-I and C-II sequences may be uncertain due to the variability associated with the use of competing calibration procedures, the 6- to 7-k year difference between the two phases is consistent with similar global LGM to Late-glacial recessional timelines identified at sites in New Zealand, North America and Europe. This, they argued, suggests that in this portion of the Andes, these glacial cycles were in synchrony with global events and that the variation in specific reported dates may be more a function of imprecise dating methods. As with the Junin Plain, Smith et al . (2005) identified Group A moraines in the Zongo Valley of the Cordillera Real, Bolivia ( Fig. 56.14 B), and suggested a date range of ~ 15–12 ka based on SED. In the Cordillera Cochabamba ( Fig. 56.15 ), Zech et al . (2007) dated prominent lateral moraines in both the Valle Huara Loma and the Valle de Rio Suturi to 13.3–11.0 ka, again broadly consistent with advances identified elsewhere in the tropical Andes at roughly the same time as the Younger Dryas chron. The authors also dated the most exterior, lower-elevation moraine in the Valle de Rio Suturi to 16.9 ka, though they admitted difficulty in reconciling this date with the ages of older moraines identified in the Valle Haura Loma and noted that the particular boulder from which the sample was obtained had clear signs of erosion, suggesting an example of cosmogenic underestimation. Nevado Sajama (18.1°S, 68.9°W; Fig. 56.16 ), a 6500-m stratovolcano in Bolivia's Cordillera Occidental, is among the southernmost glacierised peaks in the tropical Andes. Smith et al . (2009) applied SED methods to classify four groups of moraines on the mountain, one of which dates from the Late-glacial. Ground Moraine 1, with minimum elevations between 4350 and 4635 m, is composed of previously englacial or supraglacial materials and is broadly dated to between 16.9 and 11.3 ka. However, lateral and terminal moraines associated with Ground Moraines 1 (and Holocene Ground Moraine 2) are composed of two different morainic structures, tall bounding moraines and short cross-cutting moraines, which confounds age estimation attempts. The tall moraines lack the boulders necessary for SED analysis; however, the authors hypothesised that these are relic landforms rather than the products of a Late-glacial or MIS 2 event while the short cross-cutting moraines were proposed to be the result of past cold-based ice, a glacier type not previously identified in the tropical Andes. Because of the intermixture of these moraine types and the lack of differentiation on the original Smith et al . (2009) map, they are not dated in Fig. 56.16 . In any case, the authors argue that the most extensive local glacial advance appears to have occurred during the Late-glacial period rather than synchronous with the global LGM, since the outermost moraines appear no older than 16.9 ka, though they do not rule out the possibility of an earlier MIS 2 advance that has yet to be identified in Bolivia's Cordillera Occidental. 56.6 MIS 1 (Holocene) glaciations (11.7 ka–present) Groups of superimposed moraines above 4300 m on the Chimborazo–Carihuairazo massif are classified as Holocene Group 1 moraines by Clapperton and McEwan (1985) and Clapperton (1990) ( Fig. 56.5 A and B). These are interpreted as the result of multiple, similarly sized Neoglacial (> 6 ka) re-advances. In the Cordillera Blanca ( Fig. 56.6 A–F), Clapperton (1981) classified Group 3 and Group 4 moraines as representative of Holocene ice-face fluctuations, while Rodbell (1993a) identified four separate Holocene moraine groups in the range. In the Juellesh Valley, Glasser et al . (2009) dated a largely unaltered moraine 1.5 km below the present-day glacier terminus at 7.6 ka and suggested that this date is broadly coincident with the 8200-year cooling event identified in Greenland and elsewhere. No such advance is identified in the Cordillera Huayhuash ( Fig. 56.12 ); however, the Stage I moraines described by Hall et al . (2009) indicate a minor Middle Holocene advance between ~ 5.6 and 3.5 ka. In the Cordillera Callejon, west of the Junin Plain ( Fig. 56.7 A), Wright (1983) noted that, while the current ice coverage is approximately 6 km 2 , it appears to have been as much as 22 km 2 , ‘in the recent past’. No specific dates are provided, however. In the Cordillera Vilcabamba (13.4°S, 72.6°W; Fig. 56.17 ), northwest of Cuzco, Peru, Licciardi et al . (2009) obtained surface exposure dates for two Holocene moraine sequences. The oldest and most extensive of the two sequences yielded an estimated date of 8.6 ± 0.3 ka which is similar in time frame to the advance described above in the Cordillera Blanca. The authors also obtained a date of 200 ± 20 years (i.e. CE 1810 ± 20) on an inner moraine in the Rio Blanco Valley. From a procedural standpoint, this is interesting because it shows that 10 Be SED can be used on morphological features of even historic age. From a climatological standpoint, these results indicate that a glacial advance occurred here that was broadly contemporaneous with the northern hemisphere Little Ice Age (LIA; CE 1300–1860) and that the present-day glacier has receded nearly 2 km since this time. Compared to lichenometry studies of recent Holocene moraines elsewhere in the tropical Andes (e.g. Rodbell, 1992; Jomelli et al . , 2009 ), which identify LIA maxima at CE 1630 ± 30 in the Cordillera Blanca and ~ CE 1669–1690 in the Cordillera Oriental of Bolivia, the LIA here appears to lag by over a century. While this may be due to imprecision associated with both lichenometry and SED, the authors believed that there were in fact differences in the timing of LIA glacial maxima within these regions. The authors also noted that the LIA maximum in the Cordillera Vilcabamba postdates a transition from wetter to drier conditions recorded in Quelccaya ice cores but is coincident with an abrupt shift towards nutrient-rich, oxygen-depleted ocean water recorded in marine sediment cores obtained at the same latitude off the Peruvian coast ( Licciardi et al . , 2009 ). In the Cordillera Vilcanota ( Fig. 56.8 ), Holocene moraines have been classified as the U1 sequence ( Mark et al . , 2004 ). Goodman et al . (2001) obtained a radiocarbon date of 390 years from the highest peat bog they cored in the Upismayo Valley, indicating that all moraines located up-valley from this point are LIA in origin. A minimum-limiting radiocarbon date of 2.9 ka was obtained for a moraine ~ 2 km down-valley from the present ice face in the same study. Goodman et al . (2001) concluded that the LIA was the most extensive Late Holocene glacial advance both here and at the Quelccaya Ice Cap. The Huancané I sequence described by Mercer and Palacios (1977) at Quelccaya ( Fig. 56.13 ) is of Holocene provenance, and moraines of this sequence occur less than 1 km from the 1977 face of the ice cap. The authors noted that the Huancané I moraines contain a great deal of bulldozed peat, and one apparently stratigraphically intact peat deposit returned a radiocarbon date of 750–850 years. Mark et al . (2002) calculated a maximum-limiting age for the Huancané I moraines of 300 (+ 202/−300) years, indicating that this moraine sequence marks the LIA extent of the ice cap. The authors also noted that peat exposed at the present ice margin has been radiocarbon dated to ~ 2.8 ka, implying that the ice cap has in fact been smaller during the Holocene than it is at present. In the Cordillera Cochabamba ( Fig. 56.15 ), Zech et al . (2007) dated one recessional moraine to 7.0 ka but noted that they have been unable to corroborate this date with other evidence. Three of the four ground moraine classifications identified by Smith et al . (2009) on Nevado Sajama date from the Holocene ( Fig. 56.16 ). Ground Moraine 2 occurs in 12 of the 15 valleys radiating from the mountain, with minimum elevations ranging from 4585 to 4925 m. Like the Late-glacial Ground Moraine 1, these are composed of previously englacial and/or supraglacial material. Using SED, the authors estimate an age of 7.1–4.0 ka for this sequence. Ground Moraine 3 has been identified in eight of Sajama's valleys, at elevations ranging from 4745 to 5000 m. The structure of this sequence appears similar to that of a rock glacier in that they lack lateral moraines; however, they are not related to rock glaciers currently present on the mountain. These are estimated to date from 4.7 to 3.3 ka. A final group, the Plateau Ground Moraines, are found only on high-elevation, low angle plateaus separated from the main massif by secondary peaks and extensive bedrock exposures. Because of this spatial characteristic and their lack of associate lateral and terminal moraines, these are interpreted as being the result of small, cold-based ice caps. Plateau moraines are estimated to date from 7.0 to 6.5 ka. Smith et al . (2009) noted that the Holocene glacial sequences identified on Sajama are potentially synchronous with Holocene advances identified in the Cordillera Blanca by Rodbell (1992) . Further, they suggested that the Holocene advances appear to coincide with lower lake levels at Lake Titicaca, on the Peruvian–Bolivian Altiplano. While such lowstands have previously been interpreted as representing a warm/dry climate period, the authors hypothesise that while it may have been drier at this time, perhaps it was not as warm, thus allowing glacial advance contemporaneous with reduced regional lake levels. These Holocene advances, however, are not coeval with any Holocene glacial advances reported from the Cordillera Vilcabamba ( Licciardi et al . , 2009 ). There, Licciardi et al . (2009) found that Early Holocene glacial behaviour did closely correlate with variation in Titicaca's lake levels, suggesting that both are forced by some combination of increased precipitation and decreased temperature. 56.7 Conclusions The Quaternary glacial chronology of the tropical Andes has come into better focus over the past 5 years as numerous additional studies highlighting both new sites and utilising new dating techniques have been published. Examining the literature, it is now possible to identify broadly synchronous glacial advances within the region: 1. An MIS 2 advance that culminates between 25 and 20 ka (with emphasis on the lower end of this range, coeval with the global LGM) is marked by moraine sequences of the Rurec in the Cordillera Blanca (20.5 ka) ( Rodbell, 1993a; Farber et al . , 2005; Bromley et al . , 2009 ); Stage IV in the Cordillera Huayhuash (22–20 ka) ( Hall et al . , 2009 ); Group C (and Punrun) in the Junin Plain and Cordillera Real (25–22 ka) ( Wright, 1983; Smith et al . , 2005; Bromley et al . , 2009 ); and C-I on Nevado Coropuna ( Bromley et al . , 2009 ). 2. A second MIS 2 glacial advance that culminates ~ 16 ka is evidenced by moraines of the M5 on Rucu Pichincha and the Potrerillos Plateau (~ 15.4 ka) ( Heine and Heine, 1996 ); Laguna Baja in the Cordillera Blanca (16.5 ka) ( Rodbell, 1993a; Farber et al . , 2005 ); Group B in the Junin Plain and Cordillera Real (16.1 ka) ( Smith et al . , 2005; Zech et al . , 2007; Bromley et al . , 2009 ); and probably some portion of the U3 moraines in the Cordillera Vilcanota (16.9 ka) ( Mercer and Palacios, 1977; Goodman et al . , 2001; Mark et al . , 2002 ). 3. Finally, there is strong evidence for a glacial advance either just prior to or coeval with the Younger Dryas chron, including the Potrerillos Advance on the Potrerillos Plateau (12.9 ka) ( Clapperton et al . , 1997 ); the Breque Moraine in the central Cordillera Blanca (12.9 ka) ( Rodbell and Seltzer, 2000 ); Juellesh and Tuco Valley moraines in the southern Cordillera Blanca (12.5 ka) ( Glasser et al . , 2009 ); Stage III in the Cordillera Huayhuash (14–13 ka) ( Hall et al . , 2009 ); Huancané II moraines below the Quelccaya Ice Cap (13.3–12.9 ka) ( Mercer and Palacios, 1977; Rodbell and Seltzer, 2000; Goodman et al . , 2001; Mark et al . , 2002 ); and C-II moraines on Nevado Coropuna (13.0 ka) ( Bromley et al . , 2009 ). Not every dated moraine sequence fits neatly within this timeline, however, and numerous problems must be resolved before any assumptions can be made about palaeoglaciation in the tropical Andes. First, identification and acceptance of a preferred SED scaling method that best accounts for the variations inherent to CRN decay at low latitudes and high altitudes is necessary for SED studies to be compared with one another and studies using other dating methodologies. Second, an improved understanding of the relationship between precipitation and temperature forcing mechanisms of glacial behaviour is needed, both between the inner tropical Andes (Ecuador) and the outer tropical Andes (Peru and Bolivia), as well as between the Cordilleras Occidental and Oriental in all three countries. Third, because of its potential importance in explaining climatic linkages between the northern hemisphere and the tropics, a better understanding of glacial behaviour during the Younger Dryas chron (12.9–11.7 ka) is necessary. The presence of evidence for glacial advance during the Younger Dryas chron in the tropical Andes has been an issue of particular contention, and while the interpretation of Rodbell and Seltzer (2000) is supported by evidence from several Peruvian sites, much uncertainty remains. Fourth, additional investigations of Holocene glacial behaviour in the region are needed, especially with regard to a potential signal from the northern hemisphere 8200-year event identified at select sites in Peru. Finally, despite the number of new studies, additional research is needed at sites where the palaeoglacial chronology remains unexamined or poorly understood, especially in the Ecuadorian Andes. References Balco et al., 2008 G. Balco J.O. Stone N.A. Lifton T.J. Dunai A complete and easily accessible means of calculating surface exposure ages or erosion rates from Be-10 and Al-26 measurements Quatern. Geochronol. 3 2008 174 195 Bromley et al., 2009 G.R.M. Bromley J.M. Schaefer G. Winckler B.L. Hall C.E. Todd K.M. Rademaker Relative timing of last glacial maximum and late-glacial events in the central tropical Andes Quatern. Sci. Rev. 28 2009 2514 2526 Clapperton, 1981 C.M. Clapperton Quaternary glaciations in the Cordillera Blanca, Peru and the Cordillera Real, Bolivia 1981 Revista Centro Interamericano de Fotointerpretacion C.I.A.F., Bogata, Columbia, pp. 93–111 Clapperton, 1983 C.M. Clapperton The glaciation of the Andes Quatern. Sci. Rev. 2 1983 83 84 IN1–IN2, 85–155 Clapperton, 1986 C.M. Clapperton Glacial geomorphology, Quaternary glacial sequence and paleoclimatic inferences in the Ecuadorian Andes V. Gardiner International Geomorphology 1986, Part II 1986 Wiley London 843 870 Clapperton, 1990 C.M. Clapperton Glacial and Volcanic Geomorphology of the Chimborazo–Carihuairazo Massif, Ecuadorian Andes Trans. R. Soc. Edinburgh Earth Sci. 81 1990 91 116 Clapperton and McEwan, 1985 C.M. Clapperton C. McEwan Late Quaternary moraines in the Chimborazo Area, Ecuador Arctic Alpine Res. 17 1985 135 142 Clapperton et al., 1997 C.M. Clapperton M. Hall P. Mothes M.J. Hole J.W. Still K.F. Helmens A Younger Dryas icecap in the equatorial Andes Quatern. Res. 47 1997 13 28 Farber et al., 2005 D.L. Farber G.S. Hancock R.C. Finkel D.T. Rodbell The age and extent of tropical alpine glaciation in the Cordillera Blanca, Peru J. Quatern. Sci. 20 2005 759 776 Glasser et al., 2009 N.F. Glasser S. Clemmens C. Schnabel C.R. Fenton L. McHargue Tropical glacier fluctuations in the Cordillera Blanca, Peru between 12.5 and 7.6 ka from cosmogenic 10Be dating Quatern. Sci. Rev. 28 2009 3448 3458 Goodman et al., 2001 A.Y. Goodman D.T. Rodbell G.O. Seltzer B.G. Mark Subdivision of glacial deposits in southeastern Peru based on pedogenic development and radiometric ages Quatern. Res. 56 2001 31 50 Hall et al., 2009 S.R. Hall D.L. Farber J.M. Ramage D.T. Rodbell R.C. Finkel J.A. Smith Geochronology of Quaternary glaciations from the tropical Cordillera Huayhuash, Peru Quatern. Sci. Rev. 28 2009 2991 3009 Hastenrath, 2009 S. Hastenrath Past glaciation in the tropics Quatern. Sci. Rev. 28 2009 790 798 Heine, 1995 K. Heine Late Quaternary glacier advances in the Ecuadorian Andes: a preliminary report J. Rabassa M. Salemme Quaternary of South America and Antarctic Peninsula 1995 A.A. Balkema Rotterdam 1 22 Heine, 2000 K. Heine Tropical South America during the Last Glacial Maximum: evidence from glacial, periglacial and fluvial records Quatern. Int. 72 2000 7 21 Heine and Heine, 1996 K. Heine J.T. Heine Late glacial climatic fluctuations in Ecuador: glacier retreat during younger dryas time Arctic Alpine Res. 28 1996 496 501 Jomelli et al., 2009 V. Jomelli V. Favier A. Rabatel D. Brunstein G. Hoffmann B. Francou Fluctuations of glaciers in the tropical Andes over the last millennium and palaeoclimatic implications: a review Palaeogeogr. Palaeoclimatol. Palaeoecol. 281 2009 269 282 Kaser and Osmaston, 2002 G. Kaser H. Osmaston Tropical Glaciers 2002 Cambridge University Press Cambridge, UK Klein et al., 1999 A.G. Klein G.O. Seltzer B.L. Isacks Modern and last local glacial maximum snowlines in the Central Andes of Peru, Bolivia, and Northern Chile Quatern. Sci. Rev. 18 1999 63 84 le Roux et al., 2000 J.P. le Roux C. Tavares Correa F. Alayza Sedimentology of the Rímac-Chillón alluvial fan at Lima, Peru, as related to Plio-Pleistocene sea-level changes, glacial cycles and tectonics J. South Am. Earth Sci. 13 2000 499 510 Licciardi et al., 2009 J.M. Licciardi J.M. Schaefer J.R. Taggart D.C. Lund Holocene glacier fluctuations in the Peruvian Andes indicate northern climate linkages Science 325 2009 1677 1679 Mark, 2008 B.G. Mark Tracing tropical Andean glaciers over space and time: some lessons and transdisciplinary implications Global Planet. Change 60 2008 101 114 Mark et al., 2002 B.G. Mark G.O. Seltzer D.T. Rodbell A.Y. Goodman Rates of deglaciation during the Last Glaciation and Holocene in the Cordillera Vilcanota-Quelccaya Ice Cap Region, Southeastern Perú Quatern. Res. 57 2002 287 298 Mark et al., 2004 B.G. Mark G.O. Seltzer D.T. Rodbell Late Quaternary glaciations of Ecuador, Peru and Bolivia J. Ehlers P.L. Gibbard Quaternary Glaciations—Extent and Chronology Part III 2004 Elsevier Amsterdam 151 163 Mark et al., 2005 B.G. Mark S.P. Harrison A. Spessa M. New D.J.A. Evans K.F. Helmens Tropical snowline changes at the last glacial maximum: a global assessment Quatern. Int. 138–139 2005 168 201 Martinson et al., 1987 D.G. Martinson N.G. Pisias J.D. Hays J. Imbrie T.C. Moore N.J. Shackleton Age dating and the orbital theory of the ice ages: development of a high-resolution 0 to 300,000-year chronostratigraphy Quatern. Res. 27 1987 1 29 Mercer and Palacios, 1977 J.H. Mercer M.O. Palacios Radiocarbon dating of the last glaciation in Peru Geology 5 1977 600 604 Rasmussen et al., 2006 S.O. Rasmussen K.K. Andersen A.M. Svensson J.P. Steffensen B.M. Vinther H.B. Clausen A new Greenland ice core chronology for the last glacial termination J. Geophys. Res. A Space Phys. 111 2006 D06102 Rodbell, 1992 D.T. Rodbell Lichenometric and radiocarbon dating of Holocene glaciation, Cordillera Blanca, Peru Holocene 2 1992 19 29 Rodbell, 1993a D.T. Rodbell Subdivision of Late Pleistocene moraines in the Cordillera Blanca, Peru, based on rock-weathering features, soils, and radiocarbon dates Quatern. Res. 39 1993 133 143 Rodbell, 1993b D.T. Rodbell The timing of the last deglaciation in Cordillera Oriental, Northern Peru, based on glacial geology and lake sedimentology Geol. Soc. Am. Bull. 105 1993 923 934 Rodbell and Seltzer, 2000 D.T. Rodbell G.O. Seltzer Rapid ice margin fluctuations during the Younger Dryas in the Tropical Andes Quatern. Res. 54 2000 328 338 Rodbell et al., 2002 D.T. Rodbell S. Bagnato J.C. Nebolini G.O. Seltzer M.B. Abbott A Late Glacial-Holocene Tephrochronology for Glacial Lakes in Southern Ecuador Quatern. Res. 57 2002 343 354 Rodbell et al., 2009 D.T. Rodbell J.A. Smith B.G. Mark Glaciation in the Andes during the Lateglacial and Holocene Quatern. Sci. Rev. 28 2009 2165 2212 Seltzer, 1990 G.O. Seltzer Recent glacial history and paleoclimate of the Peruvian–Bolivian Andes Quatern. Sci. Rev. 9 1990 137 152 Smith et al., 2005 J.A. Smith G.O. Seltzer D.L. Farber D.T. Rodbell R.C. Finkel Early local last glacial maximum in the tropical Andes Science 308 2005 678 681 Smith et al., 2008 J.A. Smith B.G. Mark D.T. Rodbell The timing and magnitude of mountain glaciation in the tropical Andes J. Quatern. Sci. 23 2008 609 634 Smith et al., 2009 C.A. Smith T.V. Lowell M.W. Caffee Lateglacial and Holocene cosmogenic surface exposure age glacial chronology and geomorphological evidence for the presence of cold-based glaciers at Nevado Sajama, Bolivia J. Quatern. Sci. 24 2009 360 372 Stuiver and Reimer, 1993 M. Stuiver P.J. Reimer Extended 14C database and revised CALIB radiocarbon calibration program Radiocarbon 35 1993 215 230 Walker et al., 2009 M. Walker S. Johnsen S.O. Rasmussen T. Popp J.-P. Steffensen P. Gibbard Formal definition and dating of the GSSP (Global Stratotype Section and Point) for the base of the Holocene using the Greenland NGRIP ice core, and selected auxiliary records J. Quatern. Sci. 24 2009 3 17 Wright, 1983 H.E. Wright Jr. Late-Pleistocene glaciation and climate around the Junin Plain, Central Peruvian Highlands Geogr. Ann. A Phys. Geogr. 65 1983 35 43 Yokoyama et al., 2000 Y. Yokoyama K. Lambeck P. De Deckker P. Johnston L.K. Fifield Timing of the last glacial maximum from observed sea-level minima Nature 406 2000 713 716 Zech et al., 2007 R. Zech C. Kull P.W. Kubik H. Veit LGM and Late Glacial glacier advances in the Cordillera Real and Cochabamba (Bolivia) deduced from 10Be surface exposure dating Climate Past 3 2007 623 635
更多查看译文
关键词
younger dryas
AI 理解论文
溯源树
样例
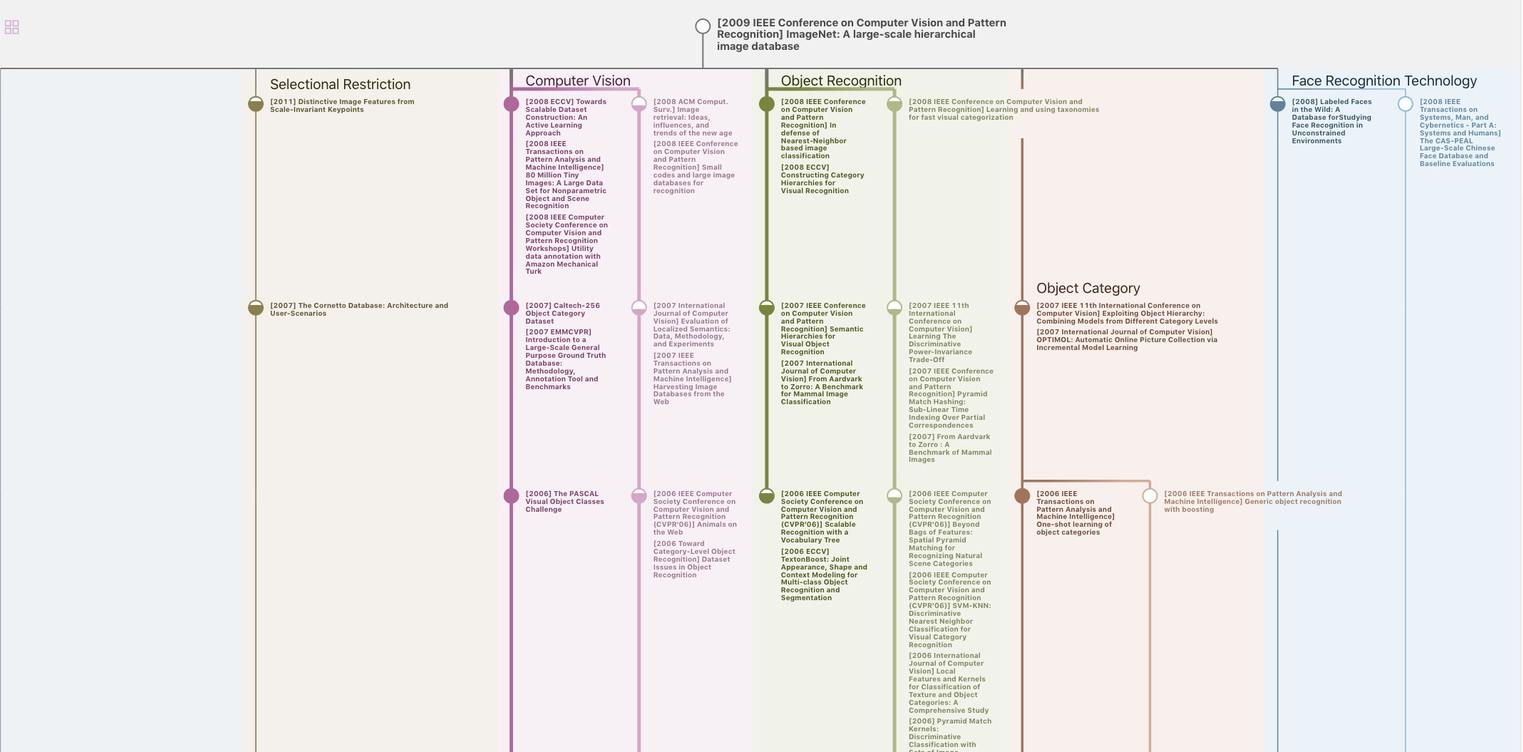
生成溯源树,研究论文发展脉络
Chat Paper
正在生成论文摘要